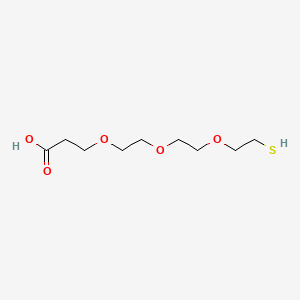
Thiol-PEG3-acid
Overview
Description
Thiol-PEG3-acid (CAS: 1347750-82-6) is a trifunctional polyethylene glycol (PEG) derivative containing a terminal thiol (-SH) group, a triethylene glycol (PEG3) spacer, and a carboxylic acid (-COOH) group. Key properties include:
- Molecular Formula: C₉H₁₈O₅S
- Molecular Weight: 238.3 g/mol
- Purity: ≥95%
- Storage: -20°C in dry conditions .
The thiol group enables conjugation with maleimide or gold surfaces, while the carboxylic acid facilitates carbodiimide-mediated coupling to amines. Applications span bioconjugation, drug delivery, and surface functionalization due to its biocompatibility and solubility .
Preparation Methods
Synthetic Routes for Thiol-PEG3-Acid
The synthesis of this compound involves sequential modifications of PEG precursors to introduce thiol and carboxylic acid functionalities. Two primary routes dominate current methodologies:
Stepwise Coupling via Carbodiimide Chemistry
This route begins with a PEG3 diol precursor. The hydroxyl group at one terminus is converted to a carboxylic acid, while the other is functionalized with a protected thiol. Key steps include:
-
Activation of PEG3 Diol :
-
Thiol Introduction :
Critical Parameters :
-
Reaction temperature: 0–25°C to minimize disulfide formation.
-
Solvent choice: DCM or tetrahydrofuran (THF) for optimal solubility.
Direct Functionalization of Pre-Modified PEG3
Commercial PEG3 derivatives with pre-installed functional groups (e.g., PEG3-amine or PEG3-maleimide) serve as intermediates:
-
Carboxylic Acid Installation :
-
Thiol Incorporation :
Advantages :
-
Reduced side reactions due to orthogonal protection strategies.
Reaction Conditions and Optimization
Solvent Systems
Solvent | Role | Optimal Concentration | Impact on Yield |
---|---|---|---|
DCM | Activation/coupling reactions | Anhydrous, 0.1–0.5 M | High (80–90%) |
DMF/DMSO | Dissolving PEG intermediates | 10–20% v/v | Moderate (70%) |
THF | Thiolation reactions | 0.2–0.4 M | High (85%) |
Source: Adapted from conjugation protocols in and .
Catalysts and Reagents
-
EDC/NHS : Enhances carboxylic acid activation efficiency by forming stable N-hydroxysuccinimide esters .
-
DMAP : Accelerates acylation reactions by deprotonating hydroxyl groups.
-
Triethylamine (TEA) : Neutralizes HCl byproducts during thiolation, preventing acid-catalyzed disulfide formation .
Optimization Findings :
-
Excess EDC (>1.2 eq) leads to crosslinking; stoichiometric ratios (1.0–1.1 eq) maximize yield .
-
Reaction times >24 hours at 25°C reduce thiol purity due to oxidation .
Purification and Characterization
Purification Techniques
-
Size-Exclusion Chromatography (SEC) :
-
Reverse-Phase HPLC :
Purity Outcomes :
Characterization Data
Technique | Key Observations | Functional Group Confirmation |
---|---|---|
¹H NMR | δ 1.65 ppm (SH, triplet), δ 2.40 ppm (-COOH) | Thiol and carboxylic acid |
FT-IR | 2560 cm⁻¹ (S-H stretch), 1710 cm⁻¹ (C=O) | Thiol and acid |
Mass Spec | [M+H]⁺ = 239.2 m/z | Molecular weight verification |
Industrial-Scale Production Considerations
Batch vs. Continuous Flow Synthesis
Parameter | Batch Reactors | Continuous Flow Systems |
---|---|---|
Yield | 70–80% | 85–90% |
Throughput | Low (1–5 kg/day) | High (20–50 kg/day) |
Oxidation Risk | Moderate (manual handling) | Low (inert atmosphere) |
Source: Industrial protocols from .
Cost Drivers
-
Raw Materials : PEG3 diol (~$120/kg) accounts for 60% of total costs.
-
Catalysts : EDC/NHS adds ~$25/kg to production.
