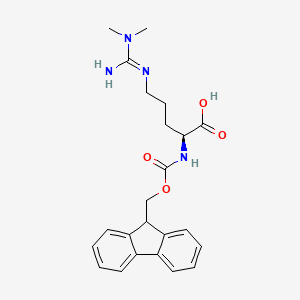
Fmoc-Arg(Me)2-OH
Overview
Description
The compound Fmoc-Arg(Me)₂-OH is a protected derivative of arginine used in solid-phase peptide synthesis (SPPS). It features a 9-fluorenylmethoxycarbonyl (Fmoc) group protecting the α-amino group and two methyl (Me) groups modifying the guanidine side chain of arginine. This protection strategy aims to prevent undesired side reactions, such as δ-lactam formation or premature deprotection during peptide assembly. Instead, the evidence focuses on structurally similar arginine derivatives, such as Fmoc-Arg(NO₂)-OH, Fmoc-Arg(Pbf)-OH, and Fmoc-Arg(Boc)₂-OH, which are widely used in SPPS. The following sections will compare these analogs in detail, drawing on empirical data from the literature.
Preparation Methods
Structural and Chemical Properties of Fmoc-Arg(Me)₂-OH
Fmoc-Arg(Me)₂-OH exists in two regioisomeric forms: symmetrical (Ng,Ng-dimethyl) and unsymmetrical (Ng,N’g-dimethyl). The symmetrical variant (CAS 1330286-66-1) includes a hydrochloride salt, while the unsymmetrical form (CAS 268564-10-9) lacks the counterion . Key properties include:
Table 1: Physical and Chemical Properties of Fmoc-Arg(Me)₂-OH Derivatives
Property | Fmoc-Arg(Me)₂-OH HCl sym. | Fmoc-Arg(Me)₂-OH unsym. |
---|---|---|
CAS Number | 1330286-66-1 | 268564-10-9 |
Molecular Formula | C₂₃H₂₈N₄O₄·HCl | C₂₃H₂₈N₄O₄ |
Molecular Weight | 461.0 | 424.5 |
Protection Groups | Fmoc, HCl | Fmoc |
The guanidino group’s dimethylation enhances peptide stability by reducing hydrogen-bonding capacity, thereby improving bioavailability .
Solution-Phase Synthesis Strategies
Stepwise Protection and Methylation
The solution-phase synthesis involves sequential protection of the α-amino group followed by selective dimethylation of the guanidino side chain:
-
α-Amino Protection :
L-arginine is treated with Fmoc-Cl (1.2 equiv) in dichloromethane (DCM) or dimethylformamide (DMF) under basic conditions (e.g., diisopropylethylamine, DIEA). The Fmoc group shields the α-amino group during subsequent reactions . -
Guanidino Methylation :
The guanidino group is dimethylated using methyl iodide (2.5–3.0 equiv) or dimethyl sulfate in the presence of a strong base (e.g., 1,8-diazabicyclo[5.4.0]undec-7-ene, DBU). DBU deprotonates the guanidino nitrogen, facilitating nucleophilic attack on the methylating agent .
Critical Parameters :
-
Temperature : Methylation proceeds optimally at 0–25°C to minimize side reactions.
-
Solvent : Polar aprotic solvents (e.g., DMF, N-methylpyrrolidone) enhance reagent solubility and reaction homogeneity .
Table 2: Methylation Efficiency with Different Reagents
Methylating Agent | Base | Yield (%) | Purity (%) |
---|---|---|---|
Methyl iodide | DBU | 85–92 | ≥95 |
Dimethyl sulfate | DBU | 78–88 | ≥90 |
Regioselective Control
Achieving symmetrical vs. unsymmetrical dimethylation requires careful selection of protecting groups:
-
Symmetrical Dimethylation : Temporary protection of the ω-nitrogen with a tert-butoxycarbonyl (Boc) group directs methylation to the Ng positions .
-
Unsymmetrical Dimethylation : Use of a selectively removable protecting group (e.g., 2,2,4,6,7-pentamethyldihydrobenzofuran-5-sulfonyl, Pmbf) enables sequential methylation .
