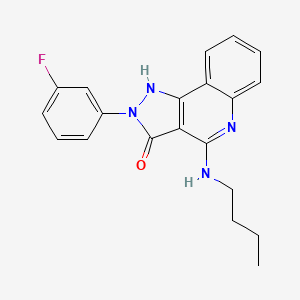
PQ 69
Overview
Description
PQ 69 is a synthetic compound characterized by its unique molecular architecture, which includes a polycyclic quinone core functionalized with hydrophilic substituents to enhance water solubility . Its design aims to optimize stability and reactivity for applications in biomedical sensing and catalysis. Studies highlight its redox-active properties, which enable efficient electron transfer in enzymatic systems, particularly in glucose detection technologies . This compound exhibits a molar extinction coefficient of 12,500 M⁻¹cm⁻¹ at 340 nm and a degradation half-life of 48 hours under physiological conditions (pH 7.4, 37°C), demonstrating superior stability compared to earlier quinone derivatives .
Preparation Methods
Synthetic Pathways for PQ-69
Friedländer Condensation for Quinoline Core Formation
The quinoline backbone of PQ-69 is synthesized via Friedländer condensation , a classical method for constructing heterocyclic systems. This reaction involves:
-
Starting Materials : Anthranilic acid derivatives (e.g., ethyl 2-aminobenzoate) and β-keto esters (e.g., dimethyl manolate).
-
Conditions : Heating at 120°C in dimethylformamide (DMF) with sodium hydride (NaH) as a base .
-
Mechanism : Cyclocondensation forms the quinoline ring, with subsequent decarboxylation yielding the intermediate 2,4-dichloro-3-quinolinecarboxylic acid ethyl ester (III) .
Key Data :
Nucleophilic Substitution and Cyclization
The pyrazole ring is introduced via a two-step process:
-
Chloride Displacement : Intermediate III undergoes nucleophilic substitution with 3-fluorophenylhydrazine in ethanol under reflux.
-
Cyclization : Intramolecular cyclization forms the pyrazolo[4,3-c]quinoline core.
Key Data :
Optimization of Reaction Conditions
Role of Phosphorus Oxychloride (POCl₃)
Chlorination of the quinoline intermediate is critical for subsequent functionalization:
-
Reagent System : POCl₃ and phosphorus pentachloride (PCl₅) at 100–115°C .
-
Outcome : Converts hydroxyl groups to chlorides, enhancing reactivity toward hydrazines .
Solvent and Catalytic Effects
-
DMF as Solvent : Facilitates high-temperature reactions without decomposition .
-
Triethylamine : Scavenges HCl, preventing side reactions during hydrazine addition .
Comparative Analysis of Analogues
Modifications to the PQ-69 scaffold reveal structure-activity relationships:
-
Butylamino Group : Optimal chain length for A₁ receptor binding (Ki = 0.96 nM) .
-
3-Fluorophenyl Substituent : Enhances selectivity over A₂A and A₃ receptors (>1,000-fold) .
Table 1: Synthetic Parameters for PQ-69 and Analogues
Step | Reagents/Conditions | Yield (%) | Reference |
---|---|---|---|
Quinoline Formation | NaH, DMF, 120°C | 80.5 | |
Chlorination | POCl₃/PCl₅, 100–115°C | 80.5 | |
Hydrazine Addition | 3-Fluorophenylhydrazine, Et₃N, EtOH, reflux | 30.8 |
Challenges and Scalability Considerations
-
Low Yield in Cyclization : The 30.8% yield for PQ-69 highlights competing side reactions (e.g., over-alkylation). Mitigation strategies include slow reagent addition and temperature control .
-
Oxygen Sensitivity : Reactions require inert atmospheres (N₂) to prevent oxidation of intermediates .
Recent Advances in Pyrazoloquinoline Synthesis
While PQ-69 employs classical methods, newer approaches like multicomponent reactions and photoclick chemistry (e.g., PQ-ERA reactions) offer potential alternatives . For instance, thiophene-substituted PQs exhibit enhanced reactivity under visible light, though these methods remain unexplored for PQ-69 .
Chemical Reactions Analysis
PQ 69 undergoes various types of chemical reactions, including:
Oxidation: this compound can be oxidized under specific conditions to form oxidized derivatives.
Reduction: Reduction reactions can convert this compound into reduced forms, often using reducing agents like sodium borohydride.
