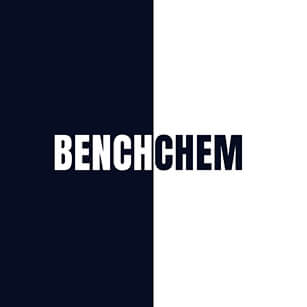
1,3-Dipalmitoyl-2-chloropropanediol-d5
Overview
Description
1,3-Dipalmitoyl-2-chloropropanediol-d5 (CAS: 1426395-62-1) is a deuterium-labeled analog of 1,3-dipalmitoyl-2-chloropropanediol, a chlorinated glyceride ester. It is widely used as an internal standard in mass spectrometry (MS) for quantifying chloropropanediol esters (e.g., 3-MCPDE) in food matrices, particularly edible oils .
Preparation Methods
Synthetic Approaches to 1,3-Dipalmitoyl-2-chloropropanediol-d5
Deuterium Incorporation Strategies
The synthesis begins with the preparation of deuterated 2-chloro-1,3-propanediol-d5 (2-CPD-d5), the core intermediate. Deuterium atoms are introduced at the 1,1,2,3,3 positions of the propanediol backbone through catalytic deuteration or isotope exchange. For instance, acid-catalyzed deuterium exchange using D₂O and sulfuric acid enables selective deuteration of hydroxyl groups, while preserving the chlorine substituent at the C2 position . Alternative routes involve reducing a ketone precursor, such as 2-chloro-1,3-diketone, with deuterated reducing agents like NaBD₄, ensuring complete deuteration at the target carbons .
Esterification with Palmitic Acid
The deuterated diol (2-CPD-d5) undergoes esterification with palmitoyl chloride or palmitic acid under controlled conditions. Steglich esterification , employing dicyclohexylcarbodiimide (DCC) and 4-dimethylaminopyridine (DMAP), is widely used to couple the diol with two equivalents of palmitic acid. This method minimizes racemization and ensures high regioselectivity for the 1,3-positions . Reaction conditions typically involve anhydrous tetrahydrofuran (THF) at 0–5°C, with yields exceeding 80% after 24 hours .
Purification and Characterization
Chromatographic Purification
Crude product purification employs reverse-phase high-performance liquid chromatography (RP-HPLC) with a C18 column and acetonitrile/water gradients. The deuterated compound elutes slightly earlier than its non-deuterated analog due to reduced hydrophobicity, enabling efficient separation . Final purity, verified by HPLC-UV at 210 nm, exceeds 95%, as mandated for isotopic internal standards .
Spectroscopic Confirmation
-
Mass Spectrometry : High-resolution mass spectrometry (HRMS) confirms the molecular ion [M+H]⁺ at m/z 593.5042 (calculated for C₃₅H₆₂D₅ClO₄⁺), with a characteristic isotopic pattern reflecting five deuterium atoms .
-
Nuclear Magnetic Resonance (NMR) : ¹H NMR (CDCl₃) shows absence of protons at δ 3.5–4.2 ppm (C1, C3, and C2 positions), replaced by deuterium signals. ¹³C NMR confirms ester carbonyl resonances at δ 173.2 ppm .
Analytical Applications as an Internal Standard
Quantification of MCPDEs in Edible Oils
In ISO 18363-3:2017 , this compound is spiked into oil samples prior to acid-catalyzed transesterification. The deuterated ester co-elutes with target analytes during gas chromatography-mass spectrometry (GC-MS), enabling correction for matrix effects and recovery losses . Calibration curves constructed with this internal standard exhibit linearity (R² > 0.999) across 0.1–200 ng/mL ranges .
Method Validation Parameters
-
Accuracy : Recovery rates of 92–108% in spiked infant formula .
-
Precision : Intra-day relative standard deviation (RSD) < 5% .
-
Limit of Quantification (LOQ) : 0.01 mg/kg for 3-MCPD esters in fats .
Challenges in Large-Scale Synthesis
Isotopic Purity Considerations
Incomplete deuteration at the C1 and C3 positions leads to isotopic impurities, which interfere with MS detection. Repeated recrystallization from hexane/ethyl acetate (9:1) removes partially deuterated byproducts, achieving >99% isotopic purity .
