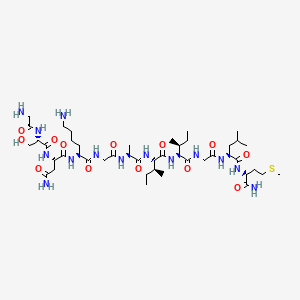
Amyloid beta-protein (25-35) amide
Overview
Description
Amyloid beta-protein (25-35) amide (Aβ25-35 amide) is an 11-residue peptide fragment derived from the amyloid beta-protein (Aβ), a key component of senile plaques in Alzheimer’s disease (AD). Its sequence (GSNKGAIIGLM-NH₂) corresponds to residues 25–35 of full-length Aβ, with a C-terminal amidation modification that enhances stability and aggregation propensity . Aβ25-35 amide retains the hydrophobic core of Aβ (residues 29–35), critical for self-assembly into β-sheet-rich fibrils . Unlike longer Aβ isoforms (e.g., Aβ1-42), Aβ25-35 amide lacks the N-terminal hydrophilic domain, simplifying its use in experimental models of amyloid toxicity .
Preparation Methods
Solid-Phase Peptide Synthesis (SPPS) of Aβ25–35 Amide
Boc/Bzl Strategy with Oxidized Methionine
The tert-butoxycarbonyl (Boc)/benzyl (Bzl) SPPS approach remains the gold standard for synthesizing Aβ25–35 amide. Kim et al. optimized this method by incorporating methionine sulfoxide (Met(O)-35) to mitigate aggregation during synthesis . The protocol involves:
-
Resin selection : Aminomethyl-functionalized resin (1.0 mmol/g loading) preloaded with Boc-Met(O)-PAM (phenylacetamidomethyl) ester.
-
Coupling conditions : 1.5 equivalents of Boc-protected amino acids activated with benzotriazol-1-yl-oxytripyrrolidinophosphonium hexafluorophosphate (BOP) in dimethylformamide (DMF)/dimethylsulfoxide (DMSO) (3:1 v/v) .
-
DMSO co-solvent : Reduces peptide chain aggregation by disrupting β-sheet formation, achieving coupling efficiencies >98% for hydrophobic residues (Ile28, Ile31) .
Aβ25–35 amide’s sequence (NH₂-Gly-Ser-Asn-Lys-Gly-Ala-Ile-Ile-Gly-Leu-Met-CONH₂ ) requires sequential coupling from Met35 to Gly25. The C-terminal amidation is achieved using a Rink amide resin, which introduces the CONH₂ group during cleavage .
Solution-Phase Amidation and Side-Chain Deprotection
Post-Synthesis Amidation Techniques
For non-resin-based approaches, solution-phase amidation employs carbodiimide reagents:
-
EDC/HOBt activation : 1-ethyl-3-(3-dimethylaminopropyl)carbodiimide (EDC) and hydroxybenzotriazole (HOBt) in DMF activate the C-terminal carboxyl group of Aβ25–35 .
-
Ammonolysis : Reaction with ammonium chloride yields the amide, with yields ranging from 65% to 78% depending on peptide concentration (0.1–0.5 mM) .
Global Deprotection Challenges
Bzl-protected side chains (e.g., Ser²⁶, Lys²⁸) require harsh acidic conditions for removal:
-
Hydrofluoric acid (HF) cleavage : Conducted at −10°C for 60 minutes, followed by ether precipitation .
-
By-product formation : Incomplete deprotection can yield truncated peptides (e.g., Aβ26–42), detectable via HPLC as secondary peaks at 214 nm .
Purification and Characterization
Reverse-Phase HPLC (RP-HPLC)
Crude Aβ25–35 amide is purified using:
-
Column : C18 stationary phase (5 µm, 250 × 4.6 mm).
-
Gradient : 10%–60% acetonitrile in 0.1% trifluoroacetic acid (TFA) over 45 minutes .
-
Critical impurities : Aβ25–35 with free C-terminal carboxylate (retention time ≈ 22.1 min vs. 24.3 min for amide) .
Mass Spectrometry Validation
Matrix-assisted laser desorption/ionization time-of-flight (MALDI-TOF) confirms molecular weight:
Aggregation Control and Fibril Formation
Solvent Pretreatment Protocols
Aβ25–35 amide’s aggregation state is modulated using:
-
Hexafluoroisopropanol (HFIP) : Dissolves preexisting fibrils (1 mg/mL, 30 min sonication), stabilizing α-helical monomers .
