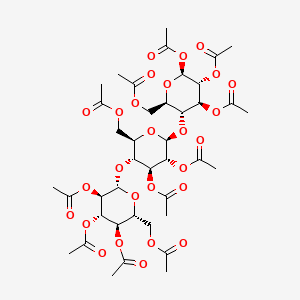
Triacetylcellulose
Overview
Description
Triacetylcellulose (TAC), also known as cellulose triacetate, is a cellulose derivative where at least 92% of the hydroxyl groups (-OH) on the glucose units of cellulose are acetylated . This full acetylation distinguishes TAC from other cellulose acetates, such as cellulose diacetate (DAC) or secondary acetate, which have lower degrees of substitution (DS). TAC is synthesized by reacting cellulose with acetic anhydride under controlled conditions, resulting in a DS of approximately 2.93 (nearly full acetylation) . It is widely utilized in high-performance applications, including optical films, chiral chromatography stationary phases, and sensor membranes, owing to its thermal stability, mechanical strength, and chemical resistance .
Preparation Methods
Solvent-Based Acetylation Using Ionic Liquids
Ionic liquids have emerged as green solvents for triacetylcellulose synthesis due to their ability to dissolve lignocellulosic biomass directly, bypassing energy-intensive pretreatment steps. In one approach, palm empty fruit bunch (EFB) cellulose was dissolved in 1-butyl-3-methylimidazolium chloride (BMIMCl), followed by acetylation with acetic anhydride at 55°C . This method achieved a DS of 2.93 in fewer steps compared to conventional routes, with nuclear magnetic resonance (NMR) confirming the substitution pattern . Similarly, pretreated sugar-cane bagasse underwent homogeneous acetylation in 1-ethyl-3-methylimidazolium acetate (EmimOAc) using isopropenyl acetate (IPA) as an acetyl donor, yielding this compound with a DS of 2.98 and 90% glucose purity . The ionic liquid acted as both solvent and organocatalyst, enabling delignification and transesterification in a single pot .
A key advantage of ionic liquid-mediated processes is their compatibility with unrefined biomass, reducing reliance on high-purity cellulose. However, challenges remain in solvent recovery and cost, as ionic liquids are expensive and require rigorous recycling protocols .
Solvent-Free Acetylation with Novel Catalysts
To minimize solvent waste, solvent-free methods have been developed using solid or mild acidic catalysts. For instance, cotton cellulose was acetylated with acetic anhydride in the presence of N-iodosuccinimide (NIS), achieving a DS of 2.84 under mild conditions . The reaction duration (1–5 hours) and catalyst loading (0.05–0.10 g per gram of cellulose) were critical variables, with Fourier-transform infrared (FT-IR) and Raman spectroscopy confirming ester bond formation . This approach eliminated the need for toxic solvents like pyridine, which are commonly used in traditional acetylation .
Another solvent-free variant utilized methane sulfonic acid as a catalyst for rice straw acetylation, achieving DS values near 3.0 with acetic acid, acetyl chloride, or IPA as acetyl donors . The process yielded over 70% this compound directly from raw rice straw, demonstrating the feasibility of using agricultural residues without prior cellulose isolation .
Heterogeneous Acetylation of Cellulosic Wastes
Heterogeneous methods, which involve solid-phase reactions, are widely used for industrial-scale production. A study on cocoa pod husk (CPH) cellulose employed a two-step process: alkaline extraction (5% NaOH) followed by acetylation with acetic anhydride and sulfuric acid, yielding this compound with a DS of 2.87 . The crystallinity index dropped from 38.4% in raw cellulose to a semi-crystalline structure post-acetylation, as shown by X-ray diffraction (XRD) . Similarly, acetylation of cotton linters and recycled newspaper using sulfuric acid and acetic anhydride produced this compound with solubility in chloroform-methanol mixtures .
These methods are cost-effective but often require harsh acids, leading to cellulose depolymerization. Patent US20060004192A1 addresses this by pre-cooling cellulose to −30°C before acylation, which reduces chain scission during sulfuric acid-catalyzed reactions .
Organocatalytic Transesterification
Organocatalytic routes offer a greener alternative to acid-catalyzed systems. For example, pretreated bagasse underwent transesterification with IPA in EmimOAc, yielding this compound and recoverable acetone as a byproduct . The reaction completed in 30 minutes at 80°C, highlighting the efficiency of organocatalysts in reducing energy input . Methane sulfonic acid also served as a low-toxicity catalyst in rice straw acetylation, enabling DS values of 2.98 with IPA .
