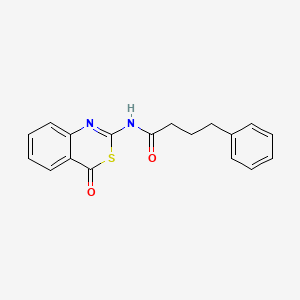
CAY10680
Overview
Description
CAY10680 is a benzothiazinone derivative with dual inhibitory activity against monoamine oxidase B (MAO-B) and adenosine A2A receptors. According to pharmacological data from , it exhibits:
- MAO-B inhibition: IC₅₀ = 34.9 nM (human)
- A2A receptor antagonism: Kᵢ = 39.5 nM (human)
- Selectivity: Minimal activity against other adenosine receptor subtypes (Kᵢ > 1 μM) and MAO-A (IC₅₀ ≥ 10 μM).
In cellular assays, this compound abolishes cAMP accumulation in CHO cells transfected with A2A receptors at concentrations of 1–20 μM, demonstrating functional antagonism .
Preparation Methods
Chemical Synthesis of CAY10680
The synthesis of this compound (CAS 1439488-21-7) revolves around constructing its benzothiazinone core, a heterocyclic structure fused with a substituted phenyl group. While proprietary details of the industrial synthesis remain undisclosed, retrosynthetic analysis and available data suggest a multi-step pathway involving cyclization and functional group transformations .
Core Benzothiazinone Formation
The benzothiazinone scaffold is synthesized via condensation of a thiourea derivative with a carbonyl-containing intermediate. The canonical SMILES string indicates the presence of a ketone group adjacent to a thiazine ring, which likely forms through intramolecular cyclization . Computational modeling of the reaction mechanism suggests that a thiourea precursor reacts with a brominated aromatic compound under basic conditions to facilitate ring closure .
Substitution and Functionalization
The final structure incorporates a butyl chain linked to a secondary amide group, introduced via nucleophilic acyl substitution. InvivoChem’s technical notes emphasize the use of DMSO as a reaction solvent due to its ability to stabilize intermediates during amide bond formation . Post-synthetic purification typically involves column chromatography with a silica gel stationary phase and a gradient elution system to isolate this compound with >98% purity .
Formulation and Solubility Optimization
This compound’s limited aqueous solubility necessitates careful solvent selection for experimental applications. Data from GlpBio and InvivoChem provide critical insights into formulation strategies .
Stock Solution Preparation
For in vitro studies, this compound is dissolved in dimethyl sulfoxide (DMSO) at concentrations up to 20 mg/mL, achieving a molarity of 61.65 mM (Table 1) . InvivoChem protocols recommend sequential dilution with corn oil or PEG300 for in vivo administration to ensure colloidal stability .
Table 1: Solvent Compatibility and Stock Solution Parameters
Parameter | DMSO | DMF | Corn Oil |
---|---|---|---|
Solubility (mg/mL) | 15 | 20 | 0.5 |
Recommended Storage | -20°C | -20°C | 4°C |
Stability Duration | 6 months | 6 months | 2 weeks |
In Vivo Formulation Protocol
A standardized protocol for rodent studies involves:
-
Dissolving 5 mg of this compound in 327 µL DMSO to create a 15.3 mg/mL stock .
-
Mixing the DMSO solution with 1,635 µL PEG300 and 327 µL Tween 80.
-
Diluting the mixture with 3,270 µL ddH₂O to achieve a final concentration of 1 mg/mL .
This formulation ensures bioavailability while minimizing solvent toxicity, critical for long-term neurodegenerative studies .
Analytical Characterization
Quality control of this compound relies on advanced spectroscopic and chromatographic techniques to verify purity and structural integrity.
High-Performance Liquid Chromatography (HPLC)
Reverse-phase HPLC with a C18 column and UV detection at 254 nm is employed to assess purity. GlpBio reports a retention time of 8.2 minutes under isocratic conditions (70:30 acetonitrile:water), with a single peak confirming homogeneity .
Mass Spectrometry
Electrospray ionization mass spectrometry (ESI-MS) correlates with the theoretical molecular weight of 324.40 g/mol, showing a predominant ion at m/z 325.41 . Minor impurities (<2%) are attributed to residual solvents or des-thio byproducts.