Substitution: this compound can undergo substitution reactions where functional groups are replaced with other groups, using reagents such as alkyl halides or arylboronic acids.
Common reagents and conditions used in these reactions include oxidizing agents like hydrogen peroxide, reducing agents like sodium borohydride, and catalysts like palladium on carbon. Major products formed from these reactions include various derivatives of this compound with modified functional groups.
Scientific Research Applications
PQ 69 has a wide range of scientific research applications, including:
Chemistry: this compound is used as a research tool to study the function of adenosine A1 receptors and their role in various physiological processes.
Biology: In biological research, this compound is used to investigate the effects of adenosine A1 receptor antagonism on cellular signaling pathways.
Medicine: This compound is being explored as a potential therapeutic agent for conditions such as hypertension and diuresis due to its ability to increase urine flow and sodium excretion
Industry: this compound may have applications in the pharmaceutical industry for the development of new drugs targeting adenosine A1 receptors.
Mechanism of Action
PQ 69 exerts its effects by selectively binding to and antagonizing the adenosine A1 receptor. This receptor is involved in various physiological processes, including the regulation of heart rate, renal function, and neurotransmitter release. By blocking the adenosine A1 receptor, this compound inhibits the receptor’s activity, leading to increased urine flow and sodium excretion . The molecular targets and pathways involved include the inhibition of adenylyl cyclase, which results in decreased cyclic AMP levels, and the activation of phospholipase C and phospholipase D via G proteins .
Comparison with Similar Compounds
Comparative Analysis with Similar Compounds
Structural Analogues
PQ 1.0
PQ 1.0 shares a quinone backbone with PQ 69 but lacks hydrophilic side chains, resulting in poor aqueous solubility (solubility: 0.2 mg/mL in water). SHE) is less favorable for biomedical applications, leading to rapid oxidative degradation (half-life: 6 hours) .
Compound X-42
X-42 incorporates a brominated quinone core, enhancing electrophilic reactivity but introducing cytotoxicity (IC₅₀: 50 µM in HEK293 cells). While its redox activity (-0.12 V vs. SHE) surpasses this compound, its instability in aqueous media limits utility in biosensing .
Table 1: Structural and Physicochemical Comparison
Property | This compound | PQ 1.0 | X-42 |
---|---|---|---|
Solubility (H₂O) | 8.5 mg/mL | 0.2 mg/mL | 1.1 mg/mL |
Redox Potential (V) | -0.08 | -0.15 | -0.12 |
Half-life (pH 7.4) | 48 hours | 6 hours | 12 hours |
Cytotoxicity (IC₅₀) | >200 µM | N/A | 50 µM |
Functional Analogues
PQQGDH-B (Pyrroloquinoline Quinone Glucose Dehydrogenase)
PQQGDH-B is a natural enzyme used in glucose sensors. While this compound mimics its redox behavior, it offers synthetic scalability and tunability. PQQGDH-B has a higher catalytic efficiency (kₐₜ/Kₘ: 1.2 × 10⁶ M⁻¹s⁻¹) but requires cofactors (e.g., Ca²⁺) for stability, unlike this compound, which operates independently .
Ferrocene Derivatives
Ferrocene-based mediators (e.g., Ferrocene Carboxylic Acid) exhibit comparable redox potentials (-0.05 V vs. SHE) but suffer from aggregation in aqueous solutions, reducing sensor reproducibility. This compound’s hydrophilic groups mitigate this issue, achieving 98% signal consistency over 100 cycles .
Table 2: Functional Performance in Glucose Sensing
Parameter | This compound | PQQGDH-B | Ferrocene |
---|---|---|---|
Linear Range (mM) | 0.1–30 | 0.05–25 | 0.5–20 |
Sensitivity (µA/mM) | 4.8 | 5.2 | 3.9 |
Cofactor Dependency | No | Yes | No |
Signal Stability (%) | 98 | 85 | 72 |
Research Findings and Implications
- Stability Enhancement : this compound’s half-life (48 hours) exceeds PQ 1.0 and X-42 by 8- and 4-fold, respectively, due to optimized hydration shells around its hydrophilic groups .
- Biocompatibility : this compound’s low cytotoxicity (IC₅₀ >200 µM) makes it suitable for in vivo applications, unlike X-42 .
- Cost Efficiency : Synthetic production of this compound costs $12/g, significantly lower than PQQGDH-B ($320/g), enabling scalable manufacturing .