Challenges and Mitigation Strategies
-
Thiol Oxidation :
-
PEG Hydrolysis :
Emerging Innovations
Enzymatic Synthesis
-
Lipase-Catalyzed Coupling :
Flow Chemistry Advancements
Chemical Reactions Analysis
Types of Reactions:
Oxidation: Thiol groups can be oxidized to form disulfides.
Reduction: Disulfides can be reduced back to thiols.
Substitution: Thiol groups can undergo nucleophilic substitution reactions with alkyl halides to form thioethers.
Common Reagents and Conditions:
Oxidation: Mild oxidants like iodine (I2) or hydrogen peroxide (H2O2) are used.
Reduction: Reducing agents like dithiothreitol (DTT) or tris(2-carboxyethyl)phosphine (TCEP) are used.
Substitution: Alkyl halides are used in the presence of a base like sodium hydroxide (NaOH).
Major Products:
Disulfides: Formed from the oxidation of thiols.
Thioethers: Formed from the substitution reactions of thiols with alkyl halides
Scientific Research Applications
Drug Development
Thiol-PEG3-acid is extensively used in the development of Proteolysis Targeting Chimeras (PROTACs). PROTACs are bifunctional molecules that recruit E3 ubiquitin ligases to target proteins for degradation. This compound acts as a linker between the ligand for the target protein and the ligand for the E3 ligase, facilitating selective degradation of disease-related proteins.
Case Study: PROTAC Synthesis
A study demonstrated the synthesis of a PROTAC using this compound as a linker, which effectively targeted and degraded an oncogenic protein in cancer cells. The results indicated a significant reduction in cell viability, showcasing the potential of this approach in cancer therapy .
Bioconjugation Techniques
This compound is crucial in bioconjugation strategies, particularly in the modification of oligonucleotides and peptides. Its thiol group allows for specific conjugation to maleimide-modified oligonucleotides, enhancing their delivery and therapeutic efficacy.
Case Study: Oligonucleotide Conjugation
In research focused on enhancing oligonucleotide delivery, this compound was conjugated to various thiol-bearing molecules. The study reported high conversion rates and successful delivery of modified oligonucleotides to target cells, improving their therapeutic potential .
Nanotechnology Applications
This compound plays a vital role in nanotechnology, particularly in the development of drug delivery systems and nanomaterials. Its ability to create stable linkages with nanoparticles enhances their biocompatibility and targeting capabilities.
Case Study: Nanoparticle Functionalization
Research involving gold nanoparticles functionalized with this compound demonstrated improved stability and targeted delivery of anticancer drugs. The study highlighted that these modified nanoparticles exhibited enhanced cellular uptake and therapeutic efficacy compared to non-modified controls .
Medical Research Applications
In medical research, this compound is utilized for creating functional coatings on biomaterials and drug carriers. This application is particularly important for improving the interaction between drugs and biological systems.
Example: Coating Biomaterials
A study explored the use of this compound to coat implantable devices, which resulted in reduced immune response and improved integration with surrounding tissues. This application underscores its potential in regenerative medicine .
Mechanism of Action
Thiol-PEG3-acid exerts its effects through the formation of stable covalent bonds with target molecules. The thiol group reacts with electrophilic groups such as maleimide, orthopyridyl disulfide, and vinylsulfone to form thioether bonds. The carboxylic acid group reacts with primary amines to form amide bonds. These reactions result in the modification of the target molecules, enhancing their solubility, stability, and functionality .
Comparison with Similar Compounds
Thiol-PEG3-Phosphonic Acid Ethyl Ester
- Molecular Formula : C₁₂H₂₇O₆PS
- Molecular Weight : 330.4 g/mol
- Functional Groups : Thiol (-SH), phosphonic acid ethyl ester (-PO₃Et)
- Purity : 90%
- Storage : -20°C .
Key Differences :
- The phosphonic acid ethyl ester group enhances metal-binding affinity (e.g., titanium or iron oxides), making it suitable for surface coatings and nanomaterials.
- Lower purity (90%) compared to Thiol-PEG3-acid (≥95%) may limit reproducibility in sensitive applications.
- Applications: Nanomaterial functionalization, metal-organic frameworks .
Thiol-PEG3-Azide
- Molecular Formula : C₈H₁₇N₃O₃S
- Molecular Weight : 235.1 g/mol
- Functional Groups : Thiol (-SH), azide (-N₃)
- Purity : ≥95%
- Storage : -5°C .
