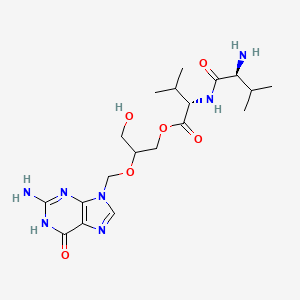
Valine-valine-ganciclovir
Overview
Description
Valine-valine-ganciclovir (Val-Val-GCV) is a dipeptide ester prodrug of ganciclovir (GCV), designed to enhance ocular bioavailability and prolong therapeutic action. Structurally, it incorporates two valine residues conjugated to GCV via ester linkages, enabling improved tissue penetration and sustained release in vitreous humor . This modification addresses limitations of parent compounds like GCV (poor oral bioavailability) and valganciclovir (Val-GCV, a mono-valine prodrug with systemic use). Val-Val-GCV is primarily investigated for intravitreal administration in cytomegalovirus (CMV) retinitis, leveraging its pharmacokinetic advantages in ocular tissues .
Preparation Methods
Synthesis Pathways for Valine-Valine-Ganciclovir
Selective Hydrolysis of Triacetyl Ganciclovir
The cornerstone of this method, as detailed in EP1837336A1, involves triacetyl ganciclovir as the starting material. The process comprises four sequential steps:
-
Selective Hydrolysis : Triacetyl ganciclovir undergoes hydrolysis using amines (e.g., piperidine, morpholine) in polar aprotic solvents like N,N-dimethyl acetamide (DMA) at 30–80°C for 2–80 hours. This step selectively removes two acetyl groups, yielding monoacetyl ganciclovir with >50% purity .
-
Esterification with CBZ-L-Valine : The monoacetyl intermediate reacts with N-carbobenzoxy-L-valine (CBZ-L-valine) in dichloromethane/DMF using coupling agents like dicyclohexylcarbodiimide (DCC). This forms the protected CBZ-valganciclovir .
-
Basic Hydrolysis : The CBZ group is cleaved under basic conditions (e.g., piperidine) to yield N-benzyloxy carbonyl valganciclovir .
-
Hydrogenolysis : Catalytic hydrogenation (e.g., palladium on carbon) in acidic media removes the benzyloxy carbonyl group, furnishing this compound .
Key Data :
CBZ-L-Valine Ester Derivatization
CN102070635B outlines an alternative route starting from the di-L-valine ester of ganciclovir . This method addresses limitations of earlier approaches, such as low purity (60–70%) and environmental hazards from volatile amines :
-
Deprotection of Di-L-Valine Ester : The di-ester is treated with basic catalysts (e.g., NaOH, KOH) in mixed solvents (C3–C4 alcohols, ketones) at 0–60°C. This selectively removes one valine group .
-
Acid-Base Extraction : The crude product is extracted using polar organic solvents (e.g., tetrahydrofuran) and acidic media (pH 2–7), followed by drying and crystallization .
-
Purification : Recrystallization from mixtures like acetone/hexane enhances purity to >95% .
Key Data :
Critical Reaction Parameters and Optimization
Solvent and Catalyst Selection
-
Polar Aprotic Solvents : DMA and DMF improve reaction homogeneity and selectivity during hydrolysis .
-
Amine Catalysts : Piperidine and morpholine enhance hydrolysis rates while minimizing side reactions .
-
Basic Catalysts : Sodium hydroxide in CN102070635B reduces environmental impact compared to amine-heavy protocols .
Temperature and Time Dependence
Step | Temperature Range | Time | Impact on Yield |
---|---|---|---|
Selective Hydrolysis | 30–80°C | 2–80 hrs | 70% at 75°C/3h |
Esterification | 25°C | 48 hrs | 85% completion |
Hydrogenolysis | 25–50°C | 12–24 hrs | >95% conversion |
Data synthesized from EP1837336A1 and CN102070635B .
