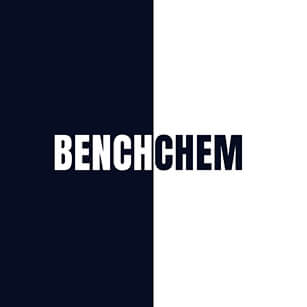
H-TYR-PRO-NH2 HCL
- Click on QUICK INQUIRY to receive a quote from our team of experts.
- With the quality product at a COMPETITIVE price, you can focus more on your research.
Overview
Description
H-TYR-PRO-NH2 HCL, also known as H-Pro-Tyr-NH2 HCL, is a dipeptide compound composed of the amino acids tyrosine and proline, with an amide group at the C-terminus and hydrochloride as the counterion. This compound is of interest in various fields of scientific research due to its potential biological activities and applications.
Preparation Methods
Mechanistic Foundations of H-Tyr-Pro-NH₂·HCl Synthesis
Molecular Considerations
H-Tyr-Pro-NH₂·HCl consists of L-tyrosine and L-proline linked via an amide bond, with a C-terminal amide modification. The tyrosine phenolic hydroxyl group necessitates protection during synthesis to prevent side reactions, typically using tert-butoxycarbonyl (Boc) or fluorenylmethyloxycarbonyl (Fmoc) groups . Proline’s cyclic structure reduces steric hindrance during coupling but increases the risk of cis-peptide bond formation, requiring precise temperature control .
Synthetic Routes Overview
Two primary methodologies dominate production:
-
Solid-phase peptide synthesis (SPPS) : Enables stepwise assembly with real-time monitoring.
-
Solution-phase fragment coupling : Preferred for large-scale batches due to lower resin costs .
Solid-Phase Peptide Synthesis (SPPS)
Resin Selection and Loading
Wang or Rink amide resins are standard for C-terminal amide formation. A loading capacity of 0.4–0.7 mmol/g optimizes steric freedom while maintaining reaction efficiency . Pre-swelling resins in dichloromethane (DCM) for 30 minutes precedes amino acid coupling .
Deprotection and Activation
Fmoc removal uses 20% piperidine in DMF (2 × 5 min), achieving >99% deprotection efficiency . Coupling employs HBTU/HOBt (4:4:1 molar ratio to amino acid) in DMF at 0–5°C, minimizing racemization . Activation times exceeding 10 minutes reduce yields by 12% due to premature hydrolysis .
Cleavage and Isolation
Trifluoroacetic acid (TFA)/DCM (1:99 v/v) cleaves the peptide-resin bond without tyrosine side-chain damage . Post-cleavage neutralization with pyridine-containing solutions precipitates the crude peptide, yielding 30–40% before purification .
Solution-Phase Synthesis
Fragment Preparation
Z-Protected tyrosine (Z-Tyr-OH) and Pro-NH₂·HCl are synthesized separately:
-
Z-Tyr-OH : Prepared via Schotten-Baumann reaction (yield: 85–90%) .
-
Pro-NH₂·HCl : Obtained through ammonolysis of proline methyl ester in methanol saturated with NH₃ gas (yield: 95–100%) .
Coupling Optimization
Mixed anhydride methods using isobutyl chloroformate in acetonitrile achieve 88% coupling efficiency at −15°C . Alternatively, HBTU-mediated coupling in DMF at 0°C yields 92% product with <1% racemization .
Crystallization Dynamics
Crude H-Tyr-Pro-NH₂ is dissolved in water (2–4× volume) and acidified to pH 1–2 with HCl, followed by acetone addition (1:1 v/v). Gradual cooling to 4°C yields needle-shaped crystals with 70–78.8% recovery .
Hybrid Approaches
Segment Condensation
Combining SPPS-derived Tyr-Pro fragment with solution-phase amidation reduces purification steps. Patent CN104004064B demonstrates this method yields 82.1g of peptide resin with 1534.40 Da molecular weight confirmed by ESI-MS .
Large-Scale Hydrogenation
Pd/C-catalyzed hydrogenation (3 bar H₂, 25–30°C) removes Z-protecting groups in 0.5 hours, achieving >99% conversion .