Comparative Analysis of Preparation Methods
Table 1: Key Parameters of this compound Synthesis Methods
Chemical Reactions Analysis
Types of Reactions
Triacetylcellulose undergoes various chemical reactions, including:
Hydrolysis: The acetyl groups can be hydrolyzed back to hydroxyl groups in the presence of water and an acid or base catalyst.
Saponification: The acetyl groups can be removed using a sodium hydroxide solution, leaving a cellulose coating on the fibers.
Common Reagents and Conditions
Hydrolysis: Water, acid or base catalyst (e.g., sulfuric acid or sodium hydroxide).
Saponification: Sodium hydroxide solution.
Major Products Formed
Hydrolysis: Cellulose and acetic acid.
Saponification: Cellulose and sodium acetate.
Scientific Research Applications
Triacetylcellulose has a wide range of applications in scientific research and industry:
Membranes: Used in the fabrication of porous membranes for filtration and separation processes.
Optical Devices: Utilized in the production of polarized films for liquid crystal displays.
Biocomposites: Employed in the development of biodegradable and “all cellulose” biocomposite systems.
Textiles: Used in the production of wrinkle-resistant and shrink-resistant fabrics.
Mechanism of Action
The mechanism of action of cellulose triacetate primarily involves its physical and chemical properties. The acetylation of cellulose increases its solubility in organic solvents, allowing it to be processed into fibers and films. The high degree of acetylation also enhances its thermal stability and resistance to hydrolysis. The molecular targets and pathways involved include the interaction of the acetyl groups with various reagents and catalysts during chemical reactions .
Comparison with Similar Compounds
Chemical Structure and Acetylation Levels
The degree of acetylation is the primary differentiating factor among cellulose derivatives. Key compounds include:
Compound | Degree of Substitution (DS) | Hydroxyl Groups Acetylated | Chemical Formula (Repeat Unit) |
---|---|---|---|
Triacetylcellulose | ~2.93–3.0 | ≥92% | C₁₂H₁₆O₈ |
Cellulose Diacetate | ~2.4 | ~76% | C₁₂H₁₄O₇ |
Cellulose Tetracetate | ~3.5 | ~100% (hypothetical) | N/A |
Cellulose Pentacetate | ~4.0 | N/A | N/A |
- This compound : Full acetylation (DS ≈ 3.0) ensures minimal hydroxyl group availability, reducing hydrophilicity and enhancing resistance to hydrolysis .
- Cellulose Diacetate : Partial acetylation (DS ≈ 2.4) retains some hydroxyl groups, making it more soluble in acetone and less thermally stable than TAC .
Physical and Thermal Properties
Thermal Stability
TAC exhibits superior heat resistance compared to diacetate, with a glass transition temperature (Tg) exceeding 180°C, making it suitable for high-temperature applications like photographic films and LCD displays. Diacetate, in contrast, softens at ~120°C .
Solubility
- TAC : Insoluble in acetone; requires chlorinated solvents (e.g., dichloromethane) or ionic liquids for processing .
- Diacetate : Soluble in acetone, enabling easier fiber spinning and film casting .
Mechanical Properties
TAC films demonstrate higher tensile strength (80–120 MPa) and lower elongation at break (5–10%) compared to diacetate (tensile strength: 40–60 MPa; elongation: 15–25%) due to its rigid, fully acetylated structure .
Chromatography
TAC’s chiral recognition capability makes it a preferred stationary phase for enantiomer separation in liquid chromatography. For example, it achieves enantiomeric purities of 7–99% for aromatic carboxamides, outperforming diacetate in resolving axial chirality .
Sensor Membranes
TAC membranes immobilized with ligands (e.g., 5-(2′,4′-dimethylphenylazo)-6-hydroxypyrimidine-2,4-dione) enable selective detection of heavy metals like Hg²⁺ and Ni²⁺. Diacetate lacks the structural stability for such applications .
Environmental and Industrial Use
TAC-derived activated carbons (BET surface area: 28–252 m²/g) show promise in wastewater treatment, outperforming bamboo-based carbons in adsorption capacity .
Traditional vs. Novel Methods
- Traditional : Requires purified cellulose and multiple acetylation steps, leading to high costs and waste .
- Novel: Ionic liquid-mediated synthesis from unrefined biomass reduces steps and improves sustainability. This method achieves DS 2.93 with 55°C reaction conditions, enhancing cost efficiency .
Environmental Impact
TAC’s low biodegradability (due to high acetylation) poses disposal challenges. However, upcycling TAC waste into activated carbon mitigates this issue . Diacetate, being more biodegradable, is less persistent but also less durable.