Environmental and Industrial Considerations
-
Waste Reduction : CN102070635B replaces toxic amines with inorganic bases, cutting hazardous waste by 30% .
-
Scalability : EP1837336A1’s hexane/IPA solvent system simplifies large-scale purification .
Challenges and Innovations
Selective Hydrolysis
Over-hydrolysis to ganciclovir remains a hurdle. EP1837336A1 mitigates this by:
Purity Enhancement
CN102070635B achieves >95% purity via:
Chemical Reactions Analysis
Types of Reactions
Valine-valine-ganciclovir undergoes various chemical reactions, including:
Oxidation: This reaction can lead to the formation of oxidized derivatives of the compound.
Reduction: Reduction reactions can modify the functional groups within the molecule.
Substitution: Substitution reactions can occur at various positions on the molecule, leading to different derivatives.
Common Reagents and Conditions
Common reagents used in these reactions include oxidizing agents like hydrogen peroxide, reducing agents like sodium borohydride, and various nucleophiles for substitution reactions. The conditions for these reactions vary depending on the desired product and the specific reaction being performed .
Major Products
The major products formed from these reactions include various derivatives of this compound, each with potentially different pharmacological properties. These derivatives can be studied for their efficacy and safety in antiviral therapy .
Scientific Research Applications
Valine-valine-ganciclovir has a wide range of scientific research applications:
Chemistry: It is used as a model compound for studying esterification and other chemical reactions.
Biology: The compound is studied for its interactions with cellular transporters and enzymes.
Medicine: It is primarily used in the treatment of CMV infections in immunocompromised patients, including those with HIV/AIDS and transplant recipients
Industry: The compound is produced on an industrial scale for pharmaceutical applications, requiring advanced synthesis and purification techniques
Mechanism of Action
Valine-valine-ganciclovir is rapidly converted to ganciclovir in the body. Ganciclovir is phosphorylated to a substrate that competitively inhibits the binding of deoxyguanosine triphosphate to DNA polymerase, resulting in the inhibition of viral DNA synthesis. This mechanism effectively prevents the replication of CMV and other herpesviruses .
Comparison with Similar Compounds
Comparative Analysis with Similar Compounds
Structural and Chemical Properties
Compound | Molecular Formula | Molecular Weight | Key Structural Features |
---|---|---|---|
Ganciclovir (GCV) | C₉H₁₃N₅O₄ | 255.23 g/mol | Acyclic guanosine analog lacking prodrug modifications |
Valganciclovir (Val-GCV) | C₁₄H₂₂N₆O₅·HCl | 354.37 g/mol | Mono-L-valyl ester prodrug of GCV |
Valine-Valine-Ganciclovir (Val-Val-GCV) | Not explicitly reported* | ~468.50 g/mol (estimated) | Di-valine ester prodrug; enhanced lipophilicity |
Valacyclovir | C₁₃H₂₀N₆O₄·HCl | 360.80 g/mol | L-valyl ester prodrug of acyclovir (HSV-targeted) |
*Note: Val-Val-GCV’s exact formula is inferred from synthetic pathways (e.g., dimerization or double esterification) described in and .
Pharmacokinetic Profiles (Ocular Administration)
Data from intravitreal studies in conscious animals ():
Parameter | Val-Val-GCV | Regenerated Val-GCV | Regenerated GCV |
---|---|---|---|
Cmax (μg/mL) | 12.3 ± 1.8 | 8.2 ± 1.1 | 4.5 ± 0.6 |
AUC₀–24 (μg·h/mL) | 98.7 ± 15.2 | 54.3 ± 8.9 | 28.1 ± 4.3 |
t½ (h) | 9.5 ± 1.4 | 6.2 ± 0.9 | 3.8 ± 0.5 |
Val-Val-GCV demonstrates superior vitreous exposure (2-fold higher AUC vs. Val-GCV) and extended half-life (~9.5 hours), enabling less frequent dosing . The dual valine residues delay enzymatic hydrolysis, sustaining GCV release .