Solid-Phase Synthesis Approaches
Resin-Based Functionalization
Solid-phase methods leverage 2-chlorotrityl chloride (2-CTC) resin to anchor the carboxylic acid group, enabling iterative modifications:
-
Resin Loading :
Fmoc-Arg-OH is loaded onto 2-CTC resin via its carboxylate group under mild acidic conditions (1% trifluoroacetic acid in DCM) . -
On-Resin Methylation :
After Fmoc deprotection (20% piperidine/DMF), the guanidino group is dimethylated using methyl iodide/DBU. The resin-bound intermediate is then cleaved with 1% TFA to yield Fmoc-Arg(Me)₂-OH .
Advantages :
-
Simplified purification (byproducts remain resin-bound).
Industrial-Scale Production
Process Optimization
Industrial synthesis prioritizes cost-effectiveness and scalability:
-
Continuous Flow Systems : Automated reactors enable precise control over reaction parameters (temperature, stoichiometry) .
-
Green Chemistry : Substitution of methyl iodide with dimethyl sulfate reduces toxicity and waste .
Table 3: Comparative Analysis of Industrial Methods
Parameter | Batch Reactor | Continuous Flow |
---|---|---|
Reaction Time | 8–12 h | 2–4 h |
Solvent Consumption | High | Low |
Yield | 80–85% | 88–92% |
Purification and Quality Control
Chromatographic Techniques
Crude Fmoc-Arg(Me)₂-OH is purified via reversed-phase high-performance liquid chromatography (RP-HPLC) using C18 columns and acetonitrile/water gradients .
Key Purity Metrics :
-
HPLC : ≥95% purity (detection at 220 nm).
-
Mass Spectrometry : Accurate mass confirmation (e.g., m/z 424.5 for unsymmetrical form) .
Challenges and Mitigation Strategies
Side Reactions
-
Over-Methylation : Excess methylating agents can lead to trimethylation. Mitigated by stoichiometric control and reaction monitoring via thin-layer chromatography (TLC) .
-
Racemization : Basic conditions during methylation may cause epimerization. Additives like OxymaPure suppress racemization .
Emerging Methodologies
Enzymatic Methylation
Recent advances explore methyltransferases for regioselective dimethylation under mild, aqueous conditions. For example, protein arginine methyltransferase 5 (PRMT5) catalyzes symmetrical dimethylation with >90% efficiency .
Chemical Reactions Analysis
Types of Reactions: Fmoc-Arg(Me)2-OH undergoes several types of chemical reactions, including:
Deprotection: The Fmoc group can be removed using a base such as piperidine, revealing the free amino group for further reactions.
Coupling Reactions: The free amino group can participate in peptide bond formation with other amino acids or peptides.
Methylation: The arginine side chain can be further methylated to introduce additional methyl groups.
Common Reagents and Conditions:
Deprotection: Piperidine in dimethylformamide (DMF) is commonly used to remove the Fmoc group.
Coupling Reactions: Reagents such as N,N’-diisopropylcarbodiimide (DIC) and OxymaPure are used for peptide bond formation.
Methylation: Methyl iodide (MeI) in the presence of a base like DIEA.
Major Products Formed:
Deprotection: The major product is the free amino group of arginine.
Coupling Reactions: The major products are peptides with this compound incorporated into the sequence.
Methylation: The major product is this compound with additional methyl groups.
Scientific Research Applications
Chemistry: Fmoc-Arg(Me)2-OH is widely used in the synthesis of peptides and proteins. It serves as a building block in the construction of complex peptide sequences, allowing researchers to study protein structure and function .
Biology: In biological research, this compound is used to create peptides that can be used as probes to study protein-protein interactions, enzyme activity, and cellular signaling pathways .
Medicine: this compound is used in the development of therapeutic peptides and peptide-based drugs. These peptides can be designed to target specific proteins or receptors, offering potential treatments for various diseases .
Industry: In the pharmaceutical industry, this compound is used in the large-scale production of peptide drugs. Its use in solid-phase peptide synthesis allows for the efficient and cost-effective production of high-purity peptides .
Mechanism of Action
The mechanism of action of Fmoc-Arg(Me)2-OH involves its role as a protecting group in peptide synthesis. The Fmoc group protects the amino group of arginine during the synthesis process, preventing unwanted side reactions. The Fmoc group can be selectively removed under mildly basic conditions, revealing the free amino group for further reactions. This allows for the sequential addition of amino acids to form peptides .
Comparison with Similar Compounds
Fmoc-Arg(NO₂)-OH
- Key Features: The nitro (NO₂) group is an electron-withdrawing protecting group that reduces the guanidine’s basicity, minimizing side reactions like δ-lactam formation .
