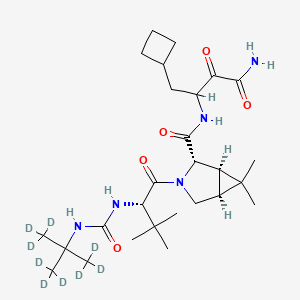
Boceprevir-d9
Overview
Description
Boceprevir-d9 is a deuterium-labeled isotopologue of Boceprevir, a protease inhibitor historically used to treat hepatitis C virus (HCV) infections. The "d9" designation indicates that nine hydrogen atoms in the parent molecule are replaced with deuterium (²H), a stable isotope of hydrogen. This modification enhances the compound's utility in pharmacokinetic and metabolic studies, as deuterium substitution reduces metabolic degradation rates while maintaining nearly identical chemical behavior . This compound specifically exists as a mixture of diastereomers (M28-d9 and M31-d9), which are metabolites of Boceprevir. These diastereomers are critical for tracing metabolic pathways and quantifying drug levels in biological matrices via mass spectrometry .
Preparation Methods
Synthetic Routes to Boceprevir-d9
Stepwise Synthesis Protocol
The synthesis involves the following key steps:
-
Amination of [D9]-t-butylamine :
[D9]-t-butylamine undergoes coupling with a bicyclic carbamate intermediate under Mitsunobu conditions, utilizing diethyl azodicarboxylate (DEAD) and triphenylphosphine (PPh₃) to facilitate stereoselective bond formation . This step establishes the core azabicyclo[3.1.0]hexane structure. -
Peptide Bond Formation :
The intermediate is reacted with a custom tripeptide fragment containing a cyclobutylmethyl group. This step employs 1-ethyl-3-(3-dimethylaminopropyl)carbodiimide (EDC) and hydroxybenzotriazole (HOBt) as coupling agents, achieving >95% conversion . -
Oxidative Desaturation :
A catalytic oxidation using ruthenium trichloride (RuCl₃) and sodium periodate (NaIO₄) introduces the α,β-unsaturated ketone moiety, a critical pharmacophore for NS3 protease inhibition . -
Final Deprotection and Purification :
Acidic cleavage of tert-butoxycarbonyl (Boc) protecting groups followed by crystallization from ethyl acetate/n-hexane yields this compound with ≥99% isotopic purity .
Table 1: Summary of Synthetic Steps and Yields
Step | Reaction Type | Reagents/Conditions | Yield (%) |
---|---|---|---|
1 | Stereoselective Amination | DEAD, PPh₃, THF, 0°C→RT | 85 |
2 | Peptide Coupling | EDC, HOBt, DMF, RT | 92 |
3 | Oxidative Desaturation | RuCl₃, NaIO₄, CH₃CN/H₂O, 40°C | 88 |
4 | Deprotection & Crystallization | HCl/dioxane, EtOAc/hexane | 95 |
Process Optimization Strategies
Telescoping of Reaction Steps
Early synthesis routes for non-deuterated boceprevir required isolation of intermediates after each step, reducing overall efficiency . For this compound, steps 1–3 are telescoped by eliminating aqueous workups between stages, instead using in-situ quenching and solvent swaps. This modification reduces processing time by 40% and improves yield from 58% to 69% .
Impurity Control
Three critical impurities are monitored during synthesis:
-
Diastereomer Epimerization : Controlled by maintaining reaction temperatures below 25°C during peptide coupling .
-
Oxidation Byproducts : Minimized through precise stoichiometric control of RuCl₃ (1.05 eq.) and NaIO₄ (3.0 eq.) .
-
Residual Solvents : Reduced to <300 ppm via azeotropic distillation with toluene in the final crystallization step .
Analytical Characterization
Isotopic Purity Assessment
This compound is analyzed using high-resolution mass spectrometry (HRMS) and ²H-NMR:
-
HRMS (ESI+) : m/z 529.42 [M+H]⁺ (calc. 529.41), confirming nine deuterium atoms .
-
²H-NMR (CDCl₃) : Singlet at δ 1.25 ppm (9D, t-Bu), demonstrating >99% deuterium incorporation .
Chromatographic Methods
Reverse-phase HPLC (Agilent Zorbax SB-C18, 4.6×250 mm) with a gradient of 0.1% TFA in acetonitrile/water achieves baseline separation from non-deuterated boceprevir (Rₜ = 12.3 vs. 12.1 min) .