Key Differences :
- The azide group enables copper-catalyzed azide-alkyne cycloaddition (CuAAC), a cornerstone of "click chemistry."
- Shorter PEG spacer (PEG3 vs. PEG12 in longer derivatives) reduces steric hindrance, favoring rapid conjugation.
- Applications: Targeted drug delivery, fluorescent labeling, and polymer synthesis .
Thiol-PEG2-Acid
- Molecular Formula : C₇H₁₄O₄S
- Molecular Weight : 194.25 g/mol
- Functional Groups : Thiol (-SH), carboxylic acid (-COOH)
- Purity : ≥95%
- Storage : -20°C .
Key Differences :
- Shorter PEG chain (PEG2 vs. PEG3) reduces hydrophilicity and in vivo circulation time.
- Ideal for applications requiring minimal spacer length, such as small-molecule conjugation or surface grafting .
Thiol-PEG12-Acid
- Molecular Formula : C₂₇H₅₄O₁₄S
- Molecular Weight : 634.78 g/mol
- Functional Groups : Thiol (-SH), carboxylic acid (-COOH)
- Purity : ≥95%
- Storage : -20°C .
Key Differences :
- Extended PEG chain (PEG12) enhances water solubility and reduces immunogenicity, critical for nanoparticle stabilization.
- Larger molecular weight may limit tissue penetration but improves pharmacokinetics in systemic delivery .
Comparative Data Table
Compound | Functional Groups | PEG Length | Molecular Weight (g/mol) | Purity | Key Applications |
---|---|---|---|---|---|
This compound | -SH, -COOH | PEG3 | 238.3 | ≥95% | Bioconjugation, drug delivery |
Thiol-PEG3-Phosphonic Acid | -SH, -PO₃Et | PEG3 | 330.4 | 90% | Metal-organic frameworks, coatings |
Thiol-PEG3-Azide | -SH, -N₃ | PEG3 | 235.1 | ≥95% | Click chemistry, fluorescent probes |
Thiol-PEG2-acid | -SH, -COOH | PEG2 | 194.25 | ≥95% | Small-molecule conjugation |
Thiol-PEG12-acid | -SH, -COOH | PEG12 | 634.78 | ≥95% | Nanoparticle stabilization, PEGylation |
Biological Activity
Thiol-PEG3-acid is a bifunctional compound that plays a significant role in the field of bioconjugation and drug development, particularly in the synthesis of Proteolysis Targeting Chimeras (PROTACs). This article explores its biological activity, structural characteristics, synthesis methods, and applications in various research domains.
Structural Characteristics
This compound is characterized by its unique structure, which includes:
- Molecular Formula : C₉H₁₈O₅S
- Molecular Weight : Approximately 238.3 g/mol
- Functional Groups : It contains a thiol group (-SH) and a terminal carboxylic acid (-COOH), which are crucial for its reactivity and conjugation capabilities.
The polyethylene glycol (PEG) backbone enhances the compound's solubility and flexibility, making it suitable for various biological applications. The hydrophilic nature of PEG improves the circulation time of conjugates in biological systems, thus enhancing their therapeutic potential.
This compound functions primarily as a linker in PROTAC molecules. PROTACs are designed to target specific proteins for degradation via the ubiquitin-proteasome system (UPS). The compound's ability to form stable conjugates with proteins and other biomolecules is vital for:
- Enhancing the delivery of therapeutic agents.
- Improving the pharmacokinetic properties of drugs.
- Facilitating selective labeling in diagnostic assays .
Biological Activity and Applications
This compound exhibits significant biological activity due to its reactivity with thiol-bearing molecules. Its applications include:
- Drug Delivery Systems : By conjugating drugs to proteins or antibodies, this compound enhances the specificity and efficacy of treatments.
- Diagnostic Assays : The compound is used to label biomolecules for imaging and detection purposes.
- Gene Therapy : It aids in the delivery of oligonucleotides by improving their stability and cellular uptake .
Case Studies
- Oligonucleotide Conjugation : In a study involving the conjugation of oligonucleotides with thiol-bearing compounds, this compound was successfully used to enhance the delivery and therapeutic potential of these oligonucleotides. The reaction conditions allowed for high conversion rates, demonstrating its effectiveness in bioconjugation methodologies .
- PROTAC Development : Research has shown that incorporating this compound into PROTAC designs significantly improves their ability to degrade target proteins selectively. This was evidenced by enhanced cellular uptake and prolonged action in biological systems, making it a valuable tool in targeted therapy development .