Chemical Reactions Analysis
Types of Reactions
CAY10680 undergoes several types of chemical reactions, including:
Oxidation: The compound can be oxidized to form sulfoxides or sulfones.
Reduction: Reduction reactions can convert the carbonyl groups to alcohols.
Substitution: The aromatic ring can undergo electrophilic substitution reactions.
Common Reagents and Conditions
Oxidation: Common oxidizing agents include hydrogen peroxide and m-chloroperbenzoic acid.
Reduction: Reducing agents such as lithium aluminum hydride or sodium borohydride are typically used.
Substitution: Electrophilic substitution reactions often involve reagents like bromine or nitric acid under acidic conditions.
Major Products
The major products formed from these reactions include sulfoxides, sulfones, alcohols, and various substituted derivatives of the benzothiazinone ring.
Scientific Research Applications
CAY10680 has a wide range of applications in scientific research:
Chemistry: Used as a model compound to study dual inhibition mechanisms.
Biology: Investigated for its effects on cellular signaling pathways involving adenosine A2A receptors.
Industry: Utilized in the development of new pharmacological agents targeting neurodegenerative disorders.
Mechanism of Action
CAY10680 exerts its effects by selectively inhibiting monoamine oxidase B and adenosine A2A receptors. Monoamine oxidase B is an enzyme involved in the catabolism of dopamine, and its inhibition leads to increased dopamine levels in the brain. Adenosine A2A receptors are involved in modulating neurotransmitter release, and their inhibition enhances dopamine receptor function. This dual action makes this compound a promising candidate for the treatment of Parkinson’s disease .
Comparison with Similar Compounds
Structural and Functional Similarity
While the provided evidence lacks direct structural analogs of CAY10680, several compounds share partial functional overlap:
Mechanistic Advantages
- Reduced Side Effects: Its selectivity for MAO-B over MAO-A minimizes dietary tyramine interactions (a common issue with non-selective MAO inhibitors) .
Key Pharmacological Data
Parameter | Value | Source |
---|---|---|
MAO-B IC₅₀ | 34.9 nM | |
A2A Kᵢ | 39.5 nM | |
cAMP Inhibition (CHO) | Complete at 1–20 μM | |
Solubility (Water) | 0.751 mg/mL | * |
Clinical Implications
- Parkinson’s Disease : Dual inhibition may synergistically enhance motor function and neuroprotection.
- Cognitive Disorders : A2A receptor blockade could improve cognitive deficits, as seen in preclinical models .
Biological Activity
CAY10680 is a compound that has garnered attention in the field of pharmacology due to its diverse biological activities. This article provides a comprehensive overview of the biological activity of this compound, including its mechanisms of action, effects on various biological systems, and relevant case studies.
Overview of this compound
This compound is a selective inhibitor of the enzyme phosphodiesterase 4 (PDE4), which plays a crucial role in various cellular signaling pathways by regulating the levels of cyclic adenosine monophosphate (cAMP). By inhibiting PDE4, this compound can elevate cAMP levels, leading to various downstream effects that are beneficial in treating inflammatory diseases and other conditions.
- Inhibition of Inflammatory Responses : this compound has been shown to reduce the production of pro-inflammatory cytokines such as TNF-α and IL-6. This effect is primarily mediated through the modulation of cAMP signaling pathways, which inhibit nuclear factor kappa B (NF-κB) activation.
- Neuroprotective Effects : Research indicates that this compound may exert neuroprotective effects by enhancing cAMP signaling in neuronal cells. This can lead to improved neuronal survival and function, particularly in models of neurodegenerative diseases.
- Anti-Proliferative Activity : Studies have demonstrated that this compound can inhibit cell proliferation in various cancer cell lines. The compound induces apoptosis through the activation of caspase pathways, demonstrating its potential as an anti-cancer agent.
Biological Activity Data
The following table summarizes key biological activities and their corresponding effects observed with this compound:
Biological Activity | Effect | Mechanism |
---|---|---|
Anti-inflammatory | Decreased cytokine production | PDE4 inhibition leading to increased cAMP levels |
Neuroprotection | Enhanced neuronal survival | Modulation of cAMP signaling |
Anti-proliferative | Induction of apoptosis in cancer cells | Activation of caspase pathways |
Antioxidant | Reduction of oxidative stress | Upregulation of antioxidant enzymes |
Case Studies and Research Findings
- Inflammatory Disease Models : In animal models of asthma, treatment with this compound resulted in significant reductions in airway inflammation and hyperresponsiveness. This suggests its potential utility in managing chronic inflammatory conditions.
