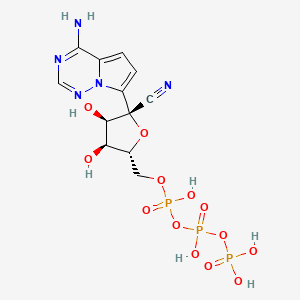
Remdesivir triphosphate
Overview
Description
Remdesivir triphosphate (RDV-TP) is the pharmacologically active metabolite of the prodrug remdesivir (GS-5734), a broad-spectrum antiviral agent. Remdesivir undergoes intracellular phosphorylation to form RDV-TP, which mimics the structure of adenosine triphosphate (ATP) but contains a 1′-cyano modification . This structural similarity allows RDV-TP to compete with ATP for incorporation into viral RNA by the RNA-dependent RNA polymerase (RdRp) of SARS-CoV-2, SARS-CoV, and MERS-CoV . Once incorporated, RDV-TP induces delayed chain termination during RNA synthesis, halting viral replication at the "i+3" position . RDV-TP exhibits high selectivity for viral RdRp over human polymerases, minimizing host toxicity . Clinical studies have demonstrated its efficacy in reducing recovery time in severe COVID-19 patients, leading to regulatory approvals by agencies such as the EMA and FDA .
Preparation Methods
Synthetic Routes and Reaction Conditions
Remdesivir triphosphate is synthesized from remdesivir through a series of phosphorylation reactions. The process involves the conversion of remdesivir to its monophosphate form, followed by further phosphorylation to yield the diphosphate and finally the triphosphate form .
Industrial Production Methods
The industrial production of this compound typically involves large-scale chemical synthesis using high-purity reagents and stringent reaction conditions to ensure the desired yield and purity. The process is optimized for scalability and cost-effectiveness, often employing automated systems for precise control of reaction parameters .
Chemical Reactions Analysis
Mechanism of Action of Remdesivir Triphosphate
- RTP, as an adenosine nucleoside triphosphate analog (GS-443902), interferes with viral RNA-dependent RNA polymerase .
- RTP evades proofreading by viral exoribonuclease (ExoN), causing a decrease in viral RNA production .
- RTP can cause the RNA-dependent RNA polymerases to pause or induce an irreversible chain termination .
- For the RNA-dependent RNA polymerases of MERS-CoV, SARS-CoV-1, and SARS-CoV-2, arrest of RNA synthesis occurs after incorporation of three additional nucleotides . Thus, remdesivir is classified as a direct-acting antiviral agent that works as a delayed chain terminator .
Binding of this compound
- Remdesivir monophosphate (RMP) is selected over adenosine monophosphate (AMP) by the RNA-dependent RNA polymerase nsp12 for nucleotide incorporation .
- Remdesivir has a cyano substitution at the C1’ position of the ribosyl moiety and a modified base structure to stabilize the linkage of the base to the C1’ atom with its strong electron-withdrawing cyano group .
- The equilibrium conformation of RTP differs from the binding mode of ATP in that it is missing an intra-molecular hydrogen bond (HB) between its O3’ and the β-phosphate group .
- The triphosphate groups of RTP exhibit multiple conformations, so their MD-ED features are only approximately defined when compared to the ATP complex .
- Ordered water molecules occupy the pocket where the substituted cyano-group of RTP occupies it in the RTP complex .
Interactions of Remdesivir
- Remdesivir is at least partially metabolized by the cytochrome P450 enzymes CYP2C8, CYP2D6, and CYP3A4 .
- Blood plasma concentrations of remdesivir are expected to decrease if it is administered together with cytochrome P450 inducers such as rifampicin, carbamazepine, phenobarbital, phenytoin, primidone, and St John's wort .
- Using chloroquine or hydroxychloroquine with remdesivir may reduce the antiviral activity of remdesivir . Coadministration of remdesivir and chloroquine phosphate or hydroxychloroquine sulfate is not recommended based on in vitro data demonstrating an antagonistic effect of chloroquine on the intracellular metabolic activation and antiviral activity of remdesivir .
