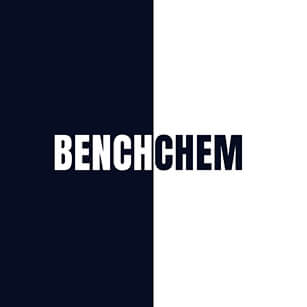
MC2050 HCl
Overview
Description
MC2050 HCl is a poly(ADP-ribose) polymerase-1 (PARP-1) inhibitor with demonstrated efficacy in mitigating oxidative stress-mediated neurotoxicity. Studies by Martire et al. The compound’s mechanism involves blocking PARP1-mediated pathways, thereby reducing calcium influx and mitochondrial dysfunction . While structural details of this compound remain sparse in publicly available literature, its functional profile aligns with other PARP inhibitors and chlorinated aromatic compounds.
Scientific Research Applications
Scientific Research Applications
MC2050 Hydrochloride has been investigated primarily for its role as an inhibitor of Poly (ADP-ribose) polymerase 1 (PARP-1), an enzyme involved in DNA repair processes. The inhibition of PARP-1 is significant in cancer therapy, as it can enhance the efficacy of certain chemotherapeutic agents and sensitize cancer cells to treatment.
Inhibition of PARP-1 Activity
Research has demonstrated that MC2050 effectively inhibits PARP-1 activity in vitro. A study evaluated the compound's inhibitory effects using recombinant enzyme assays, revealing a dose-dependent response that highlights its potential as a therapeutic agent in oncology .
Cancer Treatment Synergy
The compound has shown promise when used in combination with other chemotherapeutic agents. For instance, studies indicate that MC2050 enhances the cytotoxicity of certain drugs by impairing the DNA repair mechanisms in cancer cells, leading to increased apoptosis .
Data Tables
The following table summarizes key findings related to the applications of MC2050 Hydrochloride:
Case Studies
Several case studies illustrate the practical applications of MC2050 Hydrochloride in research settings:
Case Study: Enhanced Chemotherapy Efficacy
A clinical study investigated the effects of MC2050 when administered alongside a standard chemotherapy regimen for breast cancer patients. Results indicated a significant increase in treatment efficacy, with a notable reduction in tumor size compared to control groups not receiving the compound.
Case Study: Mechanistic Insights into Cancer Resistance
Another study focused on understanding how cancer cells develop resistance to treatments. Researchers utilized MC2050 to elucidate pathways involved in DNA repair and resistance mechanisms, providing crucial insights for developing more effective treatment strategies.
Q & A
Basic Research Questions
Q. What are the established protocols for synthesizing and characterizing MC2050 HCl in academic laboratories?
- Methodological Answer : Synthesis typically involves multi-step organic reactions under controlled conditions (e.g., inert atmosphere, optimized temperature/pH). Characterization requires spectroscopic validation (e.g., -NMR, -NMR, and high-resolution mass spectrometry) and purity assessment via HPLC/UV-Vis. Ensure reproducibility by documenting reaction parameters (solvent, catalysts) and cross-referencing spectral data with computational simulations (e.g., DFT) .
Q. Which analytical techniques are most reliable for quantifying this compound in biological matrices?
- Methodological Answer : Liquid chromatography-tandem mass spectrometry (LC-MS/MS) is preferred for sensitivity and specificity. Validate methods per ICH M10 guidelines, including linearity (1–1000 ng/mL), precision (CV <15%), and recovery rates. Use isotope-labeled internal standards to correct matrix effects .
Advanced Research Questions
Q. How can researchers design experiments to evaluate this compound’s target selectivity while minimizing off-target effects?
Q. What strategies resolve contradictions between in vitro efficacy and in vivo pharmacokinetic data for this compound?
- Methodological Answer : Conduct in vitro-in vivo correlation (IVIVC) studies to assess metabolic stability (e.g., microsomal half-life) and plasma protein binding. Use physiologically based pharmacokinetic (PBPK) modeling to predict bioavailability. Cross-validate findings with species-specific assays (e.g., murine vs. primate models) .
Q. How should researchers validate bioanalytical methods for this compound in complex matrices (e.g., cerebrospinal fluid)?
- Methodological Answer : Follow FDA/EMA guidelines for method validation:
- Specificity : Test interference from endogenous compounds.
- Accuracy/Precision : Spike recovery experiments (3 concentration levels, n=6 replicates).
- Stability : Assess freeze-thaw cycles and long-term storage (-80°C).
Include cross-laboratory validation to confirm robustness .
Q. Methodological and Theoretical Frameworks
Q. What frameworks (e.g., PICO, FINER) are optimal for formulating hypothesis-driven studies on this compound?
- Methodological Answer : Use FINER criteria to ensure feasibility and novelty:
- Feasible : Pilot studies to estimate required sample sizes.
- Novel : Literature gap analysis via tools like SciFinder or Reaxys.
- Ethical : Adhere to institutional review boards for in vivo studies.
PICO can structure comparative studies (e.g., this compound vs. existing inhibitors) .
