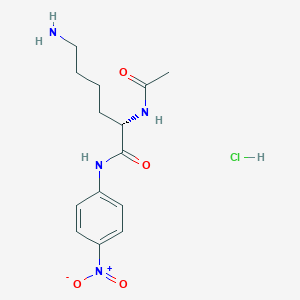
N-alpha-Acetyl-L-lysine p-nitroanilide hydrochloride
Overview
Description
N-alpha-Acetyl-L-lysine p-nitroanilide hydrochloride (CAS 201677-92-1, free base CAS 50931-35-6) is a chromogenic peptide derivative widely used in enzymology and proteomics research. Its molecular formula is C₁₃H₁₇ClN₄O₄, with a molecular weight of 344.80 g/mol (hydrochloride form) . Structurally, it consists of N-acetylated L-lysine linked to p-nitroaniline via an amide bond. The compound serves as a substrate for enzymes that cleave lysine-containing peptides, releasing 4-nitroaniline (detectable spectrophotometrically at 410 nm) . Its applications include:
Preparation Methods
Acetylation of L-Lysine
The synthesis begins with the acetylation of the α-amino group of L-lysine to produce N-alpha-acetyl-L-lysine. This step ensures selective protection of the amino group, preventing unwanted side reactions during subsequent coupling.
Reagents and Conditions
-
Reagents : Acetic anhydride, pyridine (base catalyst), and L-lysine.
-
Solvent : Anhydrous methanol or dimethylformamide (DMF).
-
Temperature : 0–4°C (initial reaction), followed by room temperature incubation.
-
Reaction Time : 6–12 hours.
The reaction proceeds via nucleophilic acyl substitution, where the amino group attacks the electrophilic carbonyl carbon of acetic anhydride. Pyridine neutralizes the generated acetic acid, shifting the equilibrium toward product formation .
Workup and Isolation
Post-reaction, the mixture is concentrated under reduced pressure. The residue is dissolved in water, acidified to pH 2–3 with hydrochloric acid, and extracted with ethyl acetate to remove unreacted reagents. The aqueous layer is lyophilized to yield N-alpha-acetyl-L-lysine as a white solid.
Coupling with p-Nitroaniline
The acetylated lysine is coupled with p-nitroaniline to form the amide bond. This step requires activation of the carboxyl group to enhance electrophilicity.
Activation Strategies
-
Acyl Chloride Formation : Thionyl chloride (SOCl₂) converts the carboxyl group into an acyl chloride.
-
Conditions : Reflux in anhydrous dichloromethane (DCM) for 2–4 hours.
-
-
Mixed Carbonate Approach : Alternatively, carbodiimide coupling agents like EDC (1-ethyl-3-(3-dimethylaminopropyl)carbodiimide) with HOBt (hydroxybenzotriazole) are used in DMF .
Coupling Reaction
-
Reagents : Activated N-alpha-acetyl-L-lysine, p-nitroaniline, triethylamine (TEA).
-
Solvent : Anhydrous DCM or DMF.
-
Temperature : 0°C (initial), then room temperature.
-
Reaction Time : 12–24 hours.
The reaction is monitored via thin-layer chromatography (TLC). Upon completion, the solvent is evaporated, and the crude product is precipitated using ice-cold diethyl ether.
Formation of the Hydrochloride Salt
The free base of the coupled product is converted to its hydrochloride salt to enhance solubility and stability.
Procedure
-
The product is dissolved in minimal anhydrous ethanol.
-
Hydrogen chloride gas is bubbled through the solution until precipitation occurs.
-
Alternatively, concentrated hydrochloric acid is added dropwise under stirring.
The mixture is cooled to 4°C to promote crystallization. The precipitate is filtered, washed with cold ethanol, and dried under vacuum.
Purification and Characterization
Final purification ensures high purity, critical for biochemical applications.
Methods
-
Recrystallization : The hydrochloride salt is recrystallized from a mixture of ethanol and water (3:1 v/v).
-
Column Chromatography : Silica gel chromatography with a gradient of methanol in chloroform (0–5%) removes residual impurities.
Analytical Validation
-
HPLC : Reverse-phase HPLC (C18 column, 0.1% TFA in acetonitrile/water) confirms >98% purity.
-
NMR : ¹H NMR (D₂O, 400 MHz) shows characteristic peaks: δ 8.15 (d, 2H, aromatic), δ 4.25 (m, 1H, α-CH), δ 3.10 (t, 2H, ε-CH₂) .
-
Mass Spectrometry : ESI-MS m/z 344.79 [M+H]⁺, consistent with the molecular formula C₁₄H₂₁ClN₄O₄ .