Scientific Research Applications
COVID-19 Treatment
Remdesivir was one of the first antiviral treatments authorized for emergency use against COVID-19. Clinical trials have shown that it can reduce recovery time in hospitalized patients with severe COVID-19 symptoms. The efficacy is attributed to the rapid conversion of remdesivir into its triphosphate form within human cells, which then inhibits SARS-CoV-2 replication .
Key Findings from Clinical Studies :
- A study involving nonhuman primates demonstrated that remdesivir provided both prophylactic and therapeutic benefits against MERS-CoV .
- In vitro studies indicated that remdesivir triphosphate effectively inhibited RdRp from SARS-CoV-2, showcasing potent antiviral activity .
Broader Antiviral Potential
Research has indicated that this compound may have applications beyond COVID-19:
- Ebola Virus : Remdesivir has shown efficacy against Ebola in animal models, providing a potential treatment option for future outbreaks .
- Flavivirus Family : Studies have demonstrated that remdesivir can inhibit RdRps from flaviviruses such as Zika and Dengue, suggesting its potential as a broad-spectrum antiviral agent .
Case Study 1: Efficacy Against Ebola Virus
In a clinical trial conducted during the Ebola outbreak in West Africa, remdesivir was administered to patients with confirmed Ebola virus disease. Results indicated a reduction in viral load and improved survival rates among treated individuals compared to historical controls .
Case Study 2: Treatment of Severe COVID-19
In a multi-center clinical trial involving hospitalized patients with severe COVID-19 pneumonia, administration of remdesivir led to a statistically significant reduction in recovery time compared to placebo. The study highlighted the importance of early intervention with remdesivir to improve patient outcomes .
Mechanism of Action
Remdesivir triphosphate exerts its antiviral effects by incorporating into the viral RNA strand during replication. Once incorporated, it causes delayed chain termination, preventing the completion of the RNA strand and thereby inhibiting viral replication. The compound targets the viral RNA-dependent RNA polymerase, binding to its active site and competing with natural nucleotides .
Comparison with Similar Compounds
Structural and Mechanistic Comparisons
The table below summarizes key differences between RDV-TP and structurally/functionally analogous compounds:
Key Findings
Efficacy and Selectivity :
- RDV-TP incorporates into viral RNA 4x more efficiently than ATP in SARS-CoV-2 RdRp . Its delayed termination mechanism is concentration-dependent, with greater inhibition at low NTP levels .
- Human mitochondrial RNA polymerase discriminates against RDV-TP with a selectivity ratio of ~500:1 (ATP vs. RDV-TP), reducing off-target effects .
In Vitro vs. Cell Culture Activity :
- Despite an in vitro IC₅₀ of 2.01 mM against SARS-CoV-2 RdRp, RDV-TP shows potent cellular activity (IC₅₀ = 0.77 μM) due to efficient intracellular accumulation .
Comparison with Enisamium’s VR17-04 :
- VR17-04, a metabolite of enisamium, inhibits SARS-CoV-2 RdRp with an IC₅₀ of 2–3 mM, comparable to RDV-TP . However, its clinical efficacy remains unproven.
Molecular Interactions
- Binding to RdRp : RDV-TP forms hydrogen bonds with Asp760 and Asp761 in SARS-CoV-2 RdRp, stabilizing its incorporation into RNA . Molecular docking studies confirm its binding pose aligns with crystallographic data (PDB: 7BV2) .
- Allosteric Effects: RDV-TP may allosterically activate RdRp at non-catalytic sites, enhancing template-dependent inhibition .
Clinical and Regulatory Status
- RDV-TP’s parent drug, remdesivir, is approved for COVID-19 in adults and children (>12 years) with severe disease .
Biological Activity
Remdesivir, originally developed as a treatment for Ebola virus, has gained significant attention as an antiviral agent against SARS-CoV-2, the virus responsible for COVID-19. The active metabolite of remdesivir, remdesivir triphosphate (RTP), plays a crucial role in its antiviral efficacy. This article explores the biological activity of this compound, focusing on its mechanism of action, pharmacological properties, and clinical implications.
