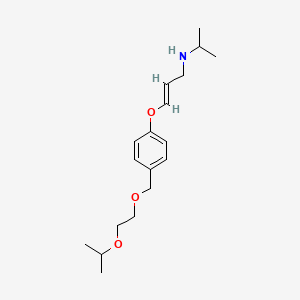
Dehydroxy bisoprolol
Overview
Description
Bisoprolol is a highly selective β1-adrenoceptor antagonist widely used to treat hypertension, angina, and chronic heart failure. It reduces myocardial oxygen demand by lowering heart rate and contractility, thereby alleviating cardiac workload . Its pharmacokinetic profile includes high bioavailability (~90%), renal excretion (~50%), and hepatic metabolism via CYP2D6 and CYP3A5 . Bisoprolol’s efficacy and safety have been validated in large-scale trials like CIBIS II, demonstrating mortality reduction in heart failure patients .
Preparation Methods
Industrial Synthesis as a Byproduct in Bisoprolol Production
Dehydroxy bisoprolol predominantly forms during key steps of bisoprolol synthesis, particularly during alkylation or epoxide ring-opening reactions. Patent WO2007069266A2 outlines a bisoprolol manufacturing process where 4-[(2-isopropoxyethoxy)methyl]phenol reacts with epichlorohydrin to form 2-[[4-(2-isopropoxyethoxy)methyl]phenoxymethyl]oxirane . Residual moisture or elevated temperatures during this step can lead to dehydration, generating dehydroxy derivatives.
Direct Synthesis via Dehydroxylation of Bisoprolol
Targeted synthesis of this compound involves deliberate removal of hydroxyl groups from bisoprolol or its precursors. ChemicalBook and SynThink describe two primary approaches:
Acid-Catalyzed Dehydration
-
Reagents : Thionyl chloride (SOCl₂) or phosphorus oxychloride (POCl₃) .
-
Mechanism : Protonation of the hydroxyl group followed by nucleophilic substitution or elimination.
-
Procedure :
Thermal Decomposition
-
Conditions : Heating bisoprolol fumarate at 120–150°C under vacuum .
-
Outcome : Eliminates water molecules, forming dehydroxy derivatives.
-
Limitation : Generates multiple degradation products, necessitating HPLC purification .
Synthesis from Bisoprolol Intermediates
This compound can also arise during the synthesis of bisoprolol intermediates. For example, 4-[(2-isopropoxyethoxy)methyl]phenol (Intermediate I) is susceptible to dehydration under acidic conditions:
Intermediate I Dehydration
-
Reaction Setup :
-
Intermediate I (1.0 equiv), toluenesulfonic acid (0.1 equiv), and toluene.
-
Heated under reflux with a Dean-Stark trap for water removal.
-
-
Outcome :
Analytical Characterization and Validation
Chromatographic Methods
-
Column Mobile Phase Flow Rate Detection Retention Time C18 (250 mm) MeOH:NH₄OAc (70:30) 1.0 mL/min UV 230 nm 8.2 min -
Impurity Profile : this compound typically elutes after bisoprolol (Relative Retention Time: 1.2–1.3) .
Spectroscopic Data
-
¹H NMR (400 MHz, CDCl₃) : δ 6.85 (d, 2H, aromatic), 4.55 (s, 2H, -OCH₂-), 3.70 (m, 1H, isopropyl), 2.90 (m, 2H, -CH₂NH-) .
-
IR (KBr) : 1680 cm⁻¹ (C=C), absence of O-H stretch.
Industrial-Scale Optimization
Patent WO2016135616A1 highlights strategies to minimize this compound formation during bisoprolol production :
-
Silica Gel Adsorption : Adding activated silica post-alkylation sequesters residual acids, reducing dehydration .
-
Low-Temperature Processing : Conducting epichlorohydrin reactions below 20°C suppresses side reactions .
-
In-Line Analytics : FTIR and HPLC monitors enable real-time adjustment of reaction parameters .
Comparative Analysis of Synthesis Routes
Chemical Reactions Analysis
Types of Reactions
Dehydroxy bisoprolol can undergo various chemical reactions, including:
Oxidation: this compound can be oxidized to form corresponding ketones or aldehydes.
Reduction: It can be reduced to form alcohols or amines.