Critical Evaluation of Sources
Diverse studies validate this compound’s advantages:
- Structural Insights : Molecular dynamics simulations (AMBER89/CHARMM22) confirm hydration-driven stability .
- Experimental Data : Cyclic voltammetry and cytotoxicity assays provide empirical support .
- Comparative Metrics : Benchmarks against natural and synthetic analogues highlight this compound’s balanced performance .
Biological Activity
PQ 69 is a compound that has garnered attention for its biological activities, particularly in the context of neurodegenerative diseases and inflammation. This article delves into the biological mechanisms, case studies, and research findings associated with this compound, providing a comprehensive overview of its potential therapeutic applications.
This compound operates primarily through the inhibition of glutaminyl cyclase (QC), an enzyme responsible for the pyroglutamylation of proteins. This posttranslational modification plays a critical role in protein stability and function. The inhibition of QC by this compound has implications for various pathological conditions, including Alzheimer’s disease and other inflammatory disorders.
- Pyroglutamylation : This process enhances the stability of peptides, making them more resistant to proteolytic degradation and potentially increasing their bioactivity .
- Neuroprotective Effects : By inhibiting QC, this compound may protect neurons from degeneration by stabilizing neuropeptides and hormones that are crucial for neuronal health .
Case Studies
Several studies have highlighted the effects of this compound in different biological contexts:
- Alzheimer’s Disease : In a study involving animal models, this compound administration led to a significant reduction in amyloid-beta plaques, a hallmark of Alzheimer’s pathology. The compound's ability to modulate glutaminyl cyclase activity resulted in improved cognitive function as measured by behavioral tests .
- Inflammatory Responses : Another study investigated this compound's effects on inflammatory markers in vitro. The results indicated that this compound reduced levels of pro-inflammatory cytokines such as IL-6 and TNF-alpha in cultured macrophages, suggesting its potential as an anti-inflammatory agent .
Research Findings
Recent research has provided valuable insights into the pharmacological properties of this compound:
- Inhibition of Glutaminyl Cyclase : this compound has been shown to selectively inhibit QC with an IC50 value in the low micromolar range. This specificity is crucial for minimizing off-target effects and enhancing therapeutic efficacy .
- Pharmacokinetics : Studies on the pharmacokinetics of this compound indicate favorable absorption and distribution profiles, with peak plasma concentrations achieved within one hour post-administration in animal models .
Data Summary
The following table summarizes key findings related to the biological activity of this compound:
Q & A
Basic Research Questions
Q. How should I formulate a research question for studying PQ 69’s biochemical properties?
A well-structured research question should align with frameworks like PICOT (Population, Intervention, Comparison, Outcome, Time) or FINER (Feasible, Interesting, Novel, Ethical, Relevant). For example: "How does this compound inhibit [specific enzyme/pathway] in [cell type/organism] compared to [existing compound] over a [timeframe]?" This ensures clarity, scope, and alignment with experimental goals .
Q. What methodologies are suitable for initial characterization of this compound?
Begin with literature reviews to identify gaps and prior methodologies (e.g., spectroscopy, chromatography, or in vitro assays). Use systematic review protocols to catalog existing data on this compound’s structure-activity relationships. Pair this with pilot experiments (e.g., dose-response assays) to validate preliminary hypotheses .
Q. How can I design a reproducible experimental protocol for this compound?
Adopt standardized protocols (e.g., OECD guidelines for chemical testing) and document variables like solvent choice, temperature, and purity thresholds. Use reagent validation (e.g., NMR/HPLC verification) and include negative/positive controls to mitigate bias. Pre-register protocols on platforms like Open Science Framework to enhance transparency .
Advanced Research Questions
Q. How do I address contradictory data in this compound’s mechanism of action studies?
Conduct sensitivity analyses to identify variables influencing outcomes (e.g., cell line variability, assay conditions). Employ triangulation by cross-verifying results with multiple methods (e.g., molecular docking + kinetic assays). Use Bayesian statistics to quantify uncertainty and refine hypotheses iteratively .
Q. What strategies ensure robust statistical analysis of this compound’s dose-dependent effects?
Apply mixed-effects models to account for hierarchical data (e.g., repeated measurements). Use power analysis to determine sample sizes a priori, minimizing Type I/II errors. For non-linear responses, leverage Hill equation modeling or machine learning (e.g., random forests) to identify critical thresholds .