Comparative Analysis
To better understand this compound's unique properties, here is a comparison with similar compounds:
Compound Name | Functional Groups | Unique Features |
---|---|---|
Thiol-PEG3-acetic acid | Thiol, Carboxylic Acid | Used as a linker for PROTACs similar to this compound but with an acetic acid moiety. |
Hydroxy-PEG3-thiol | Thiol, Hydroxyl | Contains an additional hydroxyl group for different reactivity patterns. |
Amino-PEG3-thiol | Thiol, Amine | Facilitates different conjugation strategies due to amino reactivity. |
Synthesis Methods
This compound can be synthesized through various methods that typically involve:
- Direct Reaction : Combining thiol-containing PEG derivatives with carboxylic acids under controlled conditions.
- Coupling Agents : Utilizing agents like EDC (1-ethyl-3-(3-dimethylaminopropyl)carbodiimide) to facilitate amide bond formation during synthesis.
These methods allow for customization based on the desired application and functionalization needs .
Q & A
Q. Basic: What are the standard protocols for synthesizing Thiol-PEG3-acid and ensuring high purity?
Answer:
this compound is typically synthesized via stepwise PEGylation, starting with the activation of the terminal acid group (e.g., using NHS esters) and subsequent thiolation. Key steps include:
- Reaction conditions : Use anhydrous solvents (e.g., DMF or DMSO) under inert atmosphere to prevent oxidation of the thiol group .
- Purification : Size-exclusion chromatography (SEC) or dialysis removes unreacted PEG precursors. Reverse-phase HPLC further isolates the target compound .
- Characterization : Confirm purity (>95%) via H/C NMR (peaks at δ 1.6–1.8 ppm for thiol protons) and mass spectrometry (e.g., MALDI-TOF) .
Q. Advanced: How can researchers optimize this compound conjugation efficiency in bioconjugation reactions?
Answer:
Thiol-maleimide or thiol-tetrazine "click chemistry" is commonly used. Optimization strategies include:
- pH control : Maintain pH 6.5–7.5 to balance thiol reactivity and stability .
- Molar ratio : Use a 1.5–2:1 excess of this compound to target molecules (e.g., antibodies) to minimize unreacted substrates .
- Kinetic monitoring : Track reaction progress via Ellman’s assay (free thiol quantification) or HPLC .
- Post-conjugation validation : Confirm linkage stability under physiological conditions (e.g., 37°C in PBS) using SDS-PAGE or fluorescence quenching assays .
Q. Basic: What analytical techniques are essential for characterizing this compound in solution?
Answer:
- Dynamic Light Scattering (DLS) : Measures hydrodynamic radius to confirm monodispersity (PDI < 0.1) .
- UV-Vis spectroscopy : Quantifies thiol concentration via absorbance at 412 nm (Ellman’s reagent method) .
- FT-IR : Identifies PEG backbone vibrations (C-O-C at 1100 cm) and thiol S-H stretches (2550–2600 cm) .
Q. Advanced: How should researchers address discrepancies in solubility data for this compound across studies?
Answer:
Solubility variations arise from differences in:
- Solvent polarity : this compound is hydrophilic (logP ≈ -1.2) but precipitates in high-ionic-strength buffers. Use co-solvents (e.g., 10% acetonitrile) for aqueous stability .
- Temperature : Solubility decreases above 25°C; pre-cool buffers to 4°C before dissolution .
- Batch variability : Compare vendor certificates of analysis (CoA) for PEG chain length and sulfhydryl content .
Systematically replicate experiments using standardized buffers (e.g., 10 mM sodium phosphate, pH 7.4) to isolate variables .
Q. Basic: What are the critical storage conditions for this compound to prevent degradation?
Answer:
- Temperature : Store lyophilized powder at -20°C in airtight, amber vials to avoid moisture and light exposure .
- Solution stability : Prepare fresh solutions for experiments; freeze aliquots at -80°C for ≤1 month .
- Thiol protection : Add 1 mM TCEP (tris(2-carboxyethyl)phosphine) to reduce disulfide formation .
Q. Advanced: How can researchers resolve conflicting data on this compound’s crosslinking efficiency in hydrogel formation?
Answer:
Conflicting data often stem from:
- PEG chain mobility : Longer PEG spacers (e.g., PEG8) reduce steric hindrance; compare with PEG3 derivatives .
- Crosslinker density : Adjust molar ratios of this compound to maleimide-functionalized polymers (e.g., 4-arm PEG-maleimide) .