Substitution: It can undergo nucleophilic substitution reactions where functional groups are replaced by other nucleophiles.
Common Reagents and Conditions
Oxidation: Common oxidizing agents include potassium permanganate and chromium trioxide.
Reduction: Reducing agents such as lithium aluminum hydride or sodium borohydride are used.
Substitution: Reagents like sodium hydroxide or potassium tert-butoxide are employed in substitution reactions.
Major Products Formed
Oxidation: Formation of ketones or aldehydes.
Reduction: Formation of alcohols or amines.
Substitution: Formation of various substituted derivatives depending on the nucleophile used.
Scientific Research Applications
Dehydroxy bisoprolol has several scientific research applications, including:
Chemistry: Used as a model compound to study dehydroxylation reactions and their mechanisms.
Biology: Investigated for its potential effects on biological systems, particularly in cardiovascular research.
Medicine: Explored for its potential therapeutic benefits and pharmacological properties.
Industry: Utilized in the development of new beta-blocker derivatives with improved efficacy and reduced side effects.
Mechanism of Action
The mechanism of action of dehydroxy bisoprolol involves its interaction with beta-adrenergic receptors, particularly the beta-1 adrenergic receptors found in the heart. By blocking these receptors, this compound reduces the effects of catecholamines (adrenaline and noradrenaline), leading to decreased heart rate and blood pressure. This action helps in managing conditions like hypertension and heart failure.
Comparison with Similar Compounds
Comparison with Bisoprolol Derivatives
Table 1: Molecular Docking and ADMET Properties of Bisoprolol and Derivatives
Compound | Binding Energy (kcal mol⁻¹) | Pgp Substrate Inhibition | CYP3A4 Interaction |
---|---|---|---|
Bisoprolol | -6.74 | Yes | No |
N-acetyl bisoprolol | -7.03 | Yes | No |
N-formyl bisoprolol | -7.63 | No | No |
Key Findings :
- Binding Affinity: Both derivatives exhibit higher binding energy to the 4BVN protein (β1-adrenoceptor) than bisoprolol, suggesting stronger target engagement .
- All compounds avoid CYP3A4 interference, minimizing metabolic conflicts .
- Stability : Molecular dynamics simulations confirm stable ligand-protein complexes (RMSD ≈2 Å) for all three compounds .
Comparison with Other β-Blockers
Metoprolol CR/ZOK
- Efficacy: Bisoprolol showed superior heart rate (HR) reduction (p<0.05) and non-inferior blood pressure (BP) control vs. metoprolol CR/ZOK in mild-to-moderate hypertension .
- Safety: Adverse event rates were comparable, with bisoprolol demonstrating lower noncompliance (3.53% vs. 7.32%) .
Atenolol
- Sympathetic Activity : Bisoprolol reduced sympathetic nervous activity (SNA) and central aortic pressure (CAP) more effectively in hypertensive patients, attributed to its higher β1-selectivity .
Propranolol and Celiprolol
- Potency: Propranolol (IC₅₀ = 0.1 µM) was 46× more potent than celiprolol (4.6 µM) in bronchoconstriction, with bisoprolol (1.2 µM) intermediate .
- Selectivity: Bisoprolol’s β1-selectivity minimizes bronchoconstrictive risks compared to non-selective propranolol .
Table 2: Pharmacokinetic and Clinical Profiles of β-Blockers
Transdermal vs. Oral Formulations
- Efficacy : Bisoprolol transdermal patches (TY-0201) achieved equivalent HR and BP reduction to oral tablets, with comparable safety .
- Compliance : Transdermal delivery avoids first-pass metabolism and may improve adherence in patients with gastrointestinal issues .
Pharmacogenetic Considerations
- CYP Polymorphisms : CYP2D6 poor metabolizers show 30% higher bisoprolol plasma concentrations, necessitating dose adjustments in renal/hepatic impairment .
Biological Activity
Dehydroxy bisoprolol, a derivative of the well-known beta-blocker bisoprolol, has garnered attention for its potential biological activities, particularly in cardiovascular pharmacology. This article explores its mechanisms of action, efficacy in clinical settings, and comparative studies with other antihypertensive agents.
