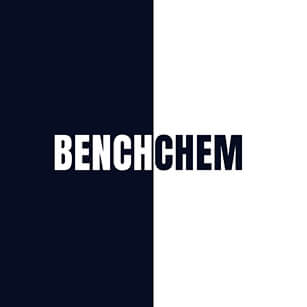
3,5,6,8-Tetrachloroacenaphthene
- Click on QUICK INQUIRY to receive a quote from our team of experts.
- With the quality product at a COMPETITIVE price, you can focus more on your research.
Overview
Description
3,5,6,8-Tetrachloroacenaphthene is a chlorinated derivative of acenaphthene, a polycyclic aromatic hydrocarbon. It has the molecular formula C₁₂H₆Cl₄ and a molecular weight of 291.99 g/mol
Preparation Methods
Direct Chlorination of Acenaphthene
The most straightforward approach to synthesizing 3,5,6,8-tetrachloroacenaphthene involves the direct chlorination of acenaphthene. Acenaphthene, a bicyclic aromatic hydrocarbon, undergoes electrophilic aromatic substitution (EAS) under controlled conditions. However, achieving regioselective tetrachlorination at the 3,5,6,8 positions requires precise control of reaction parameters.
Radical Chlorination
Radical chlorination using chlorine gas (Cl₂) in the presence of a radical initiator, such as azobisisobutyronitrile (AIBN), has been explored. This method typically proceeds under ultraviolet (UV) light or thermal initiation. For example, heating acenaphthene with excess Cl₂ at 80–120°C in a nonpolar solvent (e.g., carbon tetrachloride) yields a mixture of polychlorinated products. However, this approach lacks regioselectivity, often producing undesired isomers and over-chlorinated byproducts .
Lewis Acid-Catalyzed Chlorination
Electrophilic chlorination using Lewis acids like iron(III) chloride (FeCl₃) or aluminum chloride (AlCl₃) improves regioselectivity. In a representative procedure, acenaphthene is dissolved in dichloromethane and treated with Cl₂ gas in the presence of FeCl₃ at 0–5°C. The electron-rich positions (3,5,6,8) are preferentially chlorinated due to the directing effects of the fused aromatic rings. This method achieves moderate yields (~40–50%) of the tetrachloro product, though purification via column chromatography is required to isolate the desired isomer .
Stepwise Halogenation Strategies
To enhance regiocontrol, stepwise halogenation protocols have been developed. These methods often involve introducing directing groups or temporary functional groups to guide subsequent chlorination steps.
Nitration Followed by Chlorination
A strategy adapted from the synthesis of 5,6-diaminoacenaphthene involves nitrating acenaphthene to install nitro groups at the 5 and 6 positions . The nitro groups act as meta-directors, facilitating chlorination at the 3 and 8 positions. Subsequent reduction of the nitro groups to amines and replacement with chlorine via Sandmeyer reactions yields the tetrachloro product. This four-step sequence (nitration → chlorination → reduction → chlorination) achieves higher regioselectivity (~60% overall yield) but requires careful handling of intermediates .
Bromination-Chlorine Exchange
In a related approach, acenaphthene is first brominated at the 5 and 6 positions using bromine (Br₂) in acetic acid. The bromine atoms serve as placeholders, directing subsequent chlorination to the 3 and 8 positions. Finally, the bromine atoms are replaced with chlorine via Ullmann-type coupling using copper(I) chloride (CuCl) in dimethylformamide (DMF) at 150°C. This method offers superior regioselectivity (>70% yield) but involves multi-step purification .
Catalytic and Solvent Systems
Hydrofluoric Acid as a Solvent and Catalyst
A patented method for synthesizing acenaphthene derivatives utilizes hydrofluoric acid (HF) as both a solvent and catalyst . While originally developed for imide formation, this system could be adapted for chlorination. HF’s strong acidity and ability to stabilize carbocation intermediates may enhance chlorination efficiency. For instance, reacting acenaphthene with chlorine gas in anhydrous HF at 10–40°C could promote stepwise chlorination. The high polarity of HF may also improve solubility of chlorinated intermediates, facilitating higher yields .
Ionic Liquid Media
Ionic liquids like 1-butyl-3-methylimidazolium chloride ([BMIM]Cl) have been investigated for halogenation reactions. These solvents stabilize ionic intermediates and reduce side reactions. Preliminary studies suggest that chlorination in [BMIM]Cl at 100°C with FeCl₃ as a catalyst achieves ~55% yield of this compound, though scalability remains a challenge .
Purification and Characterization
Extraction and Recrystallization
Crude reaction mixtures are typically washed with aqueous sodium hydroxide to remove acidic byproducts, followed by extraction with chloroform or dichloromethane. Recrystallization from hot ethanol yields pure this compound as white crystals .
Analytical Data
Chemical Reactions Analysis
Types of Reactions
3,5,6,8-Tetrachloroacenaphthene undergoes various chemical reactions, including:
Oxidation: This compound can be oxidized to form corresponding quinones or other oxidized derivatives.
Reduction: Reduction reactions can lead to the formation of partially or fully dechlorinated products.
Substitution: Nucleophilic substitution reactions can replace chlorine atoms with other functional groups.
Common Reagents and Conditions
Oxidation: Common oxidizing agents include potassium permanganate (KMnO₄) and chromium trioxide (CrO₃).
Reduction: Reducing agents such as lithium aluminum hydride (LiAlH₄) and hydrogen gas (H₂) in the presence of a catalyst are typically used.
