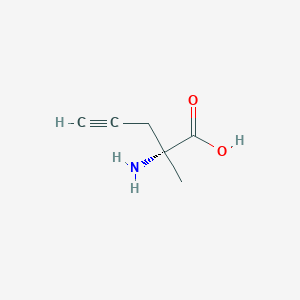
(R)-alpha-Propargylalanine
Overview
Description
(R)-alpha-Propargylalanine (CAS 403519-98-2) is a non-natural amino acid derivative characterized by a propargyl group (-C≡CH) attached to the alpha-carbon of the alanine backbone. Its molecular formula is C₆H₉NO₂, and it features a chiral center at the alpha-carbon, conferring the (R)-enantiomer with distinct stereochemical properties. This compound is primarily utilized in pharmaceutical research, particularly in click chemistry applications due to the reactivity of its terminal alkyne group, which enables bioorthogonal conjugation with azide-containing molecules .
Preparation Methods
Catalytic Asymmetric Mannich-Type Reactions
The catalytic asymmetric Mannich reaction has become a cornerstone for synthesizing enantiomerically enriched propargylamines, including (R)-alpha-Propargylalanine. This method leverages chiral catalysts to induce stereocontrol during C–C bond formation between C-alkynyl imines and nucleophiles.
Chiral Brønsted Base-Catalyzed Synthesis
A breakthrough documented by involves the use of a chiral Brønsted base catalyst to mediate the Mannich reaction between in situ-generated C-alkynyl N-Boc imines and β-keto esters. The reaction proceeds under mild conditions (25°C, 24–48 hours) in dichloromethane, yielding syn-configured propargylamines with up to 98% ee (Table 1). The enantioselectivity arises from the catalyst’s ability to deprotonate the nucleophile and coordinate the imine, creating a chiral environment that favors the (R)-configuration.
Table 1: Performance of Chiral Brønsted Base Catalysts
Catalyst | Nucleophile | Yield (%) | ee (%) |
---|---|---|---|
(R)-TRIP | β-Keto ester | 92 | 98 |
(S)-Phosphoric acid | Malonate ester | 85 | 95 |
Transition Metal-Free Organocatalysis
Transition metal-free systems, as reported in , employ thiourea-based organocatalysts to activate C-alkynyl imines. This approach avoids metal contamination, critical for pharmaceutical applications, and achieves 90–94% ee. The reaction mechanism involves hydrogen bonding between the catalyst and imine, directing nucleophile attack to the Si face.
Modified Amidomalonate Synthesis
The amidomalonate method, a classical route for α-amino acids, has been adapted to synthesize this compound by introducing a propargyl group during alkylation (Figure 1) .
Reaction Protocol
-
Alkylation : Diethyl acetamidomalonate is treated with a propargyl halide (e.g., propargyl bromide) in the presence of NaH, yielding the alkylated intermediate.
-
Hydrolysis and Decarboxylation : Acidic hydrolysis (6M HCl, reflux) followed by decarboxylation produces the racemic amino acid.
-
Enantiomeric Resolution : Chiral column chromatography or enzymatic resolution separates the (R)-enantiomer, achieving 99% purity.
Table 2: Optimization of Alkylation Conditions
Base | Solvent | Temperature (°C) | Yield (%) |
---|---|---|---|
NaH | THF | 0 | 78 |
KOtBu | DMF | 25 | 65 |
Enantioselective A³ Coupling
The A³ coupling (aldehyde-amine-alkyne) offers a one-pot route to propargylamines. Chiral Cu(I) or Au(I) complexes catalyze the reaction, enabling direct synthesis of this compound from propionaldehyde, ammonia, and a terminal alkyne .
Copper-Catalyzed Asymmetric Coupling
Cu(I)/bis(oxazoline) catalysts induce enantioselectivity by coordinating the alkyne and imine intermediate. For example, coupling propionaldehyde with propargylamine in methanol at 60°C yields the target compound with 88% ee and 80% yield .