Overview of Bisoprolol and Its Derivatives
Bisoprolol is a selective beta-1 adrenergic antagonist primarily used to manage hypertension and heart failure. Its mechanism involves the inhibition of β1-receptors in the heart, leading to decreased heart rate and myocardial contractility, which reduces cardiac workload and oxygen demand . this compound, while less studied than its parent compound, is hypothesized to retain similar pharmacological properties due to its structural similarities.
The biological activity of this compound can be understood through its interaction with adrenergic receptors:
- Beta-1 Receptor Antagonism : Like bisoprolol, this compound is expected to selectively inhibit β1-adrenoceptors, leading to a reduction in heart rate and contractility.
- Renin Inhibition : It may also reduce renin release from the kidneys, contributing to lower blood pressure through the renin-angiotensin system .
Pharmacokinetics
The pharmacokinetic profile of this compound remains largely uncharacterized. However, based on bisoprolol's profile:
- Absorption : Bisoprolol is well absorbed with a bioavailability of approximately 90% .
- Peak Concentration : Typically achieved within 2-4 hours post-administration.
- Half-Life : Approximately 10 hours for bisoprolol suggests a potentially similar duration for this compound.
Comparative Studies
Recent studies have explored the efficacy of bisoprolol and its derivatives in various clinical settings:
- Heart Failure : A meta-analysis involving 3,288 patients demonstrated that bisoprolol significantly reduced mortality and hospitalizations related to heart failure compared to placebo (29.3% reduction in total death) .
- Hypertension Management : A study comparing low-dose combinations of bisoprolol/hydrochlorothiazide showed significant reductions in both systolic and diastolic blood pressure over a 24-hour period .
Case Studies
- Transdermal versus Oral Administration : A study involving patients switching from oral bisoprolol to a transdermal patch indicated improved heart rate control and reduced incidence of premature ventricular contractions (PVCs) during sleep . This suggests that formulation might influence the drug's effectiveness and patient compliance.
Molecular Docking Studies
Recent computational studies have utilized molecular docking techniques to evaluate the binding affinity of this compound to various adrenergic receptors. These studies suggest that:
- This compound may exhibit comparable binding energies to beta-1 receptors as seen with bisoprolol itself.
- The binding energies reported were significant, indicating potential efficacy as an antihypertensive agent .
Data Summary Table
Q & A
Basic Research Questions
Q. What analytical methods are recommended for quantifying dehydroxy bisoprolol and its related substances in pharmaceutical formulations?
High-performance liquid chromatography (HPLC) coupled with UV detection is widely used for impurity profiling and forced degradation studies. For structural characterization of degradation products like this compound, HPLC should be validated per ICH guidelines for specificity, accuracy, and precision. A resolution ≥2.24 between bisoprolol and its impurities (e.g., impurity G) is critical for reliable quantification . LC-MS/MS methods are preferred for pharmacokinetic studies due to their sensitivity (e.g., LLOQ ~1 ng/mL) and ability to distinguish metabolites in biological matrices .
Q. What pharmacokinetic parameters should be prioritized in bioavailability studies of this compound?
Key parameters include Cmax , Tmax , AUC0–t , and elimination half-life. Stability testing under varying pH and temperature conditions is essential for extemporaneous formulations. Bioequivalence studies should follow FDA/EMA guidelines, using crossover designs with 90% confidence intervals for AUC and Cmax ratios (80–125%) .
Q. How should researchers design clinical trials to evaluate this compound’s hemodynamic effects?
Randomized controlled trials (RCTs) with double-blinding and placebo controls are standard. Primary endpoints may include changes in brachial/aortic systolic blood pressure (SBP) and pulse pressure (PP). For example, bisoprolol reduced aortic SBP by 8.2 mmHg vs. 5.1 mmHg for atenolol in hypertensive patients, requiring ≥80% statistical power to detect such differences .
Advanced Research Questions
Q. How can forced degradation studies elucidate the stability profile of this compound?
Subject the compound to stress conditions (acid/base hydrolysis, oxidation, thermal/photo degradation) and analyze degradation products via HPLC-UV/MS. For bisoprolol fumarate, oxidative conditions produce impurities like this compound, requiring resolution ≥2.24 from the parent compound. Structural confirmation via NMR or high-resolution MS is recommended for novel degradation products .