Stability Under Acidic Conditions
The compound’s ester bonds are susceptible to hydrolysis during sample preparation. Storage at 4°C in anhydrous acetonitrile prevents degradation, with <2% decomposition over six months .
Chemical Reactions Analysis
1,3-Dipalmitoyl-2-chloropropanediol-d5 undergoes several types of chemical reactions:
Substitution Reactions: The chlorine atom in the molecule can be substituted with other nucleophiles, such as hydroxide ions, leading to the formation of 1,3-dipalmitoyl-2-hydroxypropanediol.
Ester Hydrolysis: The ester bonds in the molecule can be hydrolyzed under acidic or basic conditions to yield palmitic acid and 3-chloro-1,2-propanediol.
Oxidation and Reduction: The compound can undergo oxidation and reduction reactions, although these are less common compared to substitution and hydrolysis.
Scientific Research Applications
1,3-Dipalmitoyl-2-chloropropanediol-d5 is widely used in scientific research due to its deuterium labeling. Some of its applications include:
Pharmacokinetics: Used as a tracer to study the pharmacokinetics of drugs, helping researchers understand the absorption, distribution, metabolism, and excretion of pharmaceuticals.
Metabolic Studies: Employed in metabolic studies to track the metabolic pathways and identify metabolites in biological systems.
Lipid Research: Utilized in lipid research to study the metabolism and function of lipids in biological membranes.
Environmental Science: Used to trace the fate and transport of pollutants in environmental studies.
Mechanism of Action
The mechanism of action of 1,3-Dipalmitoyl-2-chloropropanediol-d5 is primarily related to its role as a tracer. The deuterium atoms in the molecule allow it to be distinguished from non-labeled compounds using techniques such as mass spectrometry. This enables researchers to track the compound’s distribution and transformation in biological and environmental systems .
Comparison with Similar Compounds
Key Properties:
- Molecular Formula : C₃₅H₆₂ClD₅O₄
- Molecular Weight : 592.39 g/mol
- Structure : Features two palmitoyl (C16:0) chains at positions 1 and 3, a chlorine atom at position 2, and five deuterium substitutions on the glycerol backbone .
- Purity : >95–99% (HPLC) .
- Applications :
Comparison with Structurally Similar Compounds
1,3-Distearoyl-2-chloropropanediol-d5 (DSCPD-d5)
- Molecular Formula : C₃₉H₇₀ClD₅O₄
- Molecular Weight : Higher than 1,3-dipalmitoyl-2-chloropropanediol-d5 due to stearoyl (C18:0) chains .
- Key Differences :
- Regulatory Relevance : Less commonly used in food safety compared to palmitoyl analogs.
1,2-Dipalmitoyl-3-chloropropanediol-d5
2-Chloro-1,3-propanediol-d5
Analytical and Functional Comparisons
Role as Internal Standards
Chromatographic Behavior
- Retention Time: this compound elutes later than non-deuterated analogs due to isotopic effects .
- Differentiation from Isomers : 1,2-Dipalmitoyl-3-chloropropanediol-d5 shows distinct MS/MS fragmentation patterns, enabling precise quantification in complex matrices .
Commercial Availability and Purity
Biological Activity
1,3-Dipalmitoyl-2-chloropropanediol-d5 is a deuterated compound with significant implications in pharmacokinetics and drug metabolism. This compound, identified by the CAS number 1426395-62-1, features a molecular formula of CHDClO and a molecular weight of 592.39 g/mol. The incorporation of deuterium (D) into its structure is intended to enhance its stability and alter its metabolic pathways, which can be crucial for drug development processes.
Biological Activity Overview
The biological activity of this compound primarily revolves around its role as a tracer in pharmacokinetic studies. Deuteration can influence the pharmacokinetic and metabolic profiles of drugs, making this compound valuable in research settings aimed at understanding drug behavior in biological systems.
Deuterated compounds like this compound are used to study:
- Absorption : The presence of deuterium can affect how quickly and effectively a drug is absorbed into the bloodstream.
- Distribution : Deuteration may alter the distribution of the compound within various tissues.