Inhibition of RNA-dependent RNA Polymerase (RdRp)
this compound acts primarily by inhibiting the RNA-dependent RNA polymerase (RdRp) of various RNA viruses, including coronaviruses and flaviviruses. Upon entering infected cells, remdesivir is metabolized into RTP, which competes with adenosine triphosphate (ATP) for incorporation into the viral RNA. This incorporation leads to premature termination of RNA synthesis due to the unique structure of RTP, which allows the addition of only three nucleotides before stalling occurs at the fourth position .
Structural Insights
Recent studies utilizing molecular dynamics simulations have provided insights into how RTP selectively binds to RdRp. RTP's structure allows it to mimic ATP while also creating a barrier that prevents further elongation of the RNA chain once incorporated . This mechanism is critical for its antiviral activity against a range of viruses, including SARS-CoV-2 and others from the Filoviridae and Flaviviridae families .
Pharmacokinetics and Bioavailability
Metabolism and Distribution
After intravenous administration, remdesivir is rapidly converted to its active triphosphate form within cells. Studies have shown that RTP has a half-life of less than 8 hours in primary human lung cells, which is significantly shorter than other antiviral agents like AT-9010 . The rapid metabolism underscores the need for effective dosing regimens to maintain therapeutic levels during treatment.
Tissue Concentrations
Pharmacokinetic studies indicate that remdesivir and its metabolites distribute widely across various tissues. After administration in animal models, significant concentrations of RTP were detected in the lungs, liver, and kidneys, which are critical sites for viral replication .
Clinical Efficacy
Case Studies and Clinical Trials
Clinical trials have demonstrated that remdesivir significantly reduces recovery time in hospitalized COVID-19 patients. In one randomized controlled trial, patients treated with remdesivir recovered in an average of 11 days compared to 15 days for those receiving a placebo . Another study highlighted that remdesivir treatment led to improved clinical outcomes in patients requiring mechanical ventilation or with severe pneumonia .
Study | Population | Treatment | Outcome |
---|---|---|---|
Study 1 | Hospitalized COVID-19 patients | Remdesivir (200 mg day 1; 100 mg for 9 days) | Recovery time reduced from 15 to 11 days |
Study 2 | Patients with severe COVID-19 | Remdesivir vs standard care | Lower mortality and improved recovery rates |
Study 3 | Pediatric patients with leukemia | Remdesivir (5 mg/kg day 1; 2.5 mg/kg daily) | Patient became SARS-CoV-2 negative |
Safety Profile
While remdesivir has been associated with some adverse effects, including liver enzyme elevations and gastrointestinal symptoms, overall safety profiles from clinical trials indicate it is well-tolerated in most patients . Notably, no significant mitochondrial toxicity was observed despite concerns about RTP's interaction with mitochondrial DNA polymerase .
Q & A
Basic Research Questions
Q. What is the mechanism by which remdesivir triphosphate (RDV-TP) inhibits SARS-CoV-2 replication?
RDV-TP inhibits the viral RNA-dependent RNA polymerase (RdRp) by acting as an adenosine triphosphate (ATP) analog. It competes with ATP for incorporation into the nascent RNA strand, leading to delayed chain termination at position i+3 post-incorporation . This termination disrupts viral genome replication. Structural studies (e.g., cryo-EM of RdRp complexes) confirm RDV-TP binds to the active site of SARS-CoV-2 RdRp (nsp12-nsp7-nsp8 complex), mimicking ATP while evading proofreading by viral exonucleases .
Q. How is remdesivir metabolized to its pharmacologically active triphosphate form?
Remdesivir undergoes intracellular metabolism:
- Step 1 : Hydrolysis by esterases (e.g., carboxylesterase 1) to form an alanine metabolite.
- Step 2 : Phosphorylation by cellular kinases (e.g., adenylate kinase) to produce remdesivir monophosphate (RMP).