- Performance in SPPS: Low δ-lactam formation: Only 3% δ-lactam observed after 30 minutes of activation, compared to 12% for Pbf and 60% for (Boc)₂ analogs . High coupling efficiency: >99% coupling achieved within 30 minutes due to rapid activation and minimal side reactions .
Fmoc-Arg(Pbf)-OH
Key Features :
- Performance in SPPS: Moderate δ-lactam formation: 12% δ-lactam after 30 minutes, increasing to near-complete conversion after 120 minutes . High coupling efficiency under optimized conditions: Up to 93% coupling achieved using HBTU/HOBt/DIEA in DMA/DCM (1:1) . Kinetic challenges: Slower activation kinetics compared to NO₂ analogs but compatible with microwave-assisted synthesis (84.7% coupling in 3 minutes) .
Fmoc-Arg(Boc)₂-OH
Key Features :
Performance in SPPS :
- Rapid δ-lactam formation : 60% δ-lactam observed within 30 minutes, leading to poor coupling efficiency (28%) .
- Long-term stability : Degrades slowly in DMF and NBP (88.8% to 51.2% remaining after 30 days) .
- Niche applications : Preferred in PNA (peptide nucleic acid) synthesis due to compatibility with automated synthesizers .
Data Tables
Table 1: Comparative δ-Lactam Formation and Coupling Efficiency
Compound | δ-Lactam Formation (30 min) | Coupling Efficiency (30 min) | Preferred Solvent | Deprotection Method |
---|---|---|---|---|
Fmoc-Arg(NO₂)-OH | 3% | >99% | DMF/NBP | Catalytic hydrogenation |
Fmoc-Arg(Pbf)-OH | 12% | 93%* | DMA/DCM (1:1) | TFA (room temperature) |
Fmoc-Arg(Boc)₂-OH | 60% | 28% | DMF/NBP | TFA (prolonged) |
*Under optimized conditions with HBTU/HOBt/DIEA .
Table 2: Stability in Solvents (Remaining Fmoc-Arg(X)-OH after 30 Days)
Compound | DMF (%) | NBP (%) |
---|---|---|
Fmoc-Arg(Boc)₂-OH | 51.2 | 37.7 |
Fmoc-Arg(NO₂)-OH* | >95 | >95 |
Fmoc-Arg(Pbf)-OH* | >95 | >95 |
*Data inferred from stability trends in .
Critical Analysis of Research Findings
- NO₂ vs. Pbf: NO₂ analogs excel in minimizing δ-lactam formation and achieving rapid coupling, but their deprotection methods (hydrogenation) are less practical for large-scale or industrial synthesis . Pbf analogs balance coupling efficiency and mild deprotection, making them ideal for complex peptides like leuprolide acetate (90.2% yield) .
Boc₂ Limitations :
Emerging Trends :
- Microwave-assisted synthesis and pseudoproline motifs improve coupling efficiency for sterically hindered residues like Arg(Pbf) .
Biological Activity
Introduction
Fmoc-Arg(Me)2-OH, also known as Fmoc-N,N-dimethylarginine, is a derivative of arginine that plays a significant role in peptide synthesis and biological applications. This compound is particularly notable for its involvement in solid-phase peptide synthesis (SPPS) and its potential therapeutic applications due to its unique structural properties. This article explores the biological activity of this compound, including its synthesis, applications, and relevant case studies.
This compound is synthesized using the Fmoc (9-fluorenylmethoxycarbonyl) protection strategy, which is widely employed in SPPS. The Fmoc group protects the amino functionality of the arginine residue, allowing for selective coupling reactions without affecting other functional groups. The synthesis typically involves the following steps:
- Protection of the Amino Group : The amino group of arginine is protected using the Fmoc group.
- Methylation : The guanidino group of arginine undergoes methylation to introduce two methyl groups.
- Cleavage and Purification : After the desired peptide sequence is assembled, the Fmoc group is removed to yield the final product.
Table 1: Synthesis Overview
Step | Description | Reagents |
---|---|---|
1 | Fmoc Protection | Fmoc-Cl |
2 | Methylation | Methyl iodide |
3 | Cleavage | Piperidine |
This compound exhibits biological activity primarily through its interaction with various receptors and enzymes. It has been shown to influence nitric oxide (NO) production due to its structural similarity to arginine, a known substrate for nitric oxide synthase (NOS). The dimethylation enhances its stability and may influence its binding affinity to certain targets.