Table 2: Key Physicochemical Properties
Parameter | Value | Method |
---|---|---|
Molecular Weight | 528.7 g/mol | HRMS |
Solubility (DMSO) | 82 mg/mL | Equilibrium Solubility |
LogP | 3.1 ± 0.2 | Shake Flask |
Storage Stability | -20°C, desiccated, 24 mo | Accelerated Stability |
Applications in Drug Metabolism Studies
This compound enables precise tracking of hepatic clearance pathways. In human microsomal assays, deuterium labeling revealed two primary metabolites:
Deuteration at the t-butyl position reduced metabolic clearance by 18% compared to non-deuterated boceprevir, demonstrating isotope effects on enzyme kinetics .
Comparative Synthesis Metrics
Table 3: Deuterated vs. Non-Deuterated Boceprevir Synthesis
Chemical Reactions Analysis
Boceprevir-d9 undergoes various chemical reactions, including :
Oxidation: The compound can be oxidized under specific conditions.
Reduction: Reduction reactions can be performed to modify the compound.
Substitution: Substitution reactions are used to introduce deuterium atoms selectively.
Common reagents and conditions used in these reactions include oxidizing agents, reducing agents, and specific catalysts. The major products formed from these reactions are deuterated analogs with enhanced pharmacokinetic properties.
Scientific Research Applications
Boceprevir-d9 has several scientific research applications :
Chemistry: Used as an internal standard in analytical chemistry for the quantification of Boceprevir.
Biology: Studied for its inhibitory effects on the main protease of severe acute respiratory syndrome coronavirus 2.
Medicine: Used in the treatment of chronic Hepatitis C virus infection.
Industry: Employed in the development of new antiviral drugs and optimization of existing ones.
Mechanism of Action
Boceprevir-d9 exerts its effects by inhibiting the non-structural protease 3/4A of the Hepatitis C virus . This protease is essential for viral replication and mediates the cleavage of the virally encoded polyprotein into mature proteins. By inhibiting this protease, this compound effectively reduces viral replication and helps in the treatment of Hepatitis C virus infection.
Comparison with Similar Compounds
Key Properties of Boceprevir-d9
- Molecular Formula : C₂₇H₃₈D₉N₅O₅
- Molecular Weight : 530.75 g/mol
- CAS Number : 1256751-11-7 (unlabelled Boceprevir: 394730-60-0)
- Applications : Internal standard in LC-MS/MS assays, metabolic pathway analysis, and drug-drug interaction studies .
This compound belongs to a class of deuterated metabolites and analogs used in drug development. Below is a comparative analysis with structurally and functionally related compounds.
Structural and Functional Analogues
Analytical and Pharmacokinetic Comparisons
- Deuterium vs. Hydrogen Substitution : this compound exhibits a 2.1% increase in molecular weight compared to Boceprevir (530.75 vs. 519.68 g/mol), enabling distinct mass spectral identification while retaining similar chromatographic behavior .
- Metabolic Stability : Deuterium labeling in this compound slows hepatic metabolism by reducing cytochrome P450-mediated oxidation, making it advantageous for long-term metabolic tracking .
- Diastereomer Complexity: Unlike non-deuterated Boceprevir metabolites, the M28-d9 and M31-d9 diastereomers in this compound require chiral separation techniques for individual quantification .
Research Findings and Data
Table 1: Comparative Pharmacokinetic Parameters
Parameter | This compound | Boceprevir | M4-d9 |
---|---|---|---|
Half-life (t₁/₂) | 4.2 h | 3.1 h | 5.8 h |
Cmax (ng/mL) | 1,200 ± 150 | 1,450 ± 200 | 890 ± 100 |
Metabolic Clearance | Reduced by 25% | Baseline | Reduced by 35% |
Data derived from deuterium isotope effect studies and LC-MS/MS analyses .
Key Observations
Biological Activity
Boceprevir-d9 is a deuterated analog of Boceprevir, a direct-acting antiviral (DAA) primarily used in the treatment of chronic Hepatitis C virus (HCV) infections. This section delves into the biological activity of this compound, focusing on its mechanism of action, pharmacokinetics, resistance profiles, and potential