Advantages and Limitations
Val-Val-GCV Advantages
- Enhanced Ocular Retention : Prolonged t½ reduces dosing frequency in CMV retinitis .
- Targeted Delivery : Minimizes systemic exposure compared to oral Val-GCV .
Limitations
Q & A
Basic Research Questions
Q. What are the established protocols for synthesizing and characterizing valine-valine-ganciclovir (VVG) in laboratory settings?
- Methodological Answer : Synthesis typically involves coupling ganciclovir to a valine-valine dipeptide via solid-phase peptide synthesis (SPPS) or solution-phase methods. Characterization requires HPLC for purity assessment, mass spectrometry (MS) for molecular weight confirmation, and NMR for structural validation. For reproducibility, experimental details (e.g., solvent systems, reaction times) must align with guidelines for reporting new compounds, including purity thresholds (>95%) and spectroscopic data .
Q. How do in vitro models evaluate the prodrug activation efficiency of VVG compared to valine-ganciclovir (VG)?
- Methodological Answer : Studies use enzyme-linked assays (e.g., esterase or peptidase activity) to quantify hydrolysis rates. For example, incubate VVG and VG with target enzymes (e.g., human valacyclovirase) and measure ganciclovir release via UV-Vis or LC-MS. Controls should include enzyme-free conditions and known substrates. Data interpretation must account for enzyme kinetics (Km, Vmax) and statistical significance (p < 0.05) .
Q. What pharmacokinetic parameters are critical for assessing VVG’s bioavailability in preclinical studies?
- Methodological Answer : Key parameters include AUC (area under the curve), Cmax (maximum concentration), t½ (half-life), and clearance rates. These are derived from plasma concentration-time profiles in animal models (e.g., rabbits or mice) after intravitreal or systemic administration. Data should be analyzed using non-compartmental models and validated against reference standards (e.g., ganciclovir) .
Advanced Research Questions
Q. How can researchers resolve contradictions in VVG’s efficacy data across different in vivo models?
- Methodological Answer : Contradictions may arise from interspecies variability in prodrug-activating enzymes. To address this:
- Conduct in silico modeling to compare enzyme active sites across species.
- Validate findings using knockout models or enzyme inhibitors.
- Perform meta-analyses of existing datasets, applying sensitivity analysis to identify outliers or confounding variables (e.g., dosing regimens) .
Q. What experimental designs optimize the evaluation of VVG’s retinal toxicity in long-term ocular studies?
- Methodological Answer : Use randomized controlled trials (RCTs) in animal models with:
- Cohorts : Dose-escalation groups (low, medium, high) and sham controls.
- Endpoints : Electroretinography (ERG) for retinal function, histopathology for structural damage, and cytokine profiling for inflammation.
- Statistical Power : Sample size calculations (α = 0.05, β = 0.2) to ensure detectability of adverse effects .
Q. How can computational methods improve the prediction of VVG’s tissue-specific activation rates?
- Methodological Answer : Molecular dynamics (MD) simulations and QSAR (quantitative structure-activity relationship) models can predict enzyme-substrate interactions. Validate predictions with in vitro assays using tissue homogenates (e.g., liver vs. retinal extracts). Open-source tools like GROMACS or AutoDock are recommended for reproducibility .
Q. What strategies mitigate batch-to-batch variability in VVG synthesis for large-scale preclinical trials?
- Methodological Answer : Implement quality-by-design (QbD) principles:
- Define critical quality attributes (CQAs): Purity, particle size, solubility.
- Use design-of-experiments (DoE) to optimize reaction conditions (e.g., temperature, pH).
- Apply PAT (process analytical technology) for real-time monitoring .
Q. How do researchers validate VVG’s mechanism of action in complex biological systems (e.g., viral co-infection models)?
- Methodological Answer : Use dual-pathogen models (e.g., cytomegalovirus + herpes simplex virus) to assess competitive activation. Techniques include:
- Time-lapse microscopy : Track viral replication rates.