Optimization and Challenges
Yield Considerations
-
Acetylation Step : 85–90% yield (due to competing ε-amino acetylation).
-
Coupling Step : 60–75% yield (steric hindrance from the acetyl group).
-
Salt Formation : >95% yield.
Mitigating Side Reactions
-
Selective Protection : Using Boc (tert-butoxycarbonyl) for the ε-amino group prior to acetylation reduces byproducts.
-
Low-Temperature Coupling : Minimizes racemization and hydrolysis.
Comparative Analysis of Synthetic Routes
The table below summarizes key parameters for different preparation strategies:
Step | Reagents/Conditions | Yield (%) | Purity (%) |
---|---|---|---|
Acetylation | Acetic anhydride, pyridine | 85–90 | 90 |
Acyl Chloride Coupling | SOCl₂, TEA | 60–70 | 85 |
Carbodiimide Coupling | EDC/HOBt, DMF | 65–75 | 92 |
Salt Formation | HCl gas, ethanol | 95 | 98 |
Industrial-Scale Production
Industrial synthesis prioritizes cost-effectiveness and scalability:
Chemical Reactions Analysis
Types of Reactions
N-alpha-Acetyl-L-lysine p-nitroanilide hydrochloride primarily undergoes hydrolysis reactions when exposed to lysine-specific proteases. The hydrolysis breaks the bond between the lysine and p-nitroaniline moieties, releasing p-nitroaniline, which can be detected colorimetrically .
Common Reagents and Conditions
Common reagents used in the reactions involving this compound include proteolytic enzymes such as trypsin and lysyl oxidase. The reactions are typically carried out under mild conditions, such as physiological pH and temperature .
Major Products Formed
The major product formed from the hydrolysis of this compound is p-nitroaniline, which serves as a chromophore for colorimetric detection .
Scientific Research Applications
Key Applications
-
Biochemical Assays
- N-alpha-Acetyl-L-lysine p-nitroanilide hydrochloride serves as a substrate for various proteases, particularly serine proteases like trypsin and urokinase. Its hydrolysis releases p-nitroaniline, which can be spectrophotometrically monitored, making it a valuable tool for studying enzyme kinetics and inhibition .
- Drug Development
- Protein Labeling
- Diagnostic Applications
- Research on Metabolic Pathways
Enzymatic Activity Studies
In a study examining the kinetics of trypsin-catalyzed hydrolysis of this compound, researchers observed that the reaction rate varied significantly with pH levels, indicating that environmental conditions critically influence enzymatic activity. This highlights the importance of this compound in understanding enzyme behavior under different physiological conditions .
Inhibition Studies
Another study demonstrated that this compound could serve as a competitive inhibitor for plasmin when used alongside other substrates. This finding is significant for developing antifibrinolytic agents that could be used therapeutically to manage bleeding disorders .
Data Summary Table
Mechanism of Action
N-alpha-Acetyl-L-lysine p-nitroanilide hydrochloride exerts its effects by serving as a substrate for lysine-specific proteases. Upon enzymatic hydrolysis, the bond between the lysine and p-nitroaniline moieties is cleaved, releasing p-nitroaniline. This release can be detected colorimetrically, allowing for the quantification of protease activity . The molecular targets involved in this process are the active sites of lysine-specific proteases .
Comparison with Similar Compounds
Comparison with Similar p-Nitroanilide Derivatives
Chromogenic p-nitroanilide substrates share a common mechanism: enzymatic cleavage releases 4-nitroaniline, enabling quantification. Below is a detailed comparison:
Structural and Functional Differences
Kinetic Parameters and Enzyme Specificity
- N-alpha-Acetyl-L-lysine p-nitroanilide: Limited kinetic data available, but its lysine-acetylated structure suggests specificity for enzymes recognizing acetylated substrates (e.g., histone deacetylases or acetyl-lysine-binding proteases) .
- BAPNA : Exhibits a Km of 0.61 mM and kcat/Km of 0.23 s⁻¹·mM⁻¹ for ISP1, indicating moderate catalytic efficiency .
- FVR-pNA : Higher catalytic efficiency (kcat/Km = 0.56 s⁻¹·mM⁻¹ ) due to optimized tripeptide sequence .
- L-Ala-pNA: No detectable activity with ISP1 or trypsin, confirming the necessity of arginine/lysine for cleavage .
Key Research Findings
Substrate Specificity: Enzymes like ISP1 exhibit strict preference for arginine/lysine in the P1 position.
Inhibitor Screening : BAPNA and similar substrates are used to test serine protease inhibitors (e.g., PMSF, TLCK) .