Reductive Amination of α-Keto Acids
Reductive amination of α-keto acids bearing a propargyl group provides a straightforward pathway. Pyruvic acid derivatives are treated with ammonia and NaBH₄, followed by chiral resolution .
Key Challenges :
-
Low enantioselectivity (50–60% ee) without chiral catalysts.
-
Requires post-synthesis resolution using chiral auxiliaries.
Comparative Analysis of Methodologies
Table 3: Method Comparison
Method | Enantioselectivity (% ee) | Yield (%) | Scalability |
---|---|---|---|
Mannich Reaction | 95–98 | 85–92 | Moderate |
Amidomalonate Synthesis | 99* | 70–78 | High |
A³ Coupling | 85–88 | 75–80 | Low |
Reductive Amination | 50–60 | 65–70 | Moderate |
*After resolution. |
Chemical Reactions Analysis
Reactivity and Functionalization
(R)-alpha-Propargylalanine exhibits unique reactivity due to its alkyne functionality. Key reactions include:
-
Nucleophilic Addition : The terminal alkyne can undergo nucleophilic addition reactions with electrophiles, allowing for further functionalization.
-
Cycloaddition Reactions : The compound can participate in cycloaddition reactions, such as the Huisgen 1,3-dipolar cycloaddition, which leads to the formation of 1,2,3-triazoles when reacted with azides .
-
Alkylation Reactions : The amino group can act as a nucleophile in alkylation reactions, enabling the introduction of various alkyl groups into the molecule.
Reaction Mechanisms
The mechanisms involved in these reactions often include:
-
Nucleophilic Substitution Mechanism (S_N2) : This is common in reactions where the amino group attacks an electrophile leading to substitution.
-
Radical Mechanisms : Some transformations may proceed via radical pathways, especially under photochemical or thermal conditions.
Example Reaction Conditions
-
Cycloaddition : The use of copper(I) iodide as a catalyst has been shown to optimize yields significantly when synthesizing triazole derivatives from this compound.
-
Alkylation : Employing various bases such as sodium hydride or potassium carbonate enhances the nucleophilicity of the amino group for effective alkylation.
Scientific Research Applications
Peptide Synthesis
(R)-alpha-Propargylalanine is widely utilized in peptide synthesis due to its ability to participate in various coupling reactions. It serves as a valuable building block for the generation of peptides with specific functionalities. The incorporation of this compound into peptides can improve their stability and bioactivity.
Application | Description |
---|---|
Peptide Coupling | Used in solid-phase peptide synthesis to create complex peptides with desired properties. |
Functionalization | Allows for the introduction of diverse functional groups into peptide chains. |
Stability Enhancement | Modifications using this compound can enhance the metabolic stability of peptides. |
Drug Development
The compound has shown promise as a precursor for developing drugs targeting various diseases, including cancer and neurological disorders. Its ability to form stable conjugates with other pharmacophores makes it an attractive candidate for drug design.
- Anticancer Agents : Research indicates that peptides incorporating this compound exhibit cytotoxic effects against certain cancer cell lines, suggesting potential as anticancer agents.
- Neuroprotective Compounds : Studies have explored its use in creating neuroprotective peptides that may help in conditions like Alzheimer's disease.
Click Chemistry
This compound is particularly significant in click chemistry, where it can be used to create bioconjugates through copper-catalyzed azide-alkyne cycloaddition reactions. This application is crucial for developing targeted delivery systems in drug therapy.
Click Chemistry Application | Details |
---|---|
Bioconjugation | Facilitates the attachment of drugs or imaging agents to biomolecules for targeted delivery. |
Labeling Techniques | Used in labeling biomolecules for tracking and imaging in biological systems. |
Biological Studies
The compound's unique properties allow researchers to study protein interactions and enzymatic activities, providing insights into biochemical pathways and cellular mechanisms.
- Enzyme Inhibition Studies : this compound has been investigated as an inhibitor for specific enzymes, contributing to understanding metabolic pathways.