Research Findings and Challenges
- Optical Sensors : TAC membranes achieve detection limits of 0.1 ppb for Hg²⁺, surpassing diacetate-based sensors .
- Chiral Separations : TAC columns resolve barriers to enantiomerization (87–120 kJ/mol) for carboxamides, a feat unmatched by diacetate .
- Challenges : TAC’s synthesis requires precise control of acetylation to avoid side products (e.g., PMVP copolymers), which can reduce purity to 55% TAC in some cases .
Biological Activity
Triacetylcellulose (TAC) is a derivative of cellulose that has garnered significant attention due to its unique properties and potential applications in various fields, including biomedicine, materials science, and environmental sustainability. This article delves into the biological activity of TAC, exploring its synthesis, characterization, and various applications supported by research findings and case studies.
1. Synthesis of this compound
TAC is synthesized through the acetylation of cellulose using acetic anhydride in the presence of a catalyst, typically sulfuric acid. The degree of substitution (DS) is a critical parameter that influences the properties and biological activity of TAC. Recent studies have demonstrated eco-friendly methods for producing TAC from lignocellulosic biomass, such as Palm Empty Fruit Bunch (EFB), using ionic liquids (ILs) for cellulose dissolution followed by acetylation. This method not only reduces environmental impact but also simplifies the synthesis process.
Table 1: Synthesis Parameters for this compound
Biomass Source | Ionic Liquid Used | Acetylation Temperature (°C) | Degree of Substitution |
---|---|---|---|
Palm Empty Fruit Bunch | BMIMCl | 55 | 2.93 |
Bagasse | EMIMAc | 60 | 2.85 |
Hybrid Poplar | BMIMCl | 55 | 3.00 |
2. Characterization Techniques
The characterization of TAC is typically performed using various spectroscopic methods:
- Nuclear Magnetic Resonance (NMR) : Used to determine the degree of substitution and confirm the structure of TAC.
- Fourier Transform Infrared Spectroscopy (FTIR) : Helps in identifying functional groups and confirming acetylation.
- Thermogravimetric Analysis (TGA) : Assesses thermal stability and degradation behavior.
3. Biological Activity
The biological activity of TAC can be attributed to its structural characteristics and chemical modifications. Key areas of research include:
3.1 Antimicrobial Properties
TAC has shown promising antimicrobial activity against various pathogens. A study highlighted the incorporation of copper complexes into TAC nanofibers, which exhibited significant antibacterial effects against strains like Pseudomonas aeruginosa and Staphylococcus epidermidis. This suggests potential applications in medical textiles to prevent infections.
3.2 Biocompatibility and Drug Delivery
TAC is considered biocompatible, making it suitable for drug delivery systems. Its film-forming ability allows for controlled release of therapeutic agents. Research has indicated that TAC films can encapsulate drugs effectively while maintaining their stability and release profiles.
Case Study: Drug Delivery System Using TAC
- Objective : To evaluate the drug release profile from TAC films.
- Method : Drugs were loaded into TAC films, and their release was monitored over time.
- Results : The study found a sustained release pattern over several days, indicating that TAC could serve as an effective matrix for drug delivery.
4. Environmental Applications
TAC's biodegradability presents opportunities for environmental applications. Its use in biodegradable packaging materials has been explored, aiming to reduce plastic waste while maintaining functionality.
Q & A
Basic Research Questions
Q. What are the key physicochemical properties of triacetylcellulose, and how do they influence experimental design in material science?
- Methodological Answer : this compound (CAS 9012-09-3) is characterized by variable molar mass and solubility in polar aprotic solvents (e.g., chloroform, dimethylacetamide). Its acetyl substitution degree (typically ~2.8–3.0) determines crystallinity and thermal stability. When designing experiments, researchers should prioritize characterizing substitution uniformity via H-NMR or FTIR to ensure batch consistency. For solubility studies, solvent purity and degassing protocols are critical to avoid artifacts in viscosity measurements .
Q. How can researchers optimize synthesis protocols for this compound to achieve reproducible acetylation levels?
- Methodological Answer : Reproducible synthesis requires strict control of reaction conditions:
- Temperature : Maintain 30–40°C to prevent cellulose chain degradation.
- Catalyst : Use sulfuric acid (0.5–1.0 wt%) for homogeneous acetylation, but neutralize post-reaction to avoid depolymerization.
- Characterization : Validate acetylation levels via titration (ASTM D871-96) or C-CP/MAS NMR for solid-state analysis. Cross-reference results with solubility tests in dichloromethane/methanol mixtures to confirm substitution homogeneity .