-
Phosphate-buffered saline (PBS) : Incubation at 37°C for 48 hours induces β-sheet-rich fibrils, verified by thioflavin-T fluorescence (λₑₓ = 450 nm, λₑₘ = 482 nm) .
Time-Dependent Conformational Shifts
Circular dichroism (CD) spectroscopy reveals:
Chemical Reactions Analysis
Types of Reactions: Amyloid beta-protein (25-35) amide undergoes various chemical reactions, including aggregation, oxidation, and interaction with lipid membranes. Aggregation leads to the formation of fibrils and oligomers, which are central to its neurotoxic effects .
Common Reagents and Conditions:
Aggregation: This process is influenced by factors such as pH, temperature, and the presence of metal ions.
Major Products Formed: The primary products formed from the aggregation of this compound are fibrils and oligomers. These structures are associated with the neurodegenerative effects observed in Alzheimer’s disease .
Scientific Research Applications
Neurobiology and Mechanistic Studies
Neurotoxicity and Aggregation Studies
Amyloid beta-protein (25-35) amide is often utilized as a model to study the aggregation mechanisms of amyloid peptides. Research indicates that this fragment retains the neurotoxic properties of its longer counterparts, making it a valuable tool for understanding Alzheimer’s disease pathology. The peptide can undergo conformational changes from a soluble form to aggregated fibrillary structures, which are crucial in the formation of amyloid plaques associated with Alzheimer's disease .
Oxidative Stress and Inflammation
Studies have shown that the aggregation state of amyloid beta-protein (25-35) can influence oxidative stress levels in neuronal environments. For instance, in vivo studies demonstrated that pre-aggregated forms of this peptide exacerbate neurodegenerative effects compared to soluble forms, highlighting its role in inflammatory responses within the brain .
Drug Design and Therapeutic Applications
Inhibition of Aggregation
The this compound is frequently used in drug discovery efforts aimed at inhibiting amyloid aggregation. Various natural and synthetic compounds have been tested for their ability to interfere with the self-assembly of this peptide. For example, compounds like curcumin and myricetin have shown promise in reducing aggregation through direct interaction with the peptide . Understanding these interactions can facilitate the rational design of more effective therapeutic agents targeting Alzheimer’s disease.
Biophysical Characterization for Drug Development
Biophysical techniques such as circular dichroism spectroscopy and Thioflavin T fluorescence spectroscopy are employed to monitor the structural transitions of amyloid beta-protein (25-35). These methods help elucidate the mechanisms by which potential inhibitors disrupt aggregation pathways . Such studies are critical for developing drugs that can effectively mitigate the toxic effects associated with amyloid aggregation.
Membrane Interaction Studies
Pore Formation and Ion Conductivity
Recent research has highlighted the ability of amyloid beta-protein (25-35) to form ion-conducting pores in lipid membranes. This property is linked to its neurotoxic effects, as it can disrupt cellular homeostasis by permeabilizing membranes . Understanding how this peptide interacts with lipid bilayers provides insights into its role in neuronal cell death and offers potential avenues for therapeutic intervention.
Case Studies and Experimental Findings
Mechanism of Action
Amyloid beta-protein (25-35) amide exerts its effects primarily through its ability to aggregate into toxic oligomers and fibrils. These aggregates disrupt cellular membranes, leading to increased permeability and cell death . The peptide interacts with various molecular targets, including lipid membranes and metal ions, which facilitate its aggregation and toxicity . Additionally, it can induce oxidative stress and inflammation, further contributing to neuronal damage .
Comparison with Similar Compounds
Key Properties :
- Molecular Weight : 1060.27 g/mol .
- Isoelectric Point : 10.1; net charge +1 at pH 7 .
- Solubility : 3.33 mg/mL in water; requires sonication for dissolution .
- Stability : Prone to aggregation; solutions are unstable and recommended for immediate use .
Aβ25-35 amide exhibits neurotoxicity via apoptotic pathways, mediated by oxidative stress at methionine-35 .