- Cancer Research : In vitro studies on breast cancer cell lines revealed that this compound could inhibit cell growth and induce apoptosis. The compound's ability to modulate cAMP levels was linked to alterations in cell cycle progression.
- Neurodegenerative Disease Studies : A study investigating the effects of this compound on neuroblastoma cells showed that it could protect against oxidative stress-induced damage, indicating its potential role in neuroprotection.
Q & A
Basic Research Questions
Q. What are the key considerations for synthesizing CAY10680 with high purity for reproducible pharmacological studies?
- Methodological Answer : Synthesis protocols should prioritize controlled reaction conditions (e.g., inert atmosphere, precise temperature gradients) and employ purification techniques like preparative HPLC or column chromatography. Characterization via NMR (¹H/¹³C), high-resolution mass spectrometry (HRMS), and elemental analysis is critical for verifying purity . Batch-to-batch variability should be minimized by documenting solvent sources, catalyst purity, and reaction kinetics.
Q. How can researchers validate this compound’s proposed mechanism of action in vitro?
- Methodological Answer : Use a combination of molecular docking simulations (to predict target binding) and functional assays (e.g., enzyme inhibition kinetics, cellular viability assays). Include positive/negative controls (e.g., known inhibitors) and orthogonal validation methods like siRNA-mediated gene silencing to confirm specificity .
Q. What spectroscopic and chromatographic methods are recommended for characterizing this compound’s stability under varying storage conditions?
- Methodological Answer : Conduct accelerated stability studies using HPLC-UV to monitor degradation products. Pair with FTIR or Raman spectroscopy to detect structural changes. Store samples under controlled humidity/temperature and compare degradation rates using Arrhenius equation modeling .
Advanced Research Questions
Q. How should researchers design dose-response experiments to address conflicting data on this compound’s efficacy across different cell lines?
- Methodological Answer : Optimize assay parameters (e.g., cell passage number, seeding density, incubation time) to minimize variability. Use a multi-parametric approach:
- Dose range : Test logarithmic dilutions (e.g., 1 nM–100 µM).
- Normalization : Include housekeeping genes/proteins (e.g., GAPDH) for qPCR/Western blot data.
- Statistical rigor : Apply ANOVA with post-hoc tests (e.g., Tukey’s) to compare IC₅₀ values across cell lines. Address outliers via Grubbs’ test .
Q. What strategies can resolve contradictions between in vitro and in vivo pharmacokinetic data for this compound?
- Methodological Answer : Investigate factors like bioavailability (e.g., plasma protein binding via equilibrium dialysis) and metabolic stability (e.g., liver microsome assays). Use physiologically based pharmacokinetic (PBPK) modeling to reconcile discrepancies. Cross-validate in vivo results with LC-MS/MS quantification of parent compound/metabolites in target tissues .
Q. How can researchers optimize experimental workflows to study this compound’s off-target effects systematically?
- Methodological Answer : Employ high-content screening (HCS) with fluorescence-based multiplex assays (e.g., apoptosis, oxidative stress markers). Combine with chemoproteomics (e.g., activity-based protein profiling) to identify unintended targets. Validate hits via CRISPR-Cas9 knockout models and dose-dependent rescue experiments .
Q. What statistical approaches are recommended for analyzing time-dependent antagonism observed in this compound’s receptor binding assays?
- Methodological Answer : Use non-linear regression to fit time-course data to models like the two-step irreversible binding equation. Compare Akaike Information Criterion (AIC) values to select the best-fit model. Apply bootstrapping to estimate confidence intervals for kinetic parameters (e.g., kₒₙ, kₒff) .
Q. How should researchers integrate multi-omics data to elucidate this compound’s polypharmacology?
- Methodological Answer : Perform transcriptomic (RNA-seq) and proteomic (TMT/MS) profiling post-treatment. Use pathway enrichment tools (e.g., GSEA, MetaboAnalyst) to identify perturbed networks. Cross-reference with ChIP-seq or ATAC-seq data to assess epigenetic effects. Validate via CRISPRi/a or small-molecule epistasis experiments .