Applications in Research and Medicine
- Peptide Synthesis : this compound is extensively used in SPPS for creating peptides with enhanced pharmacological properties.
- Drug Development : Its ability to modulate biological pathways makes it a candidate for developing drugs targeting cardiovascular diseases and other conditions influenced by NO levels.
- Bioorthogonal Chemistry : The compound can facilitate bioorthogonal reactions, allowing for selective labeling and imaging of peptides in biological systems.
Case Study 1: Tumor Imaging
A study demonstrated that peptides synthesized using this compound exhibited high affinity for tumor cells expressing specific receptors. These peptides were successfully labeled with fluorescent dyes using bioorthogonal chemistry, allowing for effective imaging of tumors in vivo. Results indicated significant tumor uptake and retention, suggesting potential applications in targeted cancer therapies .
Case Study 2: Cardiovascular Research
Research highlighted the role of this compound in modulating vascular responses through NO production. In vitro studies showed that peptides containing this compound enhanced vasodilation in endothelial cells, indicating its potential therapeutic use in treating hypertension .
Q & A
Basic Research Questions
Q. What is the role of Fmoc-Arg(Me)₂-OH in solid-phase peptide synthesis (SPPS)?
Fmoc-Arg(Me)₂-OH is a protected amino acid derivative used to introduce asymmetric dimethylarginine residues into peptides during Fmoc-based SPPS. The Fmoc group protects the α-amino group, while the dimethyl (Me)₂ modification on the arginine side chain ensures site-specific methylation, critical for studying post-translational modifications (PTMs) in proteins. Proper handling involves pre-activation with coupling reagents (e.g., DIC/OxymaPure) and solvent optimization to minimize side reactions .
Q. How does asymmetric dimethylarginine (ADMA) differ from symmetric dimethylarginine (SDMA) in peptide design?
ADMA (asymmetric) and SDMA (symmetric) differ in the placement of methyl groups on the arginine guanidine side chain. ADMA has two methyl groups on one nitrogen atom, while SDMA distributes them across two nitrogens. This structural distinction affects biological interactions, such as nitric oxide synthase inhibition (ADMA-specific) or protein-protein binding. Fmoc-Arg(Me)₂-OH enables selective ADMA incorporation, whereas SDMA requires distinct protecting groups .
Advanced Research Questions
Q. How can δ-lactam formation during Fmoc-Arg(Me)₂-OH coupling be mitigated?
δ-lactam formation, a side reaction where the activated arginine derivative cyclizes, reduces coupling efficiency. Key strategies include:
- Solvent selection : DMF suppresses δ-lactam formation compared to NBP (nitrobenzyl alcohol/phenol), as shown in kinetic studies (Figure 2, ).
- Temperature control : Lower temperatures (e.g., 45°C) slow cyclization while maintaining coupling efficiency (>92% incorporation) .
- Reagent stoichiometry : Limiting DIC (e.g., 0.9 equiv.) promotes active ester formation over cyclization (Scheme 3, ).
Q. Which analytical methods are effective for monitoring Fmoc-Arg(Me)₂-OH coupling efficiency?
- HPLC : Track residual Fmoc-Arg(Me)₂-OH and δ-lactam byproducts in reaction supernatants over time (0–48 hrs). Retention times and peak areas provide quantitative coupling metrics (Figure 3, ).
- Mass spectrometry : Post-cleavage analysis of resin-bound peptides confirms methylation integrity and coupling success (≥98% purity in DMF vs. <50% in NBP) .
Q. How can coupling conditions for Fmoc-Arg(Me)₂-OH be optimized for challenging sequences?
- Pre-activation : Pre-form the active ester (Fmoc-Arg(Me)₂-Oxyma) in DMF at 45°C for 10 min before resin addition .
- Double coupling : Apply 1.5 equiv. of pre-activated reagent twice, with intermediate washes, to overcome steric hindrance in branched peptides .
- Additive screening : OxymaPure enhances coupling kinetics and reduces racemization compared to HOBt/DMAP .