Comparison with Similar Amyloid Beta Fragments and Derivatives
Aggregation Propensity and Structural Dynamics
Key Findings :
- Aβ25-35 amide and Aβ22-35 share overlapping sequences but differ in N-terminal residues. Both form fibrils, though Aβ22-35 requires longer incubation .
- Aβ1-42 aggregates faster than Aβ1-40 due to additional hydrophobic residues (Ile-41, Ala-42) .
- Methionine-35 in Aβ25-35 amide and Aβ31-35 is critical for redox-mediated toxicity, with oxidation altering aggregation kinetics .
Toxicity Mechanisms
Key Findings :
- Aβ25-35 amide and Aβ31-35 induce apoptosis through methionine-35 redox cycling, generating hydrogen peroxide .
- Aβ1-42 toxicity involves pore formation in neuronal membranes and potentiation of long-term depression (LTD) in hippocampal regions .
- Aβ25-35 amide uniquely suppresses APP secretion, diverting APP toward amyloidogenic processing .
Key Findings :
Biological Activity
Amyloid beta-protein (25-35) amide, often referred to as Aβ(25-35), is a significant fragment of the amyloid beta peptide associated with Alzheimer's disease (AD). This peptide has garnered attention due to its biological activity, particularly its role in neurodegeneration and its potential as a therapeutic target. This article explores the conformations, aggregation properties, cytotoxicity, and mechanisms of action of Aβ(25-35), supported by case studies and research findings.
Overview of Aβ(25-35)
Aβ(25-35) is a hydrophobic fragment derived from the full-length amyloid beta peptides (Aβ(1-40) and Aβ(1-42)). It consists of 11 amino acids: NH2-Gly-Ser-Asn-Lys-Gly-Ala-Ile-Ile-Gly-Leu-Met-COOH. Despite being a shorter fragment, Aβ(25-35) retains significant biological properties, including toxicity and the ability to form aggregates that contribute to neurodegenerative processes in AD .
Conformational Properties
The conformational state of Aβ(25-35) is crucial for its biological activity. Studies indicate that Aβ(25-35) undergoes a transition from a soluble, unordered form to aggregated fibrillary β-sheet structures depending on environmental conditions such as pH and ionic strength. This transition is essential for understanding its aggregation kinetics and toxicity mechanisms .
Table 1: Conformational Changes of Aβ(25-35)
Environment | Conformation Type | Observations |
---|---|---|
Milli-Q Water | Unordered | Lower cytotoxicity; flat fibrils |
Phosphate Buffer | β-sheet | Higher cytotoxicity; twisted fibrils |
Aggregation and Toxicity
The aggregation state of Aβ(25-35) significantly influences its neurotoxic effects. In vivo studies have shown that pre-aggregated forms of Aβ(25-35) induce oxidative stress and inflammation in the brain, exacerbating neurodegenerative effects compared to soluble forms . The formation of small fibril-like aggregates leads to increased oxidative stress, which is a hallmark of AD pathology .
Case Study: In Vivo Effects
In an experimental model, administration of aggregated Aβ(25-35) resulted in notable increases in markers of oxidative stress within the brain tissue. This study highlighted the correlation between aggregate morphology and inflammatory neuronal response, suggesting that early-stage aggregates are particularly harmful .
Aβ(25-35) exerts its neurotoxic effects through various mechanisms:
- Membrane Permeabilization : Aβ(25-35) can form pores in lipid membranes, facilitating calcium ion transport. This pore-forming ability is attributed to its β-sheet conformation, which allows it to insert into membranes and disrupt cellular integrity .
- Oxidative Stress Induction : The peptide's aggregation leads to increased production of reactive oxygen species (ROS), contributing to cellular damage and apoptosis in neuronal cells .
- Inflammatory Response : Intracerebral injection of Aβ(25-35) triggers significant inflammatory responses, characterized by the activation of glial cells and release of pro-inflammatory cytokines .
Self-Assembly Studies
Research utilizing circular dichroism (CD) spectroscopy has demonstrated that Aβ(25-35) transitions from an unordered conformation to a β-sheet-rich structure under specific conditions. This self-assembly process is critical for understanding how inhibitors may disrupt toxic aggregate formation .
Cytotoxicity Assessment
In vitro studies have shown that increasing concentrations of Aβ(25-35) correlate with decreasing cell viability in neuronal cell lines. For instance, SH-SY5Y cells exposed to 50 μM concentrations exhibited only 59% viability after 48 hours .
