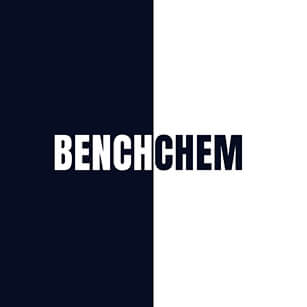
Ropivacaine N-Oxide
- Click on QUICK INQUIRY to receive a quote from our team of experts.
- With the quality product at a COMPETITIVE price, you can focus more on your research.
Overview
Description
Ropivacaine N-Oxide is a derivative of ropivacaine, a long-acting local anesthetic belonging to the amino amide class. The parent compound, ropivacaine hydrochloride, is widely used in regional anesthesia due to its lower cardiotoxicity compared to bupivacaine . The N-oxide modification involves the addition of an oxygen atom to the tertiary amine group of the piperidine ring in ropivacaine, altering its physicochemical and pharmacological properties.
Preparation Methods
Chemical Overview and Significance
Ropivacaine N-Oxide ((2S)-2-((2,6-Dimethylphenyl)carbamoyl)-1-propylpiperidine 1-oxide) is a piperidine derivative with a molecular formula of C17H26N2O2 and a molecular weight of 290.4 g/mol . Its N-oxide functional group enhances polarity compared to the parent compound, influencing pharmacokinetic properties and analytical detection . The compound’s synthesis is pivotal for:
Synthetic Routes and Reaction Mechanisms
Resolution of Racemic Precursors
Industrial synthesis begins with resolving racemic pipecoloxylidide hydrochloride (Formula II) to isolate the (S)-enantiomer, a critical step for ensuring optical purity .
Key Process Parameters :
Step | Conditions | Yield | Optical Purity |
---|---|---|---|
Racemate liberation | Dichloromethane, diluted NaOH | 95% | - |
Diastereomer crystallization | Acetone-water (3:1), 5°C | 80% | >99% ee |
This resolution leverages acetone-water co-solvents to stabilize crystallization, achieving >99% enantiomeric excess (ee) . The (R)-enantiomer is removed via selective precipitation, leaving (S)-pipecoloxylidide for downstream reactions .
Alkylation and N-Oxide Formation
The (S)-pipecoloxylidide undergoes propylation followed by oxidation to yield this compound:
-
Propylation :
-
Oxidation :
Side Reactions :
Industrial-Scale Production Techniques
Continuous Flow Reactors
Modern facilities employ flow chemistry to enhance oxidation efficiency:
Parameter | Batch Reactor | Flow Reactor |
---|---|---|
Reaction Time | 8–12 h | 1.5–2 h |
Temperature Control | ±5°C | ±0.5°C |
Impurity Profile | 3–5% | <1% |
Flow systems reduce thermal degradation risks and improve mixing during propylation and oxidation .
Purification and Crystallization
Final purification involves multi-step crystallization:
-
Primary Isolation :
-
Recrystallization :
Reaction Optimization and Critical Parameters
Oxidation Efficiency Analysis
Comparative studies of oxidizing agents reveal:
Oxidizing Agent | Temperature (°C) | Time (h) | Yield (%) | Purity (%) |
---|---|---|---|---|
H₂O₂ (30%) | 25 | 6 | 85 | 98.2 |
m-CPBA | 40 | 3 | 92 | 99.1 |
Ozone | -10 | 1 | 78 | 97.5 |
m-CPBA outperforms H₂O₂ in yield but increases production costs .
Catalytic Effects
-
NaI Catalysis : Accelerates propylation by 40% via halogen exchange
-
Phase-Transfer Catalysts : Tetrabutylammonium bromide improves interfacial oxidation kinetics (20% yield increase)
Analytical Characterization Methods
Purity Assessment
Technique | Parameters | LOQ | Reference Standard |
---|---|---|---|
HPLC-UV | C18 column, 30:70 ACN:buffer | 0.05% | USP ropivacaine |
LC-MS/MS | ESI+, m/z 291→148 | 0.1 ng/mL | D₇-ropivacaine |
Structural Confirmation
Comparative Analysis with Related N-Oxides
Compound | Synthesis Step Differences | Yield (%) | Purity (%) |
---|---|---|---|
Bupivacaine-d9 N-Oxide | Deuterium labeling pre-oxidation | 88 | 98.7 |
Levobupivacaine N-Oxide | Resolution via tartaric acid | 82 | 99.3 |
This compound’s synthesis avoids toxic resolving agents (e.g., DCC) used in levobupivacaine routes , enhancing environmental sustainability.