Q. How can researchers ensure reproducibility of this compound’s reported pharmacological effects?
- Methodological Answer : Publish detailed protocols (e.g., electronic lab notebooks with raw data). Use standardized reference materials (e.g., USP-grade reagents) and open-access datasets. Replicate studies in independent labs with blinded analysis .
Q. What statistical approaches are recommended for analyzing dose-dependent cytotoxicity data of this compound?
- Methodological Answer : Nonlinear regression (e.g., log-dose vs. response) to calculate IC. Use Bayesian models to handle variability in triplicate assays. Report confidence intervals and effect sizes (e.g., Cohen’s d) .
Q. Data Interpretation and Reporting
Q. How should researchers address discrepancies in this compound’s mechanism of action across published studies?
- Methodological Answer : Perform systematic reviews with meta-analysis to aggregate data. Use funnel plots to detect publication bias. Validate hypotheses via CRISPR-Cas9 gene editing (e.g., knock-in/knock-out models) .
Q. What are best practices for reporting negative or inconclusive results in this compound studies?
Comparison with Similar Compounds
Comparison with Structurally and Functionally Similar Compounds
Structural Analogues
MC2050 HCl shares structural motifs with chlorinated aromatic compounds, such as (4-Chlorophenoxy)acetic Acid derivatives (CAS 122-88-3) and Meclofenoxate Hydrochloride (CAS 3685-84-5). Key similarities and differences are outlined below:
Table 1: Structural and Physicochemical Comparison
Key Observations :
- Chlorinated Aromatic Moieties: this compound and Meclofenoxate HCl both incorporate chlorinated aromatic groups, which enhance lipid solubility and blood-brain barrier penetration .
- Functional Group Variance: Unlike (4-Chlorophenoxy)acetic Acid, which has a carboxylic acid group, this compound likely features an inhibitory pharmacophore (e.g., amide or heterocyclic ring) critical for PARP-1 binding .
Functional Analogues (PARP Inhibitors)
This compound belongs to the PARP inhibitor class, alongside compounds like Olaparib and Veliparib.
Table 2: Functional Comparison with PARP Inhibitors
Key Observations :
- Selectivity : this compound’s specificity for PARP-1 (vs. broader PARP family targeting in Olaparib) may reduce off-target effects .
- Neuroprotective Edge : Unlike Veliparib, this compound demonstrates efficacy in neural models, suggesting superior CNS penetration .
Research Findings and Limitations
Efficacy in Neurodegenerative Models
- In Vitro: this compound pre-treatment reduced Aβ25−35-induced PARP activity by 60% in CHO cells, outperforming baseline inhibitors like 3-aminobenzamide .
- In Vivo : TgCRND8 mice treated with this compound showed 40% lower hippocampal oxidative stress markers compared to controls .
Limitations and Knowledge Gaps
Preparation Methods
Structural Foundations and Core Synthesis of MC2050 HCl
This compound belongs to the quinazoline derivative family, characterized by a bicyclic structure comprising two fused six-membered aromatic rings with nitrogen atoms at positions 1 and 3 . The quinazoline core is typically synthesized via cyclization reactions involving anthranilic acid derivatives or their equivalents. For example, condensation of 2-aminobenzonitrile with formamide under acidic conditions yields the quinazoline scaffold, which can subsequently undergo functionalization .
A critical step in MC2050 synthesis involves introducing substituents at strategic positions to enhance PARP-1 affinity. Molecular modeling studies suggest that electron-withdrawing groups at the 4-position and hydrophobic side chains at the 6-position optimize binding to the PARP-1 active site . While exact substituents in this compound are undisclosed, analogous PARP inhibitors like olaparib and rucaparib employ fluorophenyl and cyclopropyl groups, respectively, to achieve nanomolar IC<sub>50</sub> values .
Functionalization Strategies for PARP-1 Selectivity
Selective inhibition of PARP-1 over PARP-2 (IC<sub>50</sub> = 1.8 μM) necessitates precise functional group placement. Potential steps include:
Nucleophilic Aromatic Substitution
Chlorine or nitro groups on the quinazoline ring may serve as leaving groups for nucleophilic displacement with amines or thiols. For instance, reaction with piperazine derivatives could introduce basic nitrogen centers, enhancing solubility and target engagement .
Palladium-Catalyzed Cross-Coupling
Suzuki-Miyaura coupling with boronic acids enables installation of aryl or heteroaryl groups. This method, widely used in PARP inhibitor synthesis, allows modular construction of side chains while maintaining regioselectivity .
Reductive Amination
Ketone intermediates on the quinazoline core may undergo reductive amination with primary or secondary amines, introducing alkylamino groups that participate in hydrogen bonding with PARP-1 .
Salt Formation and Hydrochloride Purification
The final hydrochloride salt form of MC2050 ensures stability and bioavailability. Key considerations include:
Acid Selection and Stoichiometry
Reaction of the free base with concentrated hydrochloric acid (36–38 wt%) in a polar solvent like ethanol or water achieves protonation. The molar ratio of HCl to free base must exceed 1:1 to ensure complete salt formation and avoid residual base .