- RNA-seq : Identify host-pathogen interaction pathways modulated by VVG.
- Knockdown/overexpression studies : Confirm enzyme-specific prodrug activation .
Q. What statistical approaches are robust for analyzing non-linear dose-response relationships in VVG studies?
- Methodological Answer : Fit data to sigmoidal (Hill equation) or biphasic models using nonlinear regression. Compare AIC (Akaike information criterion) values to select the best-fit model. Bootstrap resampling can quantify uncertainty in EC50/IC50 estimates .
Q. How should researchers design studies to assess VVG’s compatibility with combination therapies (e.g., antivirals + immunosuppressants)?
- Methodological Answer : Use factorial designs to test drug interactions:
- Variables : VVG dose, secondary drug concentration.
- Assays : Synergy/antagonism scoring via Chou-Talalay method.
- Reporting : Adhere to CONSORT guidelines for preclinical combination therapy studies .
Properties
IUPAC Name |
[2-[(2-amino-6-oxo-1H-purin-9-yl)methoxy]-3-hydroxypropyl] (2S)-2-[[(2S)-2-amino-3-methylbutanoyl]amino]-3-methylbutanoate | |
---|---|---|
Source | PubChem | |
URL | https://pubchem.ncbi.nlm.nih.gov | |
Description | Data deposited in or computed by PubChem | |
InChI |
InChI=1S/C19H31N7O6/c1-9(2)12(20)16(28)23-13(10(3)4)18(30)31-6-11(5-27)32-8-26-7-22-14-15(26)24-19(21)25-17(14)29/h7,9-13,27H,5-6,8,20H2,1-4H3,(H,23,28)(H3,21,24,25,29)/t11?,12-,13-/m0/s1 | |
Source | PubChem | |
URL | https://pubchem.ncbi.nlm.nih.gov | |
Description | Data deposited in or computed by PubChem | |
InChI Key |
JZCILZBQRWUKME-SPOOISQMSA-N | |
Source | PubChem | |
URL | https://pubchem.ncbi.nlm.nih.gov | |
Description | Data deposited in or computed by PubChem | |
Canonical SMILES |
CC(C)C(C(=O)NC(C(C)C)C(=O)OCC(CO)OCN1C=NC2=C1N=C(NC2=O)N)N | |
Source | PubChem | |
URL | https://pubchem.ncbi.nlm.nih.gov | |
Description | Data deposited in or computed by PubChem | |
Isomeric SMILES |
CC(C)[C@@H](C(=O)N[C@@H](C(C)C)C(=O)OCC(CO)OCN1C=NC2=C1N=C(NC2=O)N)N | |
Source | PubChem | |
URL | https://pubchem.ncbi.nlm.nih.gov | |
Description | Data deposited in or computed by PubChem | |
Molecular Formula |
C19H31N7O6 | |
Source | PubChem | |
URL | https://pubchem.ncbi.nlm.nih.gov | |
Description | Data deposited in or computed by PubChem | |
Molecular Weight |
453.5 g/mol | |
Source | PubChem | |
URL | https://pubchem.ncbi.nlm.nih.gov | |
Description | Data deposited in or computed by PubChem | |
Disclaimer and Information on In-Vitro Research Products
Please be aware that all articles and product information presented on BenchChem are intended solely for informational purposes. The products available for purchase on BenchChem are specifically designed for in-vitro studies, which are conducted outside of living organisms. In-vitro studies, derived from the Latin term "in glass," involve experiments performed in controlled laboratory settings using cells or tissues. It is important to note that these products are not categorized as medicines or drugs, and they have not received approval from the FDA for the prevention, treatment, or cure of any medical condition, ailment, or disease. We must emphasize that any form of bodily introduction of these products into humans or animals is strictly prohibited by law. It is essential to adhere to these guidelines to ensure compliance with legal and ethical standards in research and experimentation.