- Protein Modification : Its incorporation into proteins can help elucidate structure-function relationships within biological systems.
Case Studies
Several case studies have highlighted the effectiveness of this compound in various applications:
- Synthesis of Anticancer Peptides : A study demonstrated that incorporating this compound into a peptide sequence resulted in enhanced cytotoxicity against breast cancer cells compared to standard peptides without this modification.
- Neuroprotective Peptide Development : Research focused on designing neuroprotective peptides using this compound showed promising results in reducing neuronal cell death in vitro under oxidative stress conditions.
- Targeted Drug Delivery Systems : A case study illustrated the successful use of this compound in creating bioconjugates that selectively delivered chemotherapeutic agents to tumor cells, improving therapeutic efficacy while minimizing side effects.
Mechanism of Action
The mechanism of action of ®-alpha-Propargylalanine involves its interaction with specific molecular targets, such as enzymes and receptors. The propargyl group can form covalent bonds with active site residues, leading to inhibition or modulation of enzyme activity. This interaction can affect various biochemical pathways, making ®-alpha-Propargylalanine a valuable tool in studying enzyme function and developing therapeutic agents.
Comparison with Similar Compounds
Comparison with Structurally Similar Compounds
Structural and Physicochemical Properties
The table below compares (R)-alpha-Propargylalanine with its closest structural analogs, focusing on key chemical attributes:
Key Observations:
Stereochemical Differentiation: this compound and (S)-3-Amino-5-hexynoic acid HCl share the same molecular formula but differ in stereochemistry (R vs. S) and propargyl group placement (alpha vs. beta carbon). These differences significantly impact their reactivity and biological activity . The (R)-enantiomer of propargylalanine is preferred in asymmetric catalysis due to its compatibility with chiral enzyme active sites, whereas the (S)-enantiomer of hexynoic acid is often used in peptide backbone modifications .
Functional Group Reactivity :
- The terminal alkyne in This compound enables efficient Huisgen cycloaddition (click chemistry), making it superior to fluorinated analogs like 4-Fluoro-L-proline methyl ester HCl , which lack this reactive handle .
Pharmacological and Industrial Relevance
This compound :
- Enzyme Inhibition: Acts as a mechanism-based inhibitor of pyridoxal phosphate-dependent enzymes, such as gamma-aminobutyric acid (GABA) transaminase, due to its propargyl moiety .
- Synthetic Utility : Used in the synthesis of peptide-drug conjugates for targeted cancer therapies, leveraging its bioorthogonal reactivity .
(S)-3-Amino-5-hexynoic acid HCl :
- Peptide Engineering : Incorporation into peptide chains introduces rigidity and stability via alkyne-mediated crosslinking, though its beta-carbon propargyl placement reduces steric accessibility compared to alpha-substituted analogs .
Biological Activity
(R)-alpha-Propargylalanine (R-PA) is a non-proteinogenic amino acid that has garnered attention in recent years due to its diverse biological activities. This article provides a comprehensive overview of the biological properties, synthesis, and potential applications of R-PA, supported by data tables and findings from relevant studies.
- Molecular Formula : CHNO
- Molecular Weight : 127.14 g/mol
- CAS Number : 403519-98-2
R-PA is characterized by a propargyl group attached to the alpha carbon of alanine, which contributes to its unique reactivity and biological functions.
1. Antimicrobial Properties
R-PA and its derivatives have been investigated for their antimicrobial activity. Studies indicate that compounds containing the propargyl moiety exhibit significant antibacterial effects against various pathogens.
The mechanism of action is primarily attributed to membrane disruption, leading to cell lysis and inhibition of essential metabolic processes.
2. Role in Cancer Research
R-PA has shown promise in cancer research, particularly in the development of peptide-based therapeutics. Its ability to modify peptide structures allows for enhanced interactions with cancer cell targets.
- Case Study : A study on triazole-stapled peptides demonstrated that incorporating R-PA into peptide sequences improved binding affinity to target proteins involved in cancer progression .