Species-Specific Variations : Pacific cod trypsin hydrolyzes BAPA (Km ~0.1 mM) more efficiently than mammalian trypsins, highlighting evolutionary adaptations .
Biological Activity
N-alpha-Acetyl-L-lysine p-nitroanilide hydrochloride (Ac-Lys-pNA) is a synthetic compound widely utilized in biochemical research due to its specific interactions with proteolytic enzymes. This article delves into its biological activity, mechanisms of action, and applications in various fields.
- Molecular Formula: C14H21ClN4O4
- Molecular Weight: 344.79 g/mol
- CAS Number: 201677-92-1
Ac-Lys-pNA is characterized by its acetylated lysine structure, which enhances its solubility and stability compared to unmodified lysine derivatives.
Ac-Lys-pNA primarily functions as a substrate for specific proteolytic enzymes, particularly lysine aminopeptidases and serine proteases. Upon enzymatic hydrolysis, it releases p-nitroaniline, a chromogenic product that can be quantified spectrophotometrically.
Enzymatic Hydrolysis
The hydrolysis reaction can be summarized as follows:
This reaction is crucial for understanding enzyme kinetics and specificity.
Cellular Effects
Ac-Lys-pNA influences several cellular processes by participating in proteolytic pathways. The release of p-nitroaniline serves as an indicator of enzyme activity, impacting:
- Cell Signaling Pathways: The compound's hydrolysis can modulate signaling pathways that are critical for cellular responses.
- Gene Expression: By affecting proteolytic activity, Ac-Lys-pNA may indirectly influence the expression of genes involved in metabolism and cellular growth.
Dosage Effects in Animal Models
Research indicates that the biological effects of Ac-Lys-pNA vary with dosage. Higher concentrations have been shown to enhance enzyme activity significantly, which can lead to toxicity if not properly regulated. Studies suggest that careful dosage is essential for both experimental accuracy and safety in animal models .
Biochemical Assays
Ac-Lys-pNA is extensively used in various scientific applications:
- Enzyme Kinetics: It serves as a model substrate for studying the kinetics of lysine-specific proteases.
- Diagnostic Tools: The compound is employed in assays to detect protease activity related to diseases such as cancer and neurodegenerative disorders.
- Industrial Applications: It is utilized in enzyme-based processes within biotechnology and pharmaceutical industries.
Case Studies
-
Kinetic Studies on Trypsin Activity:
A study demonstrated the pH dependence of trypsin-catalyzed hydrolysis of Ac-Lys-pNA, revealing optimal enzymatic activity at alkaline pH levels. This information is crucial for optimizing conditions in biochemical assays . -
Protease Activity in Disease Models:
In models of periodontal disease, Ac-Lys-pNA has been used to assess the activity of cysteine proteases produced by pathogenic bacteria, providing insights into potential therapeutic interventions .
Q & A
Basic Questions
Q. What are the recommended storage conditions and handling protocols for N-alpha-Acetyl-L-lysine p-nitroanilide hydrochloride to ensure stability in laboratory settings?
- Methodological Answer: Store the compound in a desiccator at 2–8°C, protected from light and moisture, to prevent hydrolysis of the p-nitroanilide group. Aliquot stock solutions to avoid repeated freeze-thaw cycles. Prior to use, confirm solubility in assay buffers (e.g., Tris-HCl or phosphate buffers, pH 7.0–8.0) and filter-sterilize if required. Refer to safety data sheets for handling guidelines, including personal protective equipment (PPE) such as nitrile gloves and lab coats .
Q. How is this compound typically used as a substrate in protease activity assays?
- Methodological Answer: The compound serves as a chromogenic substrate, releasing p-nitroaniline upon enzymatic cleavage, detectable at 405 nm. Standard protocols involve incubating the substrate (0.1–2.0 mM) with the target enzyme (e.g., trypsin-like proteases) in a buffered system (e.g., Tris-HCl, pH 8.2) at 37°C. Kinetic measurements are performed in microplate readers using initial rate calculations. Include negative controls (e.g., enzyme-free or inhibitor-treated samples) to account for non-specific hydrolysis .
Q. What analytical techniques are employed to assess the purity and structural integrity of this compound prior to experimental use?
- Methodological Answer: Reverse-phase HPLC with a C18 column (mobile phase: acetonitrile/water gradient) is used to quantify purity (>98%) and detect related substances. Thin-layer chromatography (TLC) on silica gel plates (eluent: chloroform-methanol mixtures) provides rapid purity checks. Confirm structural integrity via NMR (1H/13C) and mass spectrometry (ESI-MS) .