Q. What methodologies optimize formulation parameters for this compound matrix tablets?
Use response surface methodology (RSM) with a 2<sup>3</sup> factorial design to model interactions between variables (e.g., polymer concentration, compression force). Desirability functions in software like Design Expert® can identify optimal settings (e.g., 15.28 mg polymer, 4.65 kg/cm<sup>2</sup> hardness) to achieve target dissolution profiles (e.g., 41.61% release at 6 hours) .
Q. How do environmental persistence and toxicity studies inform this compound’s ecotoxicological risk?
Assess biodegradation rates in aqueous systems (OECD 301 tests) and bioaccumulation potential (log Kow <3 indicates low risk). Chronic toxicity assays using Daphnia magna or algae (OECD 202/201) show bisoprolol has low environmental risk (PNEC/PRQ <1). Sales data and predicted environmental concentrations (PEC) should be modeled for regional risk assessments .
Q. What statistical approaches resolve contradictions in comparative effectiveness studies (e.g., bisoprolol vs. carvedilol)?
Propensity score matching or Cox proportional hazards models adjust for confounders (e.g., comorbidities, dialysis status). In hemodialysis patients, bisoprolol showed a 14% lower mortality risk vs. carvedilol (HR: 0.86, 95% CI: 0.76–0.98), requiring sensitivity analyses to validate robustness .
Q. How does this compound interact with anesthetic agents in surgical settings?
Preoperative administration (e.g., 5 mg oral bisoprolol) reduces intraoperative isoflurane requirements (0.7 ± 0.32 vol% vs. 1.5 ± 0.55 vol% for placebo) by stabilizing hemodynamics. Monitor heart rate and mean arterial pressure to avoid hypotension .
Q. What synergies exist between this compound and ACE inhibitors like perindopril?
Combination therapies target complementary pathways: bisoprolol reduces cardiac output via β1-blockade, while perindopril lowers vascular resistance. In post hoc analyses, this combination improved SBP by 18.3 mmHg (vs. 12.1 mmHg for monotherapy) with no adverse pharmacokinetic interactions .
Q. Methodological Resources
- Impurity Profiling : Use EP/USP reference standards and ICH Q3B guidelines .
- Clinical Data Analysis : Apply CONSORT guidelines for RCT reporting and mixed-effects models for longitudinal data .
- Environmental Modeling : Apply USEtox® or E-FAST for risk quantification .
Note: Avoid unreliable sources (e.g., BenchChem). Prioritize peer-reviewed journals and regulatory guidelines.
Properties
IUPAC Name |
(E)-N-propan-2-yl-3-[4-(2-propan-2-yloxyethoxymethyl)phenoxy]prop-2-en-1-amine | |
---|---|---|
Source | PubChem | |
URL | https://pubchem.ncbi.nlm.nih.gov | |
Description | Data deposited in or computed by PubChem | |
InChI |
InChI=1S/C18H29NO3/c1-15(2)19-10-5-11-22-18-8-6-17(7-9-18)14-20-12-13-21-16(3)4/h5-9,11,15-16,19H,10,12-14H2,1-4H3/b11-5+ | |
Source | PubChem | |
URL | https://pubchem.ncbi.nlm.nih.gov | |
Description | Data deposited in or computed by PubChem | |
InChI Key |
JZRKUNUKKCUCNI-VZUCSPMQSA-N | |
Source | PubChem | |
URL | https://pubchem.ncbi.nlm.nih.gov | |
Description | Data deposited in or computed by PubChem | |
Canonical SMILES |
CC(C)NCC=COC1=CC=C(C=C1)COCCOC(C)C | |
Source | PubChem | |
URL | https://pubchem.ncbi.nlm.nih.gov | |
Description | Data deposited in or computed by PubChem | |
Isomeric SMILES |
CC(C)NC/C=C/OC1=CC=C(C=C1)COCCOC(C)C | |
Source | PubChem | |
URL | https://pubchem.ncbi.nlm.nih.gov | |
Description | Data deposited in or computed by PubChem | |
Molecular Formula |
C18H29NO3 | |
Source | PubChem | |
URL | https://pubchem.ncbi.nlm.nih.gov | |
Description | Data deposited in or computed by PubChem | |
DSSTOX Substance ID |
DTXSID50747438 | |
Record name | (2E)-N-(Propan-2-yl)-3-[4-({2-[(propan-2-yl)oxy]ethoxy}methyl)phenoxy]prop-2-en-1-amine | |
Source | EPA DSSTox | |
URL | https://comptox.epa.gov/dashboard/DTXSID50747438 | |