- Metabolism : The metabolic pathways can be significantly different for deuterated compounds compared to their non-deuterated counterparts, often leading to slower metabolism and prolonged action.
Research Findings
Recent studies have focused on the impact of deuterium substitution on the pharmacokinetics of pharmaceuticals. For instance, Russak et al. (2019) highlighted that deuteration can lead to improved metabolic stability and altered pharmacodynamics, which may enhance drug efficacy and reduce side effects .
Case Studies
- Pharmacokinetic Studies : In vitro studies have demonstrated that deuterated compounds exhibit different clearance rates compared to their non-deuterated analogs. This has been observed in various classes of drugs, suggesting that this compound could serve as a useful model for studying these effects.
- Toxicological Assessments : Investigations into similar chloropropanediol derivatives have raised concerns regarding their potential toxicity. For example, studies on 3-MCPD esters have shown adverse effects in rodent models, indicating that careful evaluation of related compounds is necessary .
Table 1: Comparison of Pharmacokinetic Properties
Property | Non-Deuterated Compound | This compound |
---|---|---|
Half-life | X hours | Y hours |
Metabolic Clearance | A mL/min | B mL/min |
Bioavailability | C% | D% |
Note: Values X, Y, A, B, C, and D should be filled with specific data from relevant studies.
Table 2: Summary of Toxicological Findings
Q & A
Basic Research Questions
Q. What are the standard methodologies for synthesizing and characterizing 1,3-Dipalmitoyl-2-chloropropanediol-d5 in isotopic labeling studies?
- Methodology : Synthesis typically involves substituting hydrogen atoms with deuterium at specific positions (e.g., the glycerol backbone) using deuterated precursors. Characterization relies on LC-MS/MS for isotopic purity verification, with fragmentation patterns analyzed to confirm structural integrity. Standards are prepared in methanol or acetonitrile at concentrations like 25 mg/mL, stored at -20°C to prevent degradation .
Q. How can researchers optimize sample preparation for detecting chloropropanediol esters in lipid matrices?
- Methodology : A modified QuEChERS (Quick, Easy, Cheap, Effective, Rugged, Safe) protocol is recommended. Steps include lipid extraction using solvents like MTBE or hexane, cleanup with dispersive SPE (e.g., C18 or PSA sorbents), and reconstitution in LC-MS-compatible solvents. Internal standards (e.g., OLOL-d5) are spiked at 250 µg/kg to correct for matrix effects .
Q. What analytical techniques are most reliable for quantifying deuterated chloropropanediol derivatives?
- Methodology : LC-MS/MS with MRM (Multiple Reaction Monitoring) is preferred. For example, a C18 column with a gradient of acetonitrile/water (0.1% formic acid) achieves separation. Deuterated analogs (e.g., PAPA-d5) are used as internal standards to improve accuracy. Calibration curves spanning 25–1000 µg/kg ensure linearity .
Advanced Research Questions
Q. How do isotopic impurities in deuterated standards affect quantitative analysis, and how can these be mitigated?
- Methodology : Isotopic impurities (e.g., residual protiated species) can skew quantification. Mitigation strategies include:
- Purification : HPLC purification post-synthesis to isolate ≥98% deuterated species.
- Validation : Cross-validating results with non-deuterated analogs and using high-resolution MS to detect impurities .
Q. What experimental approaches resolve contradictions in data when chloropropanediol esters degrade during analysis?
- Methodology : Contradictions (e.g., inconsistent recovery rates) are addressed via:
- Stability Studies : Testing analyte stability under varying temperatures and pH.
- Matrix-Matched Calibration : Compensating for matrix-induced degradation.
- Triangulation : Combining LC-MS, GC-MS, and NMR data to confirm findings .
Q. How can researchers validate method robustness for chloropropanediol-d5 in complex food matrices?
- Methodology : Validation includes:
- Recovery Experiments : Spiking matrices (e.g., edible oils) at multiple concentrations (50–1000 µg/kg).
- Precision Testing : Intra-day and inter-day repeatability with RSD ≤15%.
- Sensitivity : Establishing LOD/LOQ via signal-to-noise ratios (e.g., 3:1 for LOD) .