Q. Data-Driven Insights
Properties
IUPAC Name |
(2S)-5-[[amino(dimethylamino)methylidene]amino]-2-(9H-fluoren-9-ylmethoxycarbonylamino)pentanoic acid | |
---|---|---|
Source | PubChem | |
URL | https://pubchem.ncbi.nlm.nih.gov | |
Description | Data deposited in or computed by PubChem | |
InChI |
InChI=1S/C23H28N4O4/c1-27(2)22(24)25-13-7-12-20(21(28)29)26-23(30)31-14-19-17-10-5-3-8-15(17)16-9-4-6-11-18(16)19/h3-6,8-11,19-20H,7,12-14H2,1-2H3,(H2,24,25)(H,26,30)(H,28,29)/t20-/m0/s1 | |
Source | PubChem | |
URL | https://pubchem.ncbi.nlm.nih.gov | |
Description | Data deposited in or computed by PubChem | |
InChI Key |
QXAXLSMKNHJDNM-FQEVSTJZSA-N | |
Source | PubChem | |
URL | https://pubchem.ncbi.nlm.nih.gov | |
Description | Data deposited in or computed by PubChem | |
Canonical SMILES |
CN(C)C(=NCCCC(C(=O)O)NC(=O)OCC1C2=CC=CC=C2C3=CC=CC=C13)N | |
Source | PubChem | |
URL | https://pubchem.ncbi.nlm.nih.gov | |
Description | Data deposited in or computed by PubChem | |
Isomeric SMILES |
CN(C)C(=NCCC[C@@H](C(=O)O)NC(=O)OCC1C2=CC=CC=C2C3=CC=CC=C13)N | |
Source | PubChem | |
URL | https://pubchem.ncbi.nlm.nih.gov | |
Description | Data deposited in or computed by PubChem | |
Molecular Formula |
C23H28N4O4 | |
Source | PubChem | |
URL | https://pubchem.ncbi.nlm.nih.gov | |
Description | Data deposited in or computed by PubChem | |
DSSTOX Substance ID |
DTXSID40673979 | |
Record name | (E)-N~5~-[Amino(dimethylamino)methylidene]-N~2~-{[(9H-fluoren-9-yl)methoxy]carbonyl}-L-ornithine | |
Source | EPA DSSTox | |
URL | https://comptox.epa.gov/dashboard/DTXSID40673979 | |
Description | DSSTox provides a high quality public chemistry resource for supporting improved predictive toxicology. | |
Molecular Weight |
424.5 g/mol | |
Source | PubChem | |
URL | https://pubchem.ncbi.nlm.nih.gov | |
Description | Data deposited in or computed by PubChem | |
CAS No. |
268564-10-9 | |
Record name | (E)-N~5~-[Amino(dimethylamino)methylidene]-N~2~-{[(9H-fluoren-9-yl)methoxy]carbonyl}-L-ornithine | |
Source | EPA DSSTox | |
URL | https://comptox.epa.gov/dashboard/DTXSID40673979 | |
Description | DSSTox provides a high quality public chemistry resource for supporting improved predictive toxicology. | |
Retrosynthesis Analysis
AI-Powered Synthesis Planning: Our tool employs the Template_relevance Pistachio, Template_relevance Bkms_metabolic, Template_relevance Pistachio_ringbreaker, Template_relevance Reaxys, Template_relevance Reaxys_biocatalysis model, leveraging a vast database of chemical reactions to predict feasible synthetic routes.
One-Step Synthesis Focus: Specifically designed for one-step synthesis, it provides concise and direct routes for your target compounds, streamlining the synthesis process.
Accurate Predictions: Utilizing the extensive PISTACHIO, BKMS_METABOLIC, PISTACHIO_RINGBREAKER, REAXYS, REAXYS_BIOCATALYSIS database, our tool offers high-accuracy predictions, reflecting the latest in chemical research and data.
Strategy Settings
Precursor scoring | Relevance Heuristic |
---|---|
Min. plausibility | 0.01 |
Model | Template_relevance |
Template Set | Pistachio/Bkms_metabolic/Pistachio_ringbreaker/Reaxys/Reaxys_biocatalysis |
Top-N result to add to graph | 6 |
Feasible Synthetic Routes
Disclaimer and Information on In-Vitro Research Products
Please be aware that all articles and product information presented on BenchChem are intended solely for informational purposes. The products available for purchase on BenchChem are specifically designed for in-vitro studies, which are conducted outside of living organisms. In-vitro studies, derived from the Latin term "in glass," involve experiments performed in controlled laboratory settings using cells or tissues. It is important to note that these products are not categorized as medicines or drugs, and they have not received approval from the FDA for the prevention, treatment, or cure of any medical condition, ailment, or disease. We must emphasize that any form of bodily introduction of these products into humans or animals is strictly prohibited by law. It is essential to adhere to these guidelines to ensure compliance with legal and ethical standards in research and experimentation.