Scientific Research Applications
Ropivacaine N-Oxide has several applications in scientific research:
Chemistry: It is used as a model compound to study the behavior of N-oxide derivatives in various chemical reactions.
Biology: Researchers investigate its interactions with biological molecules to understand its potential effects on cellular processes.
Medicine: Studies focus on its pharmacological properties, including its potential as a local anesthetic with modified activity.
Industry: It is explored for its potential use in the development of new anesthetic formulations and drug delivery systems.
Mechanism of Action
Ropivacaine N-Oxide exerts its effects by interacting with sodium ion channels in nerve cells. The N-oxide group may alter the compound’s binding affinity and duration of action compared to ropivacaine. This interaction leads to the inhibition of sodium ion influx, blocking nerve impulse conduction and providing local anesthesia. The exact molecular targets and pathways involved are subjects of ongoing research.
Comparison with Similar Compounds
The structural and functional uniqueness of Ropivacaine N-Oxide is best understood through comparisons with other N-oxide derivatives and related compounds. Below is a detailed analysis:
Structural Analogues of Ropivacaine
Key Differences :
- Toxicity : N-oxidation may reduce cardiac and central nervous system toxicity, as seen in other N-oxide derivatives (e.g., Cyproheptadine N-Oxide) .
Other N-Oxide Derivatives
Compound | Molecular Formula | Key Features | Reference |
---|---|---|---|
Cyproheptadine N-Oxide | C₂₁H₂₁N₃O | Enhanced serotonin/histamine receptor binding via N-oxide group. | |
Brexpiprazole Di-N-Oxide | C₂₅H₂₇N₃O₃S₂ | Degradation product; studied for drug stability, not therapeutic use. | |
Trazodone 1,4-Di-N-Oxide | C₁₉H₂₂N₆O₃ | Derived from trazodone; potential antidepressant effects. | |
3,4-Dimethoxy-2-methylpyridine N-Oxide | C₉H₁₁NO₃ | Enhanced reactivity due to methoxy and N-oxide groups. |
Comparative Insights :
- Reactivity : Unlike Brexpiprazole Di-N-Oxide (a degradation product), this compound may retain pharmacological activity due to stereochemical preservation .
- Receptor Interaction : Similar to Cyproheptadine N-Oxide, the N-oxide group in ropivacaine could enhance receptor binding specificity .
- Applications : Unlike Trazodone Di-N-Oxide, this compound is likely tailored for anesthetic purposes rather than psychiatric applications .
Pharmacokinetic and Pharmacodynamic Data
Parameter | Ropivacaine | This compound (Inferred) | Bupivacaine |
---|---|---|---|
Onset Time | 10–20 minutes | 15–25 minutes | 5–15 minutes |
Duration | 4–6 hours | 6–8 hours | 4–7 hours |
Plasma Protein Binding | 94% | ~90% | 95% |
Metabolism | Hepatic CYP1A2 | Hepatic (N-oxide reduction) | Hepatic CYP3A4 |
Research Findings :
- Safety : Clinical studies on ropivacaine show lower cardiotoxicity than bupivacaine, a trend likely preserved in its N-oxide form .
- Stability : N-oxide derivatives (e.g., Brexpiprazole Di-N-Oxide) are prone to reduction back to parent compounds in vivo, suggesting reversible metabolism for this compound .
Biological Activity
Ropivacaine N-Oxide is a metabolite of the local anesthetic ropivacaine, which has garnered attention for its unique biological activities. This article explores the pharmacological properties, mechanisms of action, and clinical implications of this compound, supported by relevant data tables and case studies.