Q & A
Basic Research Questions
Q. How should Aβ25-35 amide be prepared to ensure monomeric stability for in vitro neurotoxicity studies?
- Methodology :
- Solubilization : Dissolve lyophilized Aβ25-35 amide in sterile distilled water (3.33 mg/mL) or DMSO (97 mg/mL) with sonication (e.g., 10 min at 25°C) to minimize pre-aggregates .
- Aging for Aggregation : For fibril formation, incubate at 37°C for 48–72 hours in PBS (pH 7.4) or acidic conditions (pH 5.0) to mimic pathological environments .
- Quality Control : Verify monomeric vs. aggregated states via SDS-PAGE, dynamic light scattering (DLS), or Thioflavin T (ThT) fluorescence assays .
Q. What are standardized protocols for assessing Aβ25-35-induced neurotoxicity in neuronal cell models?
- Methodology :
- Cell Lines : Use PC12 (rat adrenal pheochromocytoma) or C6 (rat glioma) cells. Pre-differentiate PC12 cells with nerve growth factor (NGF) for 7 days to adopt neuron-like properties .
- Treatment : Expose cells to 20 μM Aβ25-35 for 2–48 hours. Include controls (e.g., scrambled peptide) to isolate toxicity specific to Aβ25-35 .
- Viability Assays :
- MTT Assay : Measure mitochondrial activity at 570 nm after 24–48 hours .
- Annexin V/PI Staining : Quantify apoptosis via flow cytometry .
Q. How can researchers induce and characterize Aβ25-35 fibril formation in vitro?
- Methodology :
- Incubation Conditions : Prepare Aβ25-35 (100 μM) in PBS (pH 7.4) with 0.02% NaN₃ at 37°C for 7 days under gentle agitation .
- Verification Techniques :
- Electron Microscopy (TEM) : Visualize fibril morphology .
- Circular Dichroism (CD) : Monitor β-sheet structural transitions (e.g., minima at 218 nm) .
- ThT Fluorescence : Quantify fibril kinetics using ThT (ex/em: 440/480 nm) .
Advanced Research Questions
Q. What advanced techniques are used to resolve the structural dynamics of Aβ25-35 in different microenvironments?
- Methodology :
- NMR Spectroscopy : Analyze solution-state conformations in membrane-mimetic solvents (e.g., SDS micelles) .
- Ion Mobility-Mass Spectrometry (IM-MS) : Characterize oligomeric species and their collision cross-sections .
- Computational Modeling : Use molecular dynamics simulations to predict aggregation pathways (e.g., GROMACS or AMBER software) .
Q. How can contradictory data on Aβ25-35 aggregation kinetics be resolved?
- Methodology :
- Solvent Standardization : Control pH, ionic strength, and solvent composition (e.g., avoid batch-dependent variability by using 10 mM HCl for initial solubilization) .
- Batch Consistency : Validate peptide purity (>95%) via HPLC and mass spectrometry .
- Time-Resolved Assays : Combine real-time ThT fluorescence with TEM to correlate structural and kinetic data .
Q. What strategies are effective in inhibiting Aβ25-35 aggregation or toxicity?
- Methodology :
- Molecular Tweezers : Use CLR01 (10–50 μM) to disrupt hydrophobic interactions in Aβ25-35 .
- Neuropeptide Y (NPY) : Pretreat cortical neurons with 0.5–2 μM NPY (29-64) for 24 hours to block Aβ25-35 toxicity via NGF pathway modulation .
- Natural Compounds : Screen seaweed extracts (50–100 μg/mL) for cytoprotective effects using MTT assays .
Q. How can in vitro findings on Aβ25-35 be translated to in vivo models?
- Methodology :
- Animal Models : Use transgenic C. elegans expressing human Aβ25-35 to study behavioral deficits and lifespan reduction .
- Dosing : Administer Aβ25-35 intracerebroventricularly (ICV) in rodents (e.g., 10 nmol in 5 μL saline) to mimic AD pathology .
- Biomarker Validation : Quantify Aβ25-35 in cerebrospinal fluid (CSF) using immunoaffinity-MALDI-TOF MS .