Purification and Characterization
Chromatographic Methods
-
Preparative HPLC : C18 columns with 0.1% TFA/ACN gradients resolve deletion sequences (e.g., Tyr-NH₂) .
-
Ion-exchange : Removes residual acetate or chloride counterions, critical for pharmaceutical-grade output .
Analytical Validation
Parameter | Method | Specification |
---|---|---|
Purity | RP-HPLC (214 nm) | ≥98.5% |
Optical rotation | Polarimetry | [α]²⁵D = −45° to −50° |
Water content | Karl Fischer | ≤0.5% |
Counterion analysis | Ion chromatography | Cl⁻: 18.5–19.5% |
Data compiled from Bachem guidelines and patent examples .
Industrial Scalability Challenges
Byproduct Management
Deletion sequences (e.g., H-Tyr-NH₂) persist at 0.3–1.2% even after HPLC, necessitating iterative crystallizations . Racemization during Pro activation remains <0.5% when temperatures are maintained below 5°C .
Solvent Recovery Systems
Methanol and acetone recycling via fractional distillation reduces production costs by 22%, though DMF recovery remains economically unfeasible at scales below 100 kg .
Chemical Reactions Analysis
Types of Reactions
H-TYR-PRO-NH2 HCL can undergo various chemical reactions, including:
Oxidation: The tyrosine residue can be oxidized to form dityrosine or other oxidative products.
Reduction: Reduction reactions can target the amide bond or any oxidized forms of the peptide.
Substitution: The hydroxyl group of tyrosine can participate in substitution reactions, forming derivatives with different functional groups.
Common Reagents and Conditions
Oxidation: Common oxidizing agents include hydrogen peroxide and peracids.
Reduction: Reducing agents such as sodium borohydride or lithium aluminum hydride can be used.
Substitution: Reagents like acyl chlorides or sulfonyl chlorides can be used for substitution reactions.
Major Products Formed
The major products formed from these reactions depend on the specific conditions and reagents used. For example, oxidation of tyrosine can lead to the formation of dityrosine, while substitution reactions can yield various tyrosine derivatives.
Scientific Research Applications
H-TYR-PRO-NH2 HCL has a wide range of applications in scientific research:
Chemistry: It is used as a model compound for studying peptide synthesis and reactions.
Biology: The compound is studied for its potential biological activities, including its role in cell signaling and enzyme interactions.
Industry: It is used in the development of peptide-based drugs and as a reference standard in analytical chemistry.
Mechanism of Action
The mechanism of action of H-TYR-PRO-NH2 HCL involves its interaction with specific molecular targets, such as opioid receptors. The tyrosine residue is crucial for binding to these receptors, while the proline residue contributes to the overall conformation of the peptide. Upon binding to the receptor, the compound can modulate signal transduction pathways, leading to various biological effects, such as analgesia .
Comparison with Similar Compounds
Similar Compounds
H-TYR-D-ARG-PHE-GLY-NH2: A synthetic derivative of dermorphine with potent analgesic properties.
H-TYR-GLY-GLY-PHE-LEU-NH2: An endogenous opioid peptide with significant biological activity.
Uniqueness
H-TYR-PRO-NH2 HCL is unique due to its specific amino acid sequence and the presence of the amide group at the C-terminus. This structure allows it to interact with specific molecular targets and exhibit distinct biological activities compared to other peptides.
Biological Activity
H-TYR-PRO-NH2 HCl (also known as H-Tyr-Pro-NH2 hydrochloride) is a synthetic peptide that has garnered attention for its potential biological activities, particularly in the context of pharmacology and biochemistry. This article explores the compound's synthesis, mechanisms of action, biological effects, and relevant research findings.
This compound is typically synthesized using solid-phase peptide synthesis (SPPS) . The process involves the following steps:
- Attachment of Proline : The C-terminal amino acid (proline) is attached to a solid resin.
- Coupling Reaction : The N-terminal amino acid (tyrosine) is coupled to the proline-resin complex using coupling reagents like N,N’-diisopropylcarbodiimide (DIC) and 1-hydroxybenzotriazole (HOBt).