- Step 3 : Sequential phosphorylation by phosphoglycerate kinase and creatine kinase to generate RDV-TP . Enzyme-specific assays (e.g., LC-MS quantification of metabolites in peripheral blood mononuclear cells) validate this pathway .
Advanced Research Questions
Q. What experimental methodologies are used to study RDV-TP’s inhibition kinetics against viral RdRp?
Key techniques include:
- Enzyme kinetics assays : Measuring incorporation efficiency (e.g., kcat/Km ratios) using purified RdRp complexes (nsp12-nsp7-nsp8) and radiolabeled nucleotides. RDV-TP shows 3.65-fold selectivity over ATP .
- Cryo-EM and X-ray crystallography : Resolving RdRp-RDV-TP binding at near-atomic resolution (e.g., PDB 7BV2) to identify critical interactions (e.g., hydrogen bonds with Asp760/Asp761) .
- Capillary electrophoresis : Quantifying RNA elongation products to confirm delayed termination at position i+3 .
Q. How do researchers resolve contradictions in RDV-TP’s dual inhibition of RdRp and guanylyltransferase (GTase) activity?
RDV-TP inhibits both RdRp and the NiRAN domain’s GTase activity (critical for viral RNA capping). Dose-response assays (e.g., gel-based quantification of RNA/GTase products) show IC50 values of 6.2 µM (RdRp) vs. 12.4 µM (GTase) . Structural modeling suggests RDV-TP sterically blocks GTP binding in the GTase active site, independent of RdRp inhibition. Researchers prioritize RdRp inhibition as the primary mechanism due to lower IC50 and clinical relevance .
Q. What kinetic parameters define RDV-TP’s efficacy against diverse coronaviruses?
Comparative studies using RdRps from SARS-CoV-2, SARS-CoV, and MERS-CoV reveal:
- Incorporation efficiency : RDV-TP’s kpol (polymerization rate) is ~2.5-fold higher than ATP across coronaviruses.
- Selectivity : RDV-TP’s selectivity (ratio of incorporation vs. ATP) ranges from 3.1–3.8-fold, consistent with broad-spectrum activity .
- Exonuclease evasion : RDV-TP’s 1′-cyano modification prevents excision by viral proofreading exonucleases (e.g., nsp14), unlike other nucleotide analogs .
Q. How do pharmacokinetic studies optimize intracellular RDV-TP concentrations?
- Dose selection : Clinical regimens (200 mg loading dose, 100 mg maintenance) are derived from non-human primate models, ensuring intracellular RDV-TP levels exceed IC90 (9.9 µM) for ≥24 hours .
- Metabolic profiling : LC-MS analysis of human PBMCs confirms efficient conversion (50–70% of administered dose) to RDV-TP .
Q. What secondary viral processes are affected by RDV-TP beyond RdRp inhibition?
RDV-TP disrupts:
- Viral capping : Inhibition of NiRAN GTase activity reduces m<sup>7</sup>G cap synthesis, impairing viral RNA stability and immune evasion .
- Subgenomic RNA production : In enteroviruses, RDV-TP blocks both genomic and complementary RNA synthesis, expanding its antiviral scope .
Q. How do computational models predict RDV-TP’s efficacy against emerging variants?
- Molecular docking : Simulations (e.g., AutoDock Vina) assess RDV-TP binding affinity to mutant RdRps (e.g., NSP12-P323L). RDV-TP maintains strong binding (ΔG = −7.2 kcal/mol) despite mutations .
- Machine learning : Training on RdRp sequences (e.g., COPA scores) identifies conserved residues (e.g., Asp618) critical for RDV-TP activity across coronaviruses .