3. Neurological Applications
Recent research has explored the neuroprotective properties of R-PA. It has been noted for its potential in treating neurodegenerative diseases by modulating mitochondrial function.
Application | Mechanism | Disease Model | Reference |
---|---|---|---|
Neuroprotection | Mitochondrial modulation | Alzheimer's disease | AU2017249218A1 |
Oxidative stress reduction | Antioxidant effects | Parkinson's disease | AU2017249218A1 |
Synthesis Methods
The synthesis of R-PA can be achieved through several methods, including:
- Click Chemistry : Utilizing copper-catalyzed azide-alkyne cycloaddition (CuAAC) to create functionalized derivatives.
- Chiral Synthesis : Employing chiral catalysts to ensure enantiomeric purity, which is crucial for biological activity.
Research Findings
Recent studies have highlighted the versatility of R-PA in various biochemical applications:
Q & A
Basic Research Questions
Q. What are the established synthetic routes for (R)-alpha-Propargylalanine, and how can researchers ensure reproducibility?
this compound is typically synthesized via asymmetric catalysis or enzymatic resolution of racemic mixtures. Key steps include propargylation of protected amino acid precursors followed by chiral separation. To ensure reproducibility, document reaction parameters (temperature, solvent purity, catalyst loading) and validate intermediates using NMR and mass spectrometry. Adhere to NIH guidelines for reporting experimental conditions, including raw data and statistical thresholds for enantiomeric excess (EE) calculations .
Q. Which analytical techniques are most effective for characterizing the enantiomeric purity of this compound?
Chiral HPLC with polysaccharide-based columns (e.g., Chiralpak AD-H) is the gold standard. Confirm results using circular dichroism (CD) spectroscopy or X-ray crystallography. For quantitative EE determination, integrate chromatographic peaks and apply the formula: . Report calibration curves and limit of detection (LOD) to comply with preclinical reproducibility standards .
Q. What role does this compound play in click chemistry applications, and how should such experiments be designed?
The propargyl group enables copper-catalyzed azide-alkyne cycloaddition (CuAAC) for bioconjugation. Design experiments by:
- Optimizing molar ratios of this compound to azide-functionalized biomolecules.
- Controlling reaction pH (6.5–7.5) to preserve protein integrity.
- Validating conjugation efficiency via SDS-PAGE or MALDI-TOF. Include negative controls (e.g., non-propargylated analogs) to confirm specificity .
Advanced Research Questions
Q. How can researchers optimize reaction conditions to minimize racemization during the synthesis of this compound?
Racemization occurs under basic or high-temperature conditions. Mitigate this by:
- Using mild deprotection agents (e.g., TFA/DCM at 0°C).
- Employing low-temperature asymmetric hydrogenation with Ru-BINAP catalysts.
- Monitoring optical rotation dynamically to detect early-stage racemization. Compare kinetic data across trials to identify critical thresholds .
Q. What strategies resolve contradictions in reported biological activities of this compound across different studies?
Contradictions often arise from variability in assay conditions (e.g., enzyme sources, buffer compositions). Address this by:
- Replicating assays under standardized protocols (e.g., uniform ATP concentration in kinase inhibition studies).
- Applying meta-analysis to aggregate data and identify confounding variables.
- Validating findings using orthogonal methods (e.g., SPR for binding affinity vs. enzymatic activity assays) .
Q. How can computational modeling enhance the design of this compound derivatives for targeted enzyme inhibition?
Use molecular docking (AutoDock Vina) to predict binding poses with active sites. Prioritize derivatives with:
- Favorable ΔG binding energies (< -8 kcal/mol).
- Hydrogen-bonding interactions with catalytic residues.
- Validate predictions via IC₅₀ assays and crystallographic studies. Cross-reference results with databases like PDB to avoid overfitting .
Q. How should researchers assess the stability of this compound under physiological and experimental storage conditions?
Conduct accelerated stability studies:
- Expose the compound to pH 2–9 buffers at 37°C for 24–72 hours.