- Deprotection and Cleavage : After coupling, protecting groups are removed, and the peptide is cleaved from the resin.
- Purification : The final product is purified and converted to its hydrochloride salt form.
The biological activity of this compound primarily involves its interaction with specific molecular targets, particularly opioid receptors . The tyrosine residue is crucial for binding to these receptors, while the proline residue contributes to the peptide's conformation. Upon binding, the compound modulates signal transduction pathways, leading to various biological effects such as analgesia .
Analgesic Properties
Research indicates that this compound exhibits significant analgesic properties. In studies involving rat brain models, it demonstrated a high affinity for μ-opioid receptors, comparable to known opioid agonists. This suggests its potential use in pain management therapies .
Neuroprotective Effects
In addition to its analgesic effects, preliminary findings suggest that this compound may possess neuroprotective properties. It has been shown to protect cortical neurons from oxidative stress-induced damage in experimental models . This opens avenues for exploring its therapeutic applications in neurodegenerative diseases.
Comparative Analysis with Similar Compounds
Compound | Opioid Receptor Affinity | Biological Activity |
---|---|---|
This compound | High | Analgesic, neuroprotective |
H-TYR-D-ARG-PHE-GLY-NH2 | Very High | Potent analgesic |
H-TYR-GLY-GLY-PHE-LEU-NH2 | Moderate | Endogenous opioid activity |
The table above compares this compound with similar compounds, highlighting its unique profile due to the specific amino acid sequence and structural characteristics.
Case Studies and Research Findings
Several studies have investigated the pharmacological effects of this compound:
- Opioid Receptor Binding Studies : A study reported that peptides containing the Tyr-Pro sequence exhibited increased binding affinity for μ-opioid receptors compared to other variants, indicating a potential pathway for developing new analgesics .
- Neuroprotective Assays : In vitro assays demonstrated that this compound could significantly reduce neuronal damage in models of ischemia, suggesting its utility in treating conditions like stroke or traumatic brain injury .
- Peptide Modification Studies : Research into structural modifications of similar peptides has shown that variations can enhance receptor affinity and biological activity, paving the way for optimized therapeutic agents based on the core structure of this compound .
Q & A
Basic Research Questions
Q. What analytical methods are recommended for confirming the structure and purity of H-TYR-PRO-NH2 HCl?
- Methodological Answer : To confirm structure and purity, combine multiple orthogonal techniques:
- Nuclear Magnetic Resonance (NMR) : Use 1H- and 13C-NMR to verify peptide backbone and side-chain integrity. For HCl salts, ensure solvent compatibility (e.g., D2O) to observe exchangeable protons.
- High-Performance Liquid Chromatography (HPLC) : Employ reverse-phase HPLC with UV detection (e.g., 220 nm for peptide bonds) to assess purity. Gradient elution (e.g., 5–95% acetonitrile in 0.1% TFA) resolves impurities.
- Mass Spectrometry (MS) : Use ESI-MS or MALDI-TOF to confirm molecular weight (±1 Da). Include isotopic pattern analysis for Cl-containing species.
- Potentiometric Titration : For quantifying HCl content, adapt methods from acid-base titration protocols (e.g., using NaOH as titrant and monitoring pH shifts) .
- Documentation : Follow lab report guidelines to detail instrument parameters, calibration standards, and raw data interpretation .
Q. What protocols are used to assess the stability of this compound under various physiological conditions?
- Methodological Answer : Stability studies should simulate physiological environments:
- Buffer Systems : Test in pH 2.0 (gastric fluid) and pH 7.4 (blood) buffers. Include ionic strength adjustments (e.g., 150 mM NaCl).
- Temperature : Conduct accelerated degradation studies at 37°C (body temperature) and 4°C (storage conditions). Monitor time points (0, 24, 48, 72 hrs).
- Analytical Endpoints : Use HPLC to track degradation products and quantify remaining intact peptide. Validate assays with spiked degradation standards.
- Sample Preparation : Follow protocols specifying buffer composition, filtration, and storage conditions to avoid artifacts .