Properties
IUPAC Name |
[[(2R,3S,4R,5R)-5-(4-aminopyrrolo[2,1-f][1,2,4]triazin-7-yl)-5-cyano-3,4-dihydroxyoxolan-2-yl]methoxy-hydroxyphosphoryl] phosphono hydrogen phosphate | |
---|---|---|
Source | PubChem | |
URL | https://pubchem.ncbi.nlm.nih.gov | |
Description | Data deposited in or computed by PubChem | |
InChI |
InChI=1S/C12H16N5O13P3/c13-4-12(8-2-1-6-11(14)15-5-16-17(6)8)10(19)9(18)7(28-12)3-27-32(23,24)30-33(25,26)29-31(20,21)22/h1-2,5,7,9-10,18-19H,3H2,(H,23,24)(H,25,26)(H2,14,15,16)(H2,20,21,22)/t7-,9-,10-,12+/m1/s1 | |
Source | PubChem | |
URL | https://pubchem.ncbi.nlm.nih.gov | |
Description | Data deposited in or computed by PubChem | |
InChI Key |
DFVPCNAMNAPBCX-LTGWCKQJSA-N | |
Source | PubChem | |
URL | https://pubchem.ncbi.nlm.nih.gov | |
Description | Data deposited in or computed by PubChem | |
Canonical SMILES |
C1=C2C(=NC=NN2C(=C1)C3(C(C(C(O3)COP(=O)(O)OP(=O)(O)OP(=O)(O)O)O)O)C#N)N | |
Source | PubChem | |
URL | https://pubchem.ncbi.nlm.nih.gov | |
Description | Data deposited in or computed by PubChem | |
Isomeric SMILES |
C1=C2C(=NC=NN2C(=C1)[C@]3([C@@H]([C@@H]([C@H](O3)COP(=O)(O)OP(=O)(O)OP(=O)(O)O)O)O)C#N)N | |
Source | PubChem | |
URL | https://pubchem.ncbi.nlm.nih.gov | |
Description | Data deposited in or computed by PubChem | |
Molecular Formula |
C12H16N5O13P3 | |
Source | PubChem | |
URL | https://pubchem.ncbi.nlm.nih.gov | |
Description | Data deposited in or computed by PubChem | |
Molecular Weight |
531.20 g/mol | |
Source | PubChem | |
URL | https://pubchem.ncbi.nlm.nih.gov | |
Description | Data deposited in or computed by PubChem | |
CAS No. |
1355149-45-9 | |
Record name | Remdesivir triphosphate | |
Source | ChemIDplus | |
URL | https://pubchem.ncbi.nlm.nih.gov/substance/?source=chemidplus&sourceid=1355149459 | |
Description | ChemIDplus is a free, web search system that provides access to the structure and nomenclature authority files used for the identification of chemical substances cited in National Library of Medicine (NLM) databases, including the TOXNET system. | |
Record name | GS-441524 TRIPHOSPHATE | |
Source | FDA Global Substance Registration System (GSRS) | |
URL | https://gsrs.ncats.nih.gov/ginas/app/beta/substances/AEL0YED4SU | |
Description | The FDA Global Substance Registration System (GSRS) enables the efficient and accurate exchange of information on what substances are in regulated products. Instead of relying on names, which vary across regulatory domains, countries, and regions, the GSRS knowledge base makes it possible for substances to be defined by standardized, scientific descriptions. | |
Explanation | Unless otherwise noted, the contents of the FDA website (www.fda.gov), both text and graphics, are not copyrighted. They are in the public domain and may be republished, reprinted and otherwise used freely by anyone without the need to obtain permission from FDA. Credit to the U.S. Food and Drug Administration as the source is appreciated but not required. | |
Disclaimer and Information on In-Vitro Research Products
Please be aware that all articles and product information presented on BenchChem are intended solely for informational purposes. The products available for purchase on BenchChem are specifically designed for in-vitro studies, which are conducted outside of living organisms. In-vitro studies, derived from the Latin term "in glass," involve experiments performed in controlled laboratory settings using cells or tissues. It is important to note that these products are not categorized as medicines or drugs, and they have not received approval from the FDA for the prevention, treatment, or cure of any medical condition, ailment, or disease. We must emphasize that any form of bodily introduction of these products into humans or animals is strictly prohibited by law. It is essential to adhere to these guidelines to ensure compliance with legal and ethical standards in research and experimentation.