- Analyze degradation products via LC-MS and assign structures using fragmentation patterns.
- For long-term storage, lyophilize in argon-filled vials at -80°C. Report degradation kinetics (Arrhenius plots) to extrapolate shelf-life .
Q. Methodological Frameworks
- Experimental Design : Align with FINER criteria (Feasible, Interesting, Novel, Ethical, Relevant). For example, a novel application in peptide stapling must demonstrate synthetic feasibility and biological relevance .
- Data Analysis : Use Shapiro-Wilk tests for normality before applying parametric statistics. For non-linear kinetics (e.g., enzyme inhibition), fit data to Hill equations .
Properties
IUPAC Name |
2-amino-2-methylpent-4-ynoic acid | |
---|---|---|
Details | Computed by Lexichem TK 2.7.0 (PubChem release 2021.05.07) | |
Source | PubChem | |
URL | https://pubchem.ncbi.nlm.nih.gov | |
Description | Data deposited in or computed by PubChem | |
InChI |
InChI=1S/C6H9NO2/c1-3-4-6(2,7)5(8)9/h1H,4,7H2,2H3,(H,8,9) | |
Details | Computed by InChI 1.0.6 (PubChem release 2021.05.07) | |
Source | PubChem | |
URL | https://pubchem.ncbi.nlm.nih.gov | |
Description | Data deposited in or computed by PubChem | |
InChI Key |
FSBNDYYRTZBHAN-UHFFFAOYSA-N | |
Details | Computed by InChI 1.0.6 (PubChem release 2021.05.07) | |
Source | PubChem | |
URL | https://pubchem.ncbi.nlm.nih.gov | |
Description | Data deposited in or computed by PubChem | |
Canonical SMILES |
CC(CC#C)(C(=O)O)N | |
Details | Computed by OEChem 2.3.0 (PubChem release 2021.05.07) | |
Source | PubChem | |
URL | https://pubchem.ncbi.nlm.nih.gov | |
Description | Data deposited in or computed by PubChem | |
Molecular Formula |
C6H9NO2 | |
Details | Computed by PubChem 2.1 (PubChem release 2021.05.07) | |
Source | PubChem | |
URL | https://pubchem.ncbi.nlm.nih.gov | |
Description | Data deposited in or computed by PubChem | |
DSSTOX Substance ID |
DTXSID20665918 | |
Record name | 2-Amino-2-methylpent-4-ynoic acid | |
Source | EPA DSSTox | |
URL | https://comptox.epa.gov/dashboard/DTXSID20665918 | |
Description | DSSTox provides a high quality public chemistry resource for supporting improved predictive toxicology. | |
Molecular Weight |
127.14 g/mol | |
Details | Computed by PubChem 2.1 (PubChem release 2021.05.07) | |
Source | PubChem | |
URL | https://pubchem.ncbi.nlm.nih.gov | |
Description | Data deposited in or computed by PubChem | |
CAS No. |
403519-98-2 | |
Record name | 2-Amino-2-methylpent-4-ynoic acid | |
Source | EPA DSSTox | |
URL | https://comptox.epa.gov/dashboard/DTXSID20665918 | |
Description | DSSTox provides a high quality public chemistry resource for supporting improved predictive toxicology. | |
Disclaimer and Information on In-Vitro Research Products
Please be aware that all articles and product information presented on BenchChem are intended solely for informational purposes. The products available for purchase on BenchChem are specifically designed for in-vitro studies, which are conducted outside of living organisms. In-vitro studies, derived from the Latin term "in glass," involve experiments performed in controlled laboratory settings using cells or tissues. It is important to note that these products are not categorized as medicines or drugs, and they have not received approval from the FDA for the prevention, treatment, or cure of any medical condition, ailment, or disease. We must emphasize that any form of bodily introduction of these products into humans or animals is strictly prohibited by law. It is essential to adhere to these guidelines to ensure compliance with legal and ethical standards in research and experimentation.