Advanced Research Questions
Q. How can contradictory data on this compound’s optical properties be resolved in spectroscopic studies?
- Methodological Answer : Contradictions often arise from residual solvent traces or crystallinity variations. To mitigate:
- Sample Preparation : Use vacuum drying (48 hours, 60°C) to eliminate solvent interference.
- Triangulation : Combine UV-Vis spectroscopy, XRD (to assess crystallinity), and polarized light microscopy. For example, Ensafi et al. (2011) demonstrated that immobilized 4-hydroxysalophen on this compound membranes requires rigorous solvent removal to achieve consistent lanthanum ion detection limits (0.1–10 μM) .
- Statistical Validation : Apply ANOVA to compare batch-to-batch variability, with p < 0.05 indicating significant differences .
Q. What advanced functionalization strategies enhance this compound’s applicability in membrane technology?
- Methodological Answer : Covalent functionalization (e.g., grafting sulfonic groups for proton exchange membranes) requires:
- Pre-activation : Treat membranes with plasma or alkali to generate reactive hydroxyl sites.
- Reagent Selection : Use epichlorohydrin or glycidyl methacrylate as crosslinkers, optimizing molar ratios via response surface methodology (RSM).
- Performance Testing : Evaluate ion-exchange capacity (IEC) and mechanical stability under simulated operational conditions (e.g., 80°C, 95% humidity). Havel et al. (1994) highlighted the role of flow injection analysis (FIA) for real-time monitoring of functionalized membranes in rare earth element separation .
Q. How can researchers identify literature gaps in this compound applications for sustainable material development?
- Methodological Answer : Conduct a systematic review using PICOT framework:
- Population : Focus on "biopolymer composites" or "cellulose derivatives."
- Intervention : Identify understudied functionalization methods (e.g., enzymatic acetylation).
- Comparison : Benchmark against cellulose acetate or nanocellulose.
- Outcome : Prioritize gaps in biodegradability metrics (e.g., ASTM D6400) or lifecycle analysis (LCA). Tools like VOSviewer can map keyword co-occurrence in Scopus/Web of Science datasets to visualize under-researched areas .
Q. Data Analysis & Reporting Standards
Q. What are the best practices for presenting this compound characterization data in peer-reviewed journals?
- Methodological Answer : Follow Beilstein Journal guidelines:
- Synthesis Data : Report yield, substitution degree, and purity (≥95% by HPLC). Use tables for batch comparisons (e.g., Table 1).
- Spectra : Include baseline-corrected FTIR peaks (e.g., 1745 cm for acetyl groups) with resolution ≥4 cm.
- Reproducibility : Provide standard deviations for ≥3 replicates. Supplementary materials should include raw NMR/TGA files in .cdf format .
Q. How should researchers address limitations in this compound-based experimental models?
- Methodological Answer : Explicitly discuss:
- Material Limitations : Hydrophobicity-induced fouling in filtration membranes.
- Method Constraints : Artifacts in DSC measurements due to moisture absorption.
- Mitigation Strategies : Pre-treatment protocols (e.g., silanization for surface modification) or hybrid composite designs (e.g., blending with polyvinyl alcohol). Reflective sections in manuscripts should align with IB Extended Essay criteria, emphasizing critical self-evaluation .