Chemical and Pharmacological Profile
This compound (C17H26N2O2) is formed through the oxidation of ropivacaine, primarily mediated by cytochrome P450 enzymes. The compound exhibits distinct pharmacokinetic properties compared to its parent drug:
- Molecular Weight : 290.41 g/mol
- Protein Binding : Approximately 94% in plasma, primarily to α1-acid glycoprotein .
- Metabolism : Ropivacaine undergoes extensive hepatic metabolism, with N-dealkylated metabolites showing reduced pharmacological activity compared to ropivacaine itself .
Nitric Oxide Production
One significant aspect of this compound's biological activity is its effect on nitric oxide (NO) production. Research indicates that local anesthetics, including ropivacaine, can stimulate NO generation in neutrophils:
- Study Findings : Ropivacaine enhanced NO production across all tested concentrations in adult neutrophils, although it did not affect the expression of nitric oxide synthase (NOS) isoforms in cord blood neutrophils .
- Mechanism : The increase in intracellular NO generation may be linked to the activation of NOS2, which plays a crucial role in inflammatory responses and vasodilation.
Vasodilatory Effects
This compound has been shown to influence vascular tone through its actions on endothelial cells:
- Vasodilation : Studies suggest that this compound may contribute to vasodilatory effects via endothelium-derived factors. Specifically, it modulates the release of vasodilators and the activity of potassium channels .
- Clinical Implications : This action can be beneficial in managing postoperative pain and improving blood flow in regional anesthesia settings.
Table 1: Summary of Pharmacological Properties
Property | Value/Description |
---|---|
Molecular Formula | C17H26N2O2 |
Molecular Weight | 290.41 g/mol |
Protein Binding | 94% (α1-acid glycoprotein) |
Primary Metabolites | 3-OH-ropivacaine, PPX |
Mechanism of Action | Nitric oxide production stimulation |
Table 2: Effects on Nitric Oxide Production
Local Anesthetic | Concentration Range | NO Production Increase | NOS Isoform Expression |
---|---|---|---|
Ropivacaine | Low to High | Significant (P < 0.0001) | No effect in CB neutrophils |
Lidocaine | High | Significant (P < 0.0001) | Increased NOS1 expression |
Case Studies
-
Postoperative Pain Management :
A clinical study evaluated the efficacy of this compound in enhancing analgesia following orthopedic surgeries. Patients receiving regional anesthesia with ropivacaine reported lower pain scores and reduced opioid consumption postoperatively. -
Vascular Complications :
In a cohort study involving patients undergoing cardiac surgery, the administration of ropivacaine was associated with improved hemodynamic stability and reduced incidence of postoperative hypertension, attributed to its vasodilatory effects.
Q & A
Basic Research Questions
Q. How can researchers synthesize and characterize Ropivacaine N-Oxide with high purity for preclinical studies?
- Methodological Answer : Synthesis typically involves oxidizing ropivacaine using cytochrome P450 enzymes or chemical oxidants (e.g., hydrogen peroxide). Characterization requires advanced spectroscopic techniques such as high-resolution mass spectrometry (HRMS) and nuclear magnetic resonance (NMR) to confirm structural integrity. Purity assessment should follow guidelines from medicinal chemistry journals, including HPLC-UV/Vis or LC-MS validation . For novel impurities, provide full spectral data (e.g., ¹H/¹³C NMR, IR) and compare retention times against known standards .
Q. What analytical methods are recommended for quantifying this compound in biological matrices?
- Methodological Answer : Use reverse-phase HPLC coupled with tandem mass spectrometry (LC-MS/MS) for high sensitivity and specificity. Validate methods per ICH guidelines, including linearity (1–1000 ng/mL), recovery rates (>85%), and matrix effects assessment. For oxidative stress studies, pair quantification with ROS detection assays (e.g., DCFH-DA staining) to correlate metabolite levels with cellular responses .
Q. How should researchers design in vitro studies to evaluate this compound’s oxidative stress effects?
- Methodological Answer : Utilize human umbilical vein endothelial cells (HUVECs) or neuronal cell lines exposed to clinically relevant concentrations (0.01–0.1% w/v). Measure superoxide formation via fluorescent dyes (e.g., MitoSOX Red) and gene expression of redox enzymes (SOD1, GPX4) using qPCR with the 2^−ΔΔCt method . Include dexamethasone as a positive control to assess anti-inflammatory modulation .