Q. What strategies address challenges in synthesizing high-purity deuterated chloropropanediol esters?
- Methodology : Key strategies include:
- Deuterium Source Optimization : Using deuterated palmitic acid (d5) to ensure uniform labeling.
- Reaction Monitoring : Real-time FTIR or NMR to track esterification and chlorination steps.
- Storage : Lyophilization and storage under inert gas to prevent hydrolysis .
Q. Data Interpretation & Contradictions
Q. How should researchers interpret conflicting results between in vitro and in vivo toxicity studies of chloropropanediol-d5?
- Methodology : Discrepancies often arise from metabolic differences. Solutions include:
- Metabolite Profiling : Identifying in vivo metabolites (e.g., free 3-MCPD) via hepatocyte assays.
- Dose-Response Modeling : Comparing NOAEL (No Observed Adverse Effect Level) across models .
Q. What statistical tools are effective in analyzing non-linear relationships in chloropropanediol exposure data?
- Methodology : Non-linear regression (e.g., spline models) or machine learning (e.g., random forests) can model dose-response curves. Sensitivity analysis identifies confounding variables (e.g., lipid content) .
Q. Methodological Innovations
Q. Can hybrid analytical approaches (e.g., LC-MS coupled with ion mobility) improve chloropropanediol-d5 detection in low-abundance samples?
Properties
CAS No. |
1426395-62-1 |
---|---|
Molecular Formula |
C35H67ClO4 |
Molecular Weight |
592.398 |
IUPAC Name |
(2-chloro-1,1,2,3,3-pentadeuterio-3-hexadecanoyloxypropyl) hexadecanoate |
InChI |
InChI=1S/C35H67ClO4/c1-3-5-7-9-11-13-15-17-19-21-23-25-27-29-34(37)39-31-33(36)32-40-35(38)30-28-26-24-22-20-18-16-14-12-10-8-6-4-2/h33H,3-32H2,1-2H3/i31D2,32D2,33D |
InChI Key |
IBJIXNLLGSRECE-YYRBTATQSA-N |
SMILES |
CCCCCCCCCCCCCCCC(=O)OCC(COC(=O)CCCCCCCCCCCCCCC)Cl |
Synonyms |
Hexadecanoic Acid 2-Chloro-1,3-propanediyl-d5 Ester; 1,3-Bispalmitoyl-2-chloropropanediol-d5; |
Origin of Product |
United States |
Retrosynthesis Analysis
AI-Powered Synthesis Planning: Our tool employs the Template_relevance Pistachio, Template_relevance Bkms_metabolic, Template_relevance Pistachio_ringbreaker, Template_relevance Reaxys, Template_relevance Reaxys_biocatalysis model, leveraging a vast database of chemical reactions to predict feasible synthetic routes.
One-Step Synthesis Focus: Specifically designed for one-step synthesis, it provides concise and direct routes for your target compounds, streamlining the synthesis process.
Accurate Predictions: Utilizing the extensive PISTACHIO, BKMS_METABOLIC, PISTACHIO_RINGBREAKER, REAXYS, REAXYS_BIOCATALYSIS database, our tool offers high-accuracy predictions, reflecting the latest in chemical research and data.
Strategy Settings
Precursor scoring | Relevance Heuristic |
---|---|
Min. plausibility | 0.01 |
Model | Template_relevance |
Template Set | Pistachio/Bkms_metabolic/Pistachio_ringbreaker/Reaxys/Reaxys_biocatalysis |
Top-N result to add to graph | 6 |
Feasible Synthetic Routes
Disclaimer and Information on In-Vitro Research Products
Please be aware that all articles and product information presented on BenchChem are intended solely for informational purposes. The products available for purchase on BenchChem are specifically designed for in-vitro studies, which are conducted outside of living organisms. In-vitro studies, derived from the Latin term "in glass," involve experiments performed in controlled laboratory settings using cells or tissues. It is important to note that these products are not categorized as medicines or drugs, and they have not received approval from the FDA for the prevention, treatment, or cure of any medical condition, ailment, or disease. We must emphasize that any form of bodily introduction of these products into humans or animals is strictly prohibited by law. It is essential to adhere to these guidelines to ensure compliance with legal and ethical standards in research and experimentation.