Q. Table: Key Properties of this compound
Properties
IUPAC Name |
[(2R,3R,4S,5R,6S)-4,5-diacetyloxy-3-[(2S,3R,4S,5R,6R)-3,4,5-triacetyloxy-6-(acetyloxymethyl)oxan-2-yl]oxy-6-[(2R,3R,4S,5R,6S)-4,5,6-triacetyloxy-2-(acetyloxymethyl)oxan-3-yl]oxyoxan-2-yl]methyl acetate | |
---|---|---|
Source | PubChem | |
URL | https://pubchem.ncbi.nlm.nih.gov | |
Description | Data deposited in or computed by PubChem | |
InChI |
InChI=1S/C40H54O27/c1-15(41)52-12-26-29(55-18(4)44)32(56-19(5)45)36(60-23(9)49)39(64-26)67-31-28(14-54-17(3)43)65-40(37(61-24(10)50)34(31)58-21(7)47)66-30-27(13-53-16(2)42)63-38(62-25(11)51)35(59-22(8)48)33(30)57-20(6)46/h26-40H,12-14H2,1-11H3/t26-,27-,28-,29-,30-,31-,32+,33+,34+,35-,36-,37-,38-,39+,40+/m1/s1 | |
Source | PubChem | |
URL | https://pubchem.ncbi.nlm.nih.gov | |
Description | Data deposited in or computed by PubChem | |
InChI Key |
NNLVGZFZQQXQNW-ADJNRHBOSA-N | |
Source | PubChem | |
URL | https://pubchem.ncbi.nlm.nih.gov | |
Description | Data deposited in or computed by PubChem | |
Canonical SMILES |
CC(=O)OCC1C(C(C(C(O1)OC2C(OC(C(C2OC(=O)C)OC(=O)C)OC(=O)C)COC(=O)C)OC(=O)C)OC(=O)C)OC3C(C(C(C(O3)COC(=O)C)OC(=O)C)OC(=O)C)OC(=O)C | |
Source | PubChem | |
URL | https://pubchem.ncbi.nlm.nih.gov | |
Description | Data deposited in or computed by PubChem | |
Isomeric SMILES |
CC(=O)OC[C@@H]1[C@H]([C@@H]([C@H]([C@@H](O1)O[C@@H]2[C@H](O[C@H]([C@@H]([C@H]2OC(=O)C)OC(=O)C)OC(=O)C)COC(=O)C)OC(=O)C)OC(=O)C)O[C@H]3[C@@H]([C@H]([C@@H]([C@H](O3)COC(=O)C)OC(=O)C)OC(=O)C)OC(=O)C | |
Source | PubChem | |
URL | https://pubchem.ncbi.nlm.nih.gov | |
Description | Data deposited in or computed by PubChem | |
Molecular Formula |
C40H54O27 | |
Source | PubChem | |
URL | https://pubchem.ncbi.nlm.nih.gov | |
Description | Data deposited in or computed by PubChem | |
Molecular Weight |
966.8 g/mol | |
Source | PubChem | |
URL | https://pubchem.ncbi.nlm.nih.gov | |
Description | Data deposited in or computed by PubChem | |
CAS No. |
9012-09-3 | |
Record name | Cellulose, triacetate | |
Source | EPA Chemicals under the TSCA | |
URL | https://www.epa.gov/chemicals-under-tsca | |
Description | EPA Chemicals under the Toxic Substances Control Act (TSCA) collection contains information on chemicals and their regulations under TSCA, including non-confidential content from the TSCA Chemical Substance Inventory and Chemical Data Reporting. | |
Record name | Cellulose, triacetate | |
Source | European Chemicals Agency (ECHA) | |
URL | https://echa.europa.eu/substance-information/-/substanceinfo/100.115.198 | |
Description | The European Chemicals Agency (ECHA) is an agency of the European Union which is the driving force among regulatory authorities in implementing the EU's groundbreaking chemicals legislation for the benefit of human health and the environment as well as for innovation and competitiveness. | |
Explanation | Use of the information, documents and data from the ECHA website is subject to the terms and conditions of this Legal Notice, and subject to other binding limitations provided for under applicable law, the information, documents and data made available on the ECHA website may be reproduced, distributed and/or used, totally or in part, for non-commercial purposes provided that ECHA is acknowledged as the source: "Source: European Chemicals Agency, http://echa.europa.eu/". Such acknowledgement must be included in each copy of the material. ECHA permits and encourages organisations and individuals to create links to the ECHA website under the following cumulative conditions: Links can only be made to webpages that provide a link to the Legal Notice page. | |
Record name | Cellulose triacetate | |
Source | Human Metabolome Database (HMDB) | |
URL | http://www.hmdb.ca/metabolites/HMDB0032198 | |
Description | The Human Metabolome Database (HMDB) is a freely available electronic database containing detailed information about small molecule metabolites found in the human body. | |
Explanation | HMDB is offered to the public as a freely available resource. Use and re-distribution of the data, in whole or in part, for commercial purposes requires explicit permission of the authors and explicit acknowledgment of the source material (HMDB) and the original publication (see the HMDB citing page). We ask that users who download significant portions of the database cite the HMDB paper in any resulting publications. | |
Disclaimer and Information on In-Vitro Research Products
Please be aware that all articles and product information presented on BenchChem are intended solely for informational purposes. The products available for purchase on BenchChem are specifically designed for in-vitro studies, which are conducted outside of living organisms. In-vitro studies, derived from the Latin term "in glass," involve experiments performed in controlled laboratory settings using cells or tissues. It is important to note that these products are not categorized as medicines or drugs, and they have not received approval from the FDA for the prevention, treatment, or cure of any medical condition, ailment, or disease. We must emphasize that any form of bodily introduction of these products into humans or animals is strictly prohibited by law. It is essential to adhere to these guidelines to ensure compliance with legal and ethical standards in research and experimentation.