Methodological and Reproducibility-Focused Questions
Q. What steps ensure reproducibility in this compound’s in vivo efficacy studies?
- Methodological Answer : Standardize animal models (e.g., genetic background, diet, circadian cycles). Use randomization/blinding to minimize bias. Report compliance with ARRIVE guidelines, including sample size calculations and exclusion criteria. Deposit raw data in public repositories (e.g., Zenodo) for independent validation .
Q. How can researchers address batch variability in commercial this compound samples for comparative studies?
- Methodological Answer : Source compounds from ≥3 independent suppliers. Characterize each batch via orthogonal methods (e.g., DSC for crystallinity, XRPD for polymorphism). Include batch-specific controls in experiments and perform principal component analysis (PCA) on datasets to identify batch-driven outliers .
Properties
IUPAC Name |
N-(4-oxo-3,1-benzothiazin-2-yl)-4-phenylbutanamide | |
---|---|---|
Source | PubChem | |
URL | https://pubchem.ncbi.nlm.nih.gov | |
Description | Data deposited in or computed by PubChem | |
InChI |
InChI=1S/C18H16N2O2S/c21-16(12-6-9-13-7-2-1-3-8-13)20-18-19-15-11-5-4-10-14(15)17(22)23-18/h1-5,7-8,10-11H,6,9,12H2,(H,19,20,21) | |
Source | PubChem | |
URL | https://pubchem.ncbi.nlm.nih.gov | |
Description | Data deposited in or computed by PubChem | |
InChI Key |
QMBOZLGNPMVERZ-UHFFFAOYSA-N | |
Source | PubChem | |
URL | https://pubchem.ncbi.nlm.nih.gov | |
Description | Data deposited in or computed by PubChem | |
Canonical SMILES |
C1=CC=C(C=C1)CCCC(=O)NC2=NC3=CC=CC=C3C(=O)S2 | |
Source | PubChem | |
URL | https://pubchem.ncbi.nlm.nih.gov | |
Description | Data deposited in or computed by PubChem | |
Molecular Formula |
C18H16N2O2S | |
Source | PubChem | |
URL | https://pubchem.ncbi.nlm.nih.gov | |
Description | Data deposited in or computed by PubChem | |
Molecular Weight |
324.4 g/mol | |
Source | PubChem | |
URL | https://pubchem.ncbi.nlm.nih.gov | |
Description | Data deposited in or computed by PubChem | |
Retrosynthesis Analysis
AI-Powered Synthesis Planning: Our tool employs the Template_relevance Pistachio, Template_relevance Bkms_metabolic, Template_relevance Pistachio_ringbreaker, Template_relevance Reaxys, Template_relevance Reaxys_biocatalysis model, leveraging a vast database of chemical reactions to predict feasible synthetic routes.
One-Step Synthesis Focus: Specifically designed for one-step synthesis, it provides concise and direct routes for your target compounds, streamlining the synthesis process.
Accurate Predictions: Utilizing the extensive PISTACHIO, BKMS_METABOLIC, PISTACHIO_RINGBREAKER, REAXYS, REAXYS_BIOCATALYSIS database, our tool offers high-accuracy predictions, reflecting the latest in chemical research and data.
Strategy Settings
Precursor scoring | Relevance Heuristic |
---|---|
Min. plausibility | 0.01 |
Model | Template_relevance |
Template Set | Pistachio/Bkms_metabolic/Pistachio_ringbreaker/Reaxys/Reaxys_biocatalysis |
Top-N result to add to graph | 6 |
Feasible Synthetic Routes
Disclaimer and Information on In-Vitro Research Products
Please be aware that all articles and product information presented on BenchChem are intended solely for informational purposes. The products available for purchase on BenchChem are specifically designed for in-vitro studies, which are conducted outside of living organisms. In-vitro studies, derived from the Latin term "in glass," involve experiments performed in controlled laboratory settings using cells or tissues. It is important to note that these products are not categorized as medicines or drugs, and they have not received approval from the FDA for the prevention, treatment, or cure of any medical condition, ailment, or disease. We must emphasize that any form of bodily introduction of these products into humans or animals is strictly prohibited by law. It is essential to adhere to these guidelines to ensure compliance with legal and ethical standards in research and experimentation.