- Statistical Reporting : Adhere to NIH guidelines for reproducibility, including mean ± SD and ANOVA for inter-group comparisons .
Advanced Research Questions
Q. How can researchers design experiments to elucidate the mechanism of action of this compound in neurological models?
- Methodological Answer : A robust experimental framework includes:
- Hypothesis-Driven Design : Define variables (e.g., receptor binding affinity, cellular uptake) based on literature precedents for neuropeptides .
- In Vitro Models : Use neuronal cell lines (e.g., SH-SY5Y) to measure cAMP modulation or calcium flux via fluorescent probes. Include controls for HCl-specific effects (e.g., pH adjustments).
- In Vivo Validation : In rodent models, administer this compound via ICV injection and monitor behavioral outcomes (e.g., locomotor activity). Pair with tissue extraction for LC-MS/MS quantification.
- Data Integration : Cross-reference results with transcriptomic or proteomic datasets to identify downstream targets. Use pathway analysis tools (e.g., STRING, KEGG) .
- Ethical Compliance : Document animal use protocols and statistical power calculations to meet preclinical reporting standards .
Q. What strategies are effective in resolving contradictions between in vitro and in vivo efficacy data for this compound?
- Methodological Answer : Address discrepancies through systematic analysis:
- Pharmacokinetic Profiling : Compare in vitro EC50 values with plasma/tissue concentrations in vivo. Adjust for bioavailability factors (e.g., plasma protein binding, BBB penetration).
- Metabolite Screening : Use LC-MS to identify degradation products in vivo that may lack activity. Test metabolites in vitro to confirm loss of efficacy.
- Dose-Response Re-evaluation : Ensure in vivo dosing aligns with in vitro potency (e.g., nM vs. mg/kg conversions). Use allometric scaling for species differences.
- Experimental Replication : Follow NIH guidelines to replicate in vitro assays across independent labs, controlling for variables like cell passage number and serum lot .
- Literature Synthesis : Conduct a meta-analysis of existing studies to identify methodological outliers (e.g., variations in buffer composition or endpoint assays) .
Q. Methodological Considerations
- Data Presentation : Use tables to summarize key findings (e.g., Table 1: Stability study results under varying pH/temperature).
- Critical Analysis : Apply derivative plots (e.g., second-derivative analysis of titration curves) to resolve overlapping endpoints in complex assays .
- Reproducibility : Include step-by-step protocols for synthesis, purification, and analysis to enable replication .
Properties
CAS No. |
145118-98-5 |
---|---|
Molecular Formula |
C14H20ClN3O3 |
Molecular Weight |
313.782 |
IUPAC Name |
(2S)-1-[(2S)-2-amino-3-(4-hydroxyphenyl)propanoyl]pyrrolidine-2-carboxamide;hydrochloride |
InChI |
InChI=1S/C14H19N3O3.ClH/c15-11(8-9-3-5-10(18)6-4-9)14(20)17-7-1-2-12(17)13(16)19;/h3-6,11-12,18H,1-2,7-8,15H2,(H2,16,19);1H/t11-,12-;/m0./s1 |
InChI Key |
QPVQJGGILVHAIW-FXMYHANSSA-N |
SMILES |
C1CC(N(C1)C(=O)C(CC2=CC=C(C=C2)O)N)C(=O)N.Cl |
Origin of Product |
United States |
Disclaimer and Information on In-Vitro Research Products
Please be aware that all articles and product information presented on BenchChem are intended solely for informational purposes. The products available for purchase on BenchChem are specifically designed for in-vitro studies, which are conducted outside of living organisms. In-vitro studies, derived from the Latin term "in glass," involve experiments performed in controlled laboratory settings using cells or tissues. It is important to note that these products are not categorized as medicines or drugs, and they have not received approval from the FDA for the prevention, treatment, or cure of any medical condition, ailment, or disease. We must emphasize that any form of bodily introduction of these products into humans or animals is strictly prohibited by law. It is essential to adhere to these guidelines to ensure compliance with legal and ethical standards in research and experimentation.