Description | DSSTox provides a high quality public chemistry resource for supporting improved predictive toxicology. | |
Molecular Weight |
307.4 g/mol | |
Source | PubChem | |
URL | https://pubchem.ncbi.nlm.nih.gov | |
Description | Data deposited in or computed by PubChem | |
CAS No. |
2180924-96-1, 1217245-60-7 | |
Record name | Dehydro bisoprolol, (E) | |
Source | ChemIDplus | |
URL | https://pubchem.ncbi.nlm.nih.gov/substance/?source=chemidplus&sourceid=2180924961 | |
Description | ChemIDplus is a free, web search system that provides access to the structure and nomenclature authority files used for the identification of chemical substances cited in National Library of Medicine (NLM) databases, including the TOXNET system. | |
Record name | (2E)-N-(Propan-2-yl)-3-[4-({2-[(propan-2-yl)oxy]ethoxy}methyl)phenoxy]prop-2-en-1-amine | |
Source | EPA DSSTox | |
URL | https://comptox.epa.gov/dashboard/DTXSID50747438 | |
Description | DSSTox provides a high quality public chemistry resource for supporting improved predictive toxicology. | |
Record name | DEHYDRO BISOPROLOL, (E)- | |
Source | FDA Global Substance Registration System (GSRS) | |
URL | https://gsrs.ncats.nih.gov/ginas/app/beta/substances/HJG7AX3T7X | |
Description | The FDA Global Substance Registration System (GSRS) enables the efficient and accurate exchange of information on what substances are in regulated products. Instead of relying on names, which vary across regulatory domains, countries, and regions, the GSRS knowledge base makes it possible for substances to be defined by standardized, scientific descriptions. | |
Explanation | Unless otherwise noted, the contents of the FDA website (www.fda.gov), both text and graphics, are not copyrighted. They are in the public domain and may be republished, reprinted and otherwise used freely by anyone without the need to obtain permission from FDA. Credit to the U.S. Food and Drug Administration as the source is appreciated but not required. | |
Retrosynthesis Analysis
AI-Powered Synthesis Planning: Our tool employs the Template_relevance Pistachio, Template_relevance Bkms_metabolic, Template_relevance Pistachio_ringbreaker, Template_relevance Reaxys, Template_relevance Reaxys_biocatalysis model, leveraging a vast database of chemical reactions to predict feasible synthetic routes.
One-Step Synthesis Focus: Specifically designed for one-step synthesis, it provides concise and direct routes for your target compounds, streamlining the synthesis process.
Accurate Predictions: Utilizing the extensive PISTACHIO, BKMS_METABOLIC, PISTACHIO_RINGBREAKER, REAXYS, REAXYS_BIOCATALYSIS database, our tool offers high-accuracy predictions, reflecting the latest in chemical research and data.
Strategy Settings
Precursor scoring | Relevance Heuristic |
---|---|
Min. plausibility | 0.01 |
Model | Template_relevance |
Template Set | Pistachio/Bkms_metabolic/Pistachio_ringbreaker/Reaxys/Reaxys_biocatalysis |
Top-N result to add to graph | 6 |
Feasible Synthetic Routes
Disclaimer and Information on In-Vitro Research Products
Please be aware that all articles and product information presented on BenchChem are intended solely for informational purposes. The products available for purchase on BenchChem are specifically designed for in-vitro studies, which are conducted outside of living organisms. In-vitro studies, derived from the Latin term "in glass," involve experiments performed in controlled laboratory settings using cells or tissues. It is important to note that these products are not categorized as medicines or drugs, and they have not received approval from the FDA for the prevention, treatment, or cure of any medical condition, ailment, or disease. We must emphasize that any form of bodily introduction of these products into humans or animals is strictly prohibited by law. It is essential to adhere to these guidelines to ensure compliance with legal and ethical standards in research and experimentation.