Properties
IUPAC Name |
(2S)-2-[[(2S)-2-[(2-aminoacetyl)amino]-3-hydroxypropanoyl]amino]-N-[(2S)-6-amino-1-[[2-[[(2S)-1-[[(2S,3S)-1-[[(2S,3S)-1-[[2-[[(2S)-1-[[(2S)-1-amino-4-methylsulfanyl-1-oxobutan-2-yl]amino]-4-methyl-1-oxopentan-2-yl]amino]-2-oxoethyl]amino]-3-methyl-1-oxopentan-2-yl]amino]-3-methyl-1-oxopentan-2-yl]amino]-1-oxopropan-2-yl]amino]-2-oxoethyl]amino]-1-oxohexan-2-yl]butanediamide | |
---|---|---|
Source | PubChem | |
URL | https://pubchem.ncbi.nlm.nih.gov | |
Description | Data deposited in or computed by PubChem | |
InChI |
InChI=1S/C45H82N14O13S/c1-9-24(5)36(44(71)51-21-35(64)53-29(17-23(3)4)41(68)55-27(38(49)65)14-16-73-8)59-45(72)37(25(6)10-2)58-39(66)26(7)52-34(63)20-50-40(67)28(13-11-12-15-46)56-42(69)30(18-32(48)61)57-43(70)31(22-60)54-33(62)19-47/h23-31,36-37,60H,9-22,46-47H2,1-8H3,(H2,48,61)(H2,49,65)(H,50,67)(H,51,71)(H,52,63)(H,53,64)(H,54,62)(H,55,68)(H,56,69)(H,57,70)(H,58,66)(H,59,72)/t24-,25-,26-,27-,28-,29-,30-,31-,36-,37-/m0/s1 | |
Source | PubChem | |
URL | https://pubchem.ncbi.nlm.nih.gov | |
Description | Data deposited in or computed by PubChem | |
InChI Key |
RDYXSAAQWCBCIY-SLVFWPMISA-N | |
Source | PubChem | |
URL | https://pubchem.ncbi.nlm.nih.gov | |
Description | Data deposited in or computed by PubChem | |
Canonical SMILES |
CCC(C)C(C(=O)NC(C(C)CC)C(=O)NCC(=O)NC(CC(C)C)C(=O)NC(CCSC)C(=O)N)NC(=O)C(C)NC(=O)CNC(=O)C(CCCCN)NC(=O)C(CC(=O)N)NC(=O)C(CO)NC(=O)CN | |
Source | PubChem | |
URL | https://pubchem.ncbi.nlm.nih.gov | |
Description | Data deposited in or computed by PubChem | |
Isomeric SMILES |
CC[C@H](C)[C@@H](C(=O)N[C@@H]([C@@H](C)CC)C(=O)NCC(=O)N[C@@H](CC(C)C)C(=O)N[C@@H](CCSC)C(=O)N)NC(=O)[C@H](C)NC(=O)CNC(=O)[C@H](CCCCN)NC(=O)[C@H](CC(=O)N)NC(=O)[C@H](CO)NC(=O)CN | |
Source | PubChem | |
URL | https://pubchem.ncbi.nlm.nih.gov | |
Description | Data deposited in or computed by PubChem | |
Molecular Formula |
C45H82N14O13S | |
Source | PubChem | |
URL | https://pubchem.ncbi.nlm.nih.gov | |
Description | Data deposited in or computed by PubChem | |
Molecular Weight |
1059.3 g/mol | |
Source | PubChem | |
URL | https://pubchem.ncbi.nlm.nih.gov | |
Description | Data deposited in or computed by PubChem | |
Disclaimer and Information on In-Vitro Research Products
Please be aware that all articles and product information presented on BenchChem are intended solely for informational purposes. The products available for purchase on BenchChem are specifically designed for in-vitro studies, which are conducted outside of living organisms. In-vitro studies, derived from the Latin term "in glass," involve experiments performed in controlled laboratory settings using cells or tissues. It is important to note that these products are not categorized as medicines or drugs, and they have not received approval from the FDA for the prevention, treatment, or cure of any medical condition, ailment, or disease. We must emphasize that any form of bodily introduction of these products into humans or animals is strictly prohibited by law. It is essential to adhere to these guidelines to ensure compliance with legal and ethical standards in research and experimentation.