- Environmental factors : Test gelation kinetics under varying oxygen levels (thiol oxidation reduces reactivity) .
Use rheometry to measure storage modulus (G’) and validate reproducibility across ≥3 independent trials .
Q. Basic: What are the primary applications of this compound in academic research?
Answer:
- Drug delivery : Conjugate chemotherapeutics (e.g., doxorubicin) via thiol-maleimide linkages for controlled release .
- Surface functionalization : Modify gold nanoparticles or quantum dots for biosensing (thiol-gold affinity) .
- Biomaterial engineering : Incorporate into hydrogels for 3D cell culture scaffolds .
Q. Advanced: How to design experiments comparing this compound with other thiolated PEG derivatives (e.g., Thiol-PEG4-acid)?
Answer:
- Variable isolation : Compare derivatives in identical reaction setups (pH, temperature, molar ratios) .
- Functional endpoints : Measure conjugation efficiency (HPLC), cytotoxicity (MTT assay), and in vivo circulation half-life .
- Statistical rigor : Use ANOVA with post-hoc Tukey tests to assess significance (p < 0.05) across ≥5 replicates .
Properties
IUPAC Name |
3-[2-[2-(2-sulfanylethoxy)ethoxy]ethoxy]propanoic acid | |
---|---|---|
Source | PubChem | |
URL | https://pubchem.ncbi.nlm.nih.gov | |
Description | Data deposited in or computed by PubChem | |
InChI |
InChI=1S/C9H18O5S/c10-9(11)1-2-12-3-4-13-5-6-14-7-8-15/h15H,1-8H2,(H,10,11) | |
Source | PubChem | |
URL | https://pubchem.ncbi.nlm.nih.gov | |
Description | Data deposited in or computed by PubChem | |
InChI Key |
HWTXXNAQBWZFHL-UHFFFAOYSA-N | |
Source | PubChem | |
URL | https://pubchem.ncbi.nlm.nih.gov | |
Description | Data deposited in or computed by PubChem | |
Canonical SMILES |
C(COCCOCCOCCS)C(=O)O | |
Source | PubChem | |
URL | https://pubchem.ncbi.nlm.nih.gov | |
Description | Data deposited in or computed by PubChem | |
Molecular Formula |
C9H18O5S | |
Source | PubChem | |
URL | https://pubchem.ncbi.nlm.nih.gov | |
Description | Data deposited in or computed by PubChem | |
Molecular Weight |
238.30 g/mol | |
Source | PubChem | |
URL | https://pubchem.ncbi.nlm.nih.gov | |
Description | Data deposited in or computed by PubChem | |
Retrosynthesis Analysis
AI-Powered Synthesis Planning: Our tool employs the Template_relevance Pistachio, Template_relevance Bkms_metabolic, Template_relevance Pistachio_ringbreaker, Template_relevance Reaxys, Template_relevance Reaxys_biocatalysis model, leveraging a vast database of chemical reactions to predict feasible synthetic routes.
One-Step Synthesis Focus: Specifically designed for one-step synthesis, it provides concise and direct routes for your target compounds, streamlining the synthesis process.
Accurate Predictions: Utilizing the extensive PISTACHIO, BKMS_METABOLIC, PISTACHIO_RINGBREAKER, REAXYS, REAXYS_BIOCATALYSIS database, our tool offers high-accuracy predictions, reflecting the latest in chemical research and data.
Strategy Settings
Precursor scoring | Relevance Heuristic |
---|---|
Min. plausibility | 0.01 |
Model | Template_relevance |
Template Set | Pistachio/Bkms_metabolic/Pistachio_ringbreaker/Reaxys/Reaxys_biocatalysis |
Top-N result to add to graph | 6 |
Feasible Synthetic Routes
Disclaimer and Information on In-Vitro Research Products
Please be aware that all articles and product information presented on BenchChem are intended solely for informational purposes. The products available for purchase on BenchChem are specifically designed for in-vitro studies, which are conducted outside of living organisms. In-vitro studies, derived from the Latin term "in glass," involve experiments performed in controlled laboratory settings using cells or tissues. It is important to note that these products are not categorized as medicines or drugs, and they have not received approval from the FDA for the prevention, treatment, or cure of any medical condition, ailment, or disease. We must emphasize that any form of bodily introduction of these products into humans or animals is strictly prohibited by law. It is essential to adhere to these guidelines to ensure compliance with legal and ethical standards in research and experimentation.