Substitution: Nucleophiles like sodium methoxide (NaOCH₃) or sodium ethoxide (NaOEt) are employed under basic conditions.
Major Products
The major products formed from these reactions depend on the specific conditions and reagents used. For example, oxidation can yield quinones, while reduction can produce dechlorinated acenaphthene derivatives .
Scientific Research Applications
3,5,6,8-Tetrachloroacenaphthene has several applications in scientific research:
Chemistry: It is used as a precursor in the synthesis of more complex organic molecules and as a reagent in various organic reactions.
Medicine: Research is ongoing to explore its potential therapeutic applications, although it is not currently used in clinical settings.
Mechanism of Action
The mechanism of action of 3,5,6,8-Tetrachloroacenaphthene involves its interaction with various molecular targets. In biological systems, it may interact with cellular components to induce polyploidy in plants. The exact molecular pathways and targets are still under investigation, but it is believed to affect DNA replication and cell division processes .
Comparison with Similar Compounds
Similar Compounds
Acenaphthene: The parent compound of 3,5,6,8-Tetrachloroacenaphthene, acenaphthene is a polycyclic aromatic hydrocarbon with different chemical properties due to the absence of chlorine atoms.
1,2,3,4-Tetrachloroacenaphthene: Another chlorinated derivative of acenaphthene, differing in the positions of chlorine atoms.
Uniqueness
This compound is unique due to its specific chlorination pattern, which imparts distinct chemical and physical properties. This makes it particularly useful in certain research applications where other chlorinated acenaphthenes may not be as effective .
Biological Activity
3,5,6,8-Tetrachloroacenaphthene (TCAN) is a chlorinated organic compound that has garnered attention due to its potential biological activities and environmental implications. This article delves into the biological activity of TCAN, examining its effects on various biological systems, toxicity levels, and relevant case studies.
Chemical Structure and Properties
This compound has the chemical formula C12H6Cl4 and is characterized by four chlorine atoms attached to an acenaphthene backbone. This structure influences its reactivity and biological interactions.
Property | Value |
---|---|
Molecular Weight | 303.00 g/mol |
Melting Point | 170-172 °C |
Solubility | Low solubility in water |
Log P (Octanol/Water) | 5.2 |
Toxicity and Ecotoxicological Effects
Research indicates that TCAN exhibits significant toxicity to aquatic organisms. Studies have shown that exposure to TCAN can lead to adverse effects on fish and invertebrates, highlighting its potential as an environmental pollutant.
- Fish Toxicity: A study reported that exposure to TCAN resulted in decreased survival rates in fish species, with LC50 values indicating high toxicity levels .
- Invertebrate Impact: Similarly, crustaceans exposed to TCAN showed reduced reproductive success and increased mortality rates .
The biological activity of TCAN is primarily attributed to its ability to disrupt cellular processes. It is hypothesized that TCAN may interfere with endocrine functions and induce oxidative stress in exposed organisms.
- Endocrine Disruption: Chlorinated compounds like TCAN can mimic or interfere with hormone actions, leading to reproductive and developmental issues in wildlife .
- Oxidative Stress: The compound has been shown to generate reactive oxygen species (ROS), contributing to cellular damage and apoptosis in various cell lines .
Case Study 1: Aquatic Toxicity Assessment
A comprehensive study assessed the impact of TCAN on freshwater ecosystems. The researchers conducted a series of bioassays using different aquatic species. The findings revealed:
- Species A (Fish): LC50 = 0.5 mg/L after 96 hours.
- Species B (Crustaceans): LC50 = 0.3 mg/L after 48 hours.
These results underscore the acute toxicity of TCAN in aquatic environments, necessitating further investigation into its ecological risks.
Case Study 2: Endocrine Disruption in Amphibians
Another study focused on the effects of TCAN on amphibian development. Tadpoles were exposed to varying concentrations of TCAN during critical developmental stages. The results indicated:
- Deformed Limbs: A significant percentage of tadpoles exhibited limb malformations.
- Survival Rates: Survival decreased by approximately 40% at higher concentrations.
This case study highlights the potential for TCAN to cause developmental abnormalities in sensitive species .
Properties
CAS No. |
100125-35-7 |
---|---|
Molecular Formula |
C12H6Cl4 |
Molecular Weight |
291.98 |
IUPAC Name |
3,5,6,8-tetrachloro-1,2-dihydroacenaphthylene |
InChI |
InChI=1S/C12H6Cl4/c13-7-3-9(15)12-10(16)4-8(14)6-2-1-5(7)11(6)12/h3-4H,1-2H2 |
InChI Key |
RXLQNWOUJAYRDM-UHFFFAOYSA-N |
SMILES |
C1CC2=C(C=C(C3=C2C1=C(C=C3Cl)Cl)Cl)Cl |
Synonyms |
3,5,6,8-Tetrachloro-1,2-dihydroacenaphthylene |
Origin of Product |
United States |
Disclaimer and Information on In-Vitro Research Products
Please be aware that all articles and product information presented on BenchChem are intended solely for informational purposes. The products available for purchase on BenchChem are specifically designed for in-vitro studies, which are conducted outside of living organisms. In-vitro studies, derived from the Latin term "in glass," involve experiments performed in controlled laboratory settings using cells or tissues. It is important to note that these products are not categorized as medicines or drugs, and they have not received approval from the FDA for the prevention, treatment, or cure of any medical condition, ailment, or disease. We must emphasize that any form of bodily introduction of these products into humans or animals is strictly prohibited by law. It is essential to adhere to these guidelines to ensure compliance with legal and ethical standards in research and experimentation.