Advanced Research Questions
Q. What methodological considerations are critical when determining kinetic parameters (e.g., , ) of enzymes using this substrate?
- Methodological Answer: Optimize substrate concentrations to avoid inhibition at high levels (e.g., 0.05–1.0 mM). Use non-linear regression (e.g., Michaelis-Menten or Hill plots) for parameter estimation. Account for buffer effects (e.g., ionic strength, pH) and temperature (25–37°C). For competitive inhibition studies, include varying inhibitor concentrations and calculate using Dixon plots .
Q. How can researchers resolve contradictions in enzymatic activity data obtained from different experimental setups using this substrate?
- Methodological Answer: Discrepancies often arise from variations in buffer composition (e.g., Tris vs. phosphate), substrate preparation (e.g., stock solution stability), or detection sensitivity (e.g., plate reader calibration). Standardize protocols using reference enzymes (e.g., trypsin) and validate with inter-laboratory comparisons. Address interference from endogenous proteases via pre-incubation with class-specific inhibitors (e.g., PMSF for serine proteases) .
Q. What strategies are effective in minimizing interference from endogenous substrates or enzymes when utilizing this compound in complex biological matrices?
- Methodological Answer: Pre-treat samples (e.g., plasma, tissue homogenates) with affinity chromatography or precipitation (e.g., ammonium sulfate) to remove interfering proteins. Use synthetic protease inhibitors (e.g., aprotinin, leupeptin) at non-competitive concentrations. Validate specificity via knockout models or RNA interference targeting the enzyme of interest .
Q. How can substrate inhibition effects be modeled and mitigated in kinetic studies with this compound?
- Methodological Answer: Substrate inhibition at high concentrations (>2.0 mM) can be modeled using the modified Michaelis-Menten equation:
Experimentally, reduce substrate concentrations or switch to a non-inhibitory analog. Validate models with statistical tools (e.g., Akaike information criterion) to select the best-fit kinetic mechanism .
Properties
IUPAC Name |
(2S)-2-acetamido-6-amino-N-(4-nitrophenyl)hexanamide;hydrochloride | |
---|---|---|
Source | PubChem | |
URL | https://pubchem.ncbi.nlm.nih.gov | |
Description | Data deposited in or computed by PubChem | |
InChI |
InChI=1S/C14H20N4O4.ClH/c1-10(19)16-13(4-2-3-9-15)14(20)17-11-5-7-12(8-6-11)18(21)22;/h5-8,13H,2-4,9,15H2,1H3,(H,16,19)(H,17,20);1H/t13-;/m0./s1 | |
Source | PubChem | |
URL | https://pubchem.ncbi.nlm.nih.gov | |
Description | Data deposited in or computed by PubChem | |
InChI Key |
XMMQBHVCNLUPQM-ZOWNYOTGSA-N | |
Source | PubChem | |
URL | https://pubchem.ncbi.nlm.nih.gov | |
Description | Data deposited in or computed by PubChem | |
Canonical SMILES |
CC(=O)NC(CCCCN)C(=O)NC1=CC=C(C=C1)[N+](=O)[O-].Cl | |
Source | PubChem | |
URL | https://pubchem.ncbi.nlm.nih.gov | |
Description | Data deposited in or computed by PubChem | |
Isomeric SMILES |
CC(=O)N[C@@H](CCCCN)C(=O)NC1=CC=C(C=C1)[N+](=O)[O-].Cl | |
Source | PubChem | |
URL | https://pubchem.ncbi.nlm.nih.gov | |
Description | Data deposited in or computed by PubChem | |
Molecular Formula |
C14H21ClN4O4 | |
Source | PubChem | |
URL | https://pubchem.ncbi.nlm.nih.gov | |
Description | Data deposited in or computed by PubChem | |
Molecular Weight |
344.79 g/mol | |
Source | PubChem | |
URL | https://pubchem.ncbi.nlm.nih.gov | |
Description | Data deposited in or computed by PubChem | |
Disclaimer and Information on In-Vitro Research Products
Please be aware that all articles and product information presented on BenchChem are intended solely for informational purposes. The products available for purchase on BenchChem are specifically designed for in-vitro studies, which are conducted outside of living organisms. In-vitro studies, derived from the Latin term "in glass," involve experiments performed in controlled laboratory settings using cells or tissues. It is important to note that these products are not categorized as medicines or drugs, and they have not received approval from the FDA for the prevention, treatment, or cure of any medical condition, ailment, or disease. We must emphasize that any form of bodily introduction of these products into humans or animals is strictly prohibited by law. It is essential to adhere to these guidelines to ensure compliance with legal and ethical standards in research and experimentation.