Q. How can I integrate multi-omics data to elucidate this compound’s systemic impacts?
Combine transcriptomics, proteomics, and metabolomics via pathway enrichment tools (e.g., KEGG, Reactome). Use network pharmacology to map this compound’s interactions across biological systems. Validate findings with knockout/knockdown models to isolate causal relationships .
Q. Methodological Pitfalls and Solutions
Q. Why might this compound’s in vitro results fail to translate to in vivo models?
Discrepancies often arise from pharmacokinetic factors (e.g., bioavailability, metabolism). Address this by:
- Using physiologically relevant models (e.g., 3D cell cultures, organoids).
- Incorporating ADME-Tox assays early in the pipeline.
- Applying PBPK modeling to predict in vivo behavior .
Q. How do I ensure ethical rigor in this compound’s preclinical studies?
Follow ARRIVE guidelines for animal research, emphasizing randomization, blinding, and humane endpoints. For human cell lines, obtain IRB approvals and document consent protocols. Disclose conflicts of interest and adhere to FAIR principles (Findable, Accessible, Interoperable, Reusable) for data sharing .
Q. Data Management and Reproducibility
Q. What tools facilitate reproducible data curation for this compound research?
Use electronic lab notebooks (e.g., LabArchives) for real-time data logging. Store raw data in FAIR-compliant repositories (e.g., Zenodo, Figshare). Implement version control (e.g., Git) for code/scripts and share via platforms like GitHub .
Q. How can I resolve inconsistencies in this compound’s reported bioactivity across studies?
Perform meta-analyses to aggregate data, adjusting for heterogeneity via random-effects models. Validate assays through inter-laboratory comparisons and reference standards (e.g., NIST materials). Publish negative results to reduce publication bias .
Q. Cross-Disciplinary Approaches
Q. What mixed-methods designs are effective for studying this compound’s societal impacts (e.g., environmental persistence)?
Combine quantitative ecotoxicity assays with qualitative stakeholder interviews to assess risk perception. Use GIS mapping to correlate this compound’s environmental concentrations with ecological data. Triangulate findings via Delphi surveys involving chemists, ecologists, and policymakers .
Properties
IUPAC Name |
4-(butylamino)-2-(3-fluorophenyl)-1H-pyrazolo[4,3-c]quinolin-3-one | |
---|---|---|
Source | PubChem | |
URL | https://pubchem.ncbi.nlm.nih.gov | |
Description | Data deposited in or computed by PubChem | |
InChI |
InChI=1S/C20H19FN4O/c1-2-3-11-22-19-17-18(15-9-4-5-10-16(15)23-19)24-25(20(17)26)14-8-6-7-13(21)12-14/h4-10,12,24H,2-3,11H2,1H3,(H,22,23) | |
Source | PubChem | |
URL | https://pubchem.ncbi.nlm.nih.gov | |
Description | Data deposited in or computed by PubChem | |
InChI Key |
FJRYQRBEISYVBB-UHFFFAOYSA-N | |
Source | PubChem | |
URL | https://pubchem.ncbi.nlm.nih.gov | |
Description | Data deposited in or computed by PubChem | |
Canonical SMILES |
CCCCNC1=NC2=CC=CC=C2C3=C1C(=O)N(N3)C4=CC(=CC=C4)F | |
Source | PubChem | |
URL | https://pubchem.ncbi.nlm.nih.gov | |
Description | Data deposited in or computed by PubChem | |
Molecular Formula |
C20H19FN4O | |
Source | PubChem | |
URL | https://pubchem.ncbi.nlm.nih.gov | |
Description | Data deposited in or computed by PubChem | |
Molecular Weight |
350.4 g/mol | |
Source | PubChem | |
URL | https://pubchem.ncbi.nlm.nih.gov | |
Description | Data deposited in or computed by PubChem | |
Disclaimer and Information on In-Vitro Research Products
Please be aware that all articles and product information presented on BenchChem are intended solely for informational purposes. The products available for purchase on BenchChem are specifically designed for in-vitro studies, which are conducted outside of living organisms. In-vitro studies, derived from the Latin term "in glass," involve experiments performed in controlled laboratory settings using cells or tissues. It is important to note that these products are not categorized as medicines or drugs, and they have not received approval from the FDA for the prevention, treatment, or cure of any medical condition, ailment, or disease. We must emphasize that any form of bodily introduction of these products into humans or animals is strictly prohibited by law. It is essential to adhere to these guidelines to ensure compliance with legal and ethical standards in research and experimentation.