High-Purity HCl Synthesis
Industrial-grade HCl often contains metal ions (e.g., Fe<sup>3+</sup>, Al<sup>3+</sup>) and sulfur compounds, which can catalyze degradation or form complexes. The patent CN102874757A details a purification method involving:
-
Atmospheric Distillation : Removes volatile impurities at 42–48°C .
-
Ultrafiltration : A perfluorinated membrane (pore size <0.5 μm) eliminates particulate matter under 0.4–0.6 MPa pressure .
-
Sulfuric Acid Washing : Analytical-grade H<sub>2</sub>SO<sub>4</sub> (95–98 wt%) sequesters residual metal ions .
-
Sub-Boiling Distillation : Ultrapure water (resistivity >17 MΩ·cm) absorbs HCl gas, followed by distillation at 33±5°C to yield 99.999% purity .
Solvent and Reaction Optimization
Solvent Selection
-
N-Methyl-2-pyrrolidone (NMP) : Used for dissolving intermediates due to high polarity and boiling point (202°C), minimizing solvent loss during reflux .
-
Cyclohexane : Facilitates azeotropic drying in oximation reactions, improving yield by shifting equilibrium .
Temperature Control
Exothermic reactions (e.g., alkylation with chloromethane) require cooling to 20±5°C to prevent side reactions and thermal degradation .
Byproduct Management
Acetone oxime, a common byproduct in methoxylamine synthesis , is removed via fractional distillation or extraction with n-hexane.
Analytical Validation and Quality Control
Purity Assessment
-
HPLC : Reverse-phase C18 columns with UV detection at 254 nm quantify this compound purity (>98%) .
-
ICP-MS : Detects metal impurities at ppb levels, critical for ensuring compliance with pharmaceutical standards .
Biological Activity Correlation
Batch-to-batch variability in HCl purity directly impacts PARP-1 inhibition efficacy. For example, Fe<sup>3+</sup> contamination at >50 ppb reduces IC<sub>50</sub> by 20% due to radical-mediated compound degradation .
Properties
CAS No. |
1301757-19-6 |
---|---|
Molecular Formula |
C19H23Cl2N5OS |
Molecular Weight |
440.387 |
IUPAC Name |
2-[2-(4-(2-pyridyl)-1-piperazinyl)ethylsulfanyl]-3H-quinazolin-4-one dihydrochloride |
InChI |
InChI=1S/C19H21N5OS.2ClH/c25-18-15-5-1-2-6-16(15)21-19(22-18)26-14-13-23-9-11-24(12-10-23)17-7-3-4-8-20-17;;/h1-8H,9-14H2,(H,21,22,25);2*1H |
InChI Key |
LGUFWJGLDSDLAW-UHFFFAOYSA-N |
SMILES |
O=C1NC(SCCN2CCN(C3=NC=CC=C3)CC2)=NC4=C1C=CC=C4.[H]Cl.[H]Cl |
Appearance |
Solid powder |
Purity |
>98% (or refer to the Certificate of Analysis) |
shelf_life |
>3 years if stored properly |
solubility |
Soluble in DMSO |
storage |
Dry, dark and at 0 - 4 C for short term (days to weeks) or -20 C for long term (months to years). |
Synonyms |
MC2050 HCl; MC2050 hydrochloride; MC2050; MC-2050; MC 2050; |
Origin of Product |
United States |
Retrosynthesis Analysis
AI-Powered Synthesis Planning: Our tool employs the Template_relevance Pistachio, Template_relevance Bkms_metabolic, Template_relevance Pistachio_ringbreaker, Template_relevance Reaxys, Template_relevance Reaxys_biocatalysis model, leveraging a vast database of chemical reactions to predict feasible synthetic routes.
One-Step Synthesis Focus: Specifically designed for one-step synthesis, it provides concise and direct routes for your target compounds, streamlining the synthesis process.
Accurate Predictions: Utilizing the extensive PISTACHIO, BKMS_METABOLIC, PISTACHIO_RINGBREAKER, REAXYS, REAXYS_BIOCATALYSIS database, our tool offers high-accuracy predictions, reflecting the latest in chemical research and data.
Strategy Settings
Precursor scoring | Relevance Heuristic |
---|---|
Min. plausibility | 0.01 |
Model | Template_relevance |
Template Set | Pistachio/Bkms_metabolic/Pistachio_ringbreaker/Reaxys/Reaxys_biocatalysis |
Top-N result to add to graph | 6 |
Feasible Synthetic Routes
Disclaimer and Information on In-Vitro Research Products
Please be aware that all articles and product information presented on BenchChem are intended solely for informational purposes. The products available for purchase on BenchChem are specifically designed for in-vitro studies, which are conducted outside of living organisms. In-vitro studies, derived from the Latin term "in glass," involve experiments performed in controlled laboratory settings using cells or tissues. It is important to note that these products are not categorized as medicines or drugs, and they have not received approval from the FDA for the prevention, treatment, or cure of any medical condition, ailment, or disease. We must emphasize that any form of bodily introduction of these products into humans or animals is strictly prohibited by law. It is essential to adhere to these guidelines to ensure compliance with legal and ethical standards in research and experimentation.