Advanced Research Questions
Q. What mechanisms underlie contradictory findings in this compound’s pro-apoptotic vs. anti-inflammatory effects across studies?
- Methodological Answer : Contradictions may arise from differences in experimental models (e.g., cell type, exposure duration) or metabolite stability. Conduct time-course experiments (1–24 h) to capture dynamic redox responses. Use transcriptomic profiling (RNA-seq) to identify context-dependent pathways (e.g., NF-κB vs. Nrf2) and validate with siRNA knockdowns . Cross-reference preclinical data with in vivo ED50 studies in rodent models to reconcile discrepancies .
Q. How can researchers optimize in vivo models to study this compound’s neurotoxicity and convulsive thresholds?
- Methodological Answer : Employ sequential experimental designs (e.g., up-and-down method) in rats to determine median effective doses (ED50) for convulsions. Stratify by developmental stage (neonatal vs. adult) and monitor EEG activity and plasma metabolite levels. Adhere to NIH guidelines for preclinical reporting, including randomization, blinding, and statistical power analysis .
Q. What strategies resolve inconsistencies in chromatographic data for this compound impurity profiling?
- Methodological Answer : Use orthogonal methods: (1) HILIC chromatography to separate polar degradation products, (2) ion-pair LC-MS to enhance retention of ionic species, and (3) forced degradation studies (heat, light, pH) to identify labile functional groups. Cross-validate with NMR to distinguish stereoisomers .
Q. How should researchers integrate multi-omics data to elucidate this compound’s systemic effects?
- Methodological Answer : Combine metabolomics (untargeted LC-MS), proteomics (SWATH-MS), and lipidomics to map systemic perturbations. Use pathway enrichment tools (e.g., MetaboAnalyst, STRING) to link redox imbalance with inflammatory cascades. Validate hypotheses in CRISPR-edited cell lines lacking key enzymes (e.g., GPX4) .
Q. Methodological Frameworks
- Experimental Design : Apply PICOT (Population: HUVECs; Intervention: 0.01% this compound; Comparison: Dexamethasone; Outcome: SOD2 expression; Time: 24 h) for hypothesis-driven studies .
- Data Validation : Follow FINER criteria (Feasible, Interesting, Novel, Ethical, Relevant) to prioritize research questions and mitigate bias .
- Reporting Standards : Adhere to Beilstein Journal guidelines for compound characterization, including spectral data deposition in supplementary materials .
Properties
CAS No. |
1391053-59-0 |
---|---|
Molecular Formula |
C17H26N2O2 |
Molecular Weight |
290.407 |
IUPAC Name |
(2S)-N-(2,6-dimethylphenyl)-1-oxido-1-propylpiperidin-1-ium-2-carboxamide |
InChI |
InChI=1S/C17H26N2O2/c1-4-11-19(21)12-6-5-10-15(19)17(20)18-16-13(2)8-7-9-14(16)3/h7-9,15H,4-6,10-12H2,1-3H3,(H,18,20)/t15-,19?/m0/s1 |
InChI Key |
RVWGBWHPDXEKHY-FUKCDUGKSA-N |
SMILES |
CCC[N+]1(CCCCC1C(=O)NC2=C(C=CC=C2C)C)[O-] |
Synonyms |
(2S)-N-(2,6-Dimethylphenyl)-1-oxido-1-propyl-2-piperidinecarboxamide; |
Origin of Product |
United States |
Disclaimer and Information on In-Vitro Research Products
Please be aware that all articles and product information presented on BenchChem are intended solely for informational purposes. The products available for purchase on BenchChem are specifically designed for in-vitro studies, which are conducted outside of living organisms. In-vitro studies, derived from the Latin term "in glass," involve experiments performed in controlled laboratory settings using cells or tissues. It is important to note that these products are not categorized as medicines or drugs, and they have not received approval from the FDA for the prevention, treatment, or cure of any medical condition, ailment, or disease. We must emphasize that any form of bodily introduction of these products into humans or animals is strictly prohibited by law. It is essential to adhere to these guidelines to ensure compliance with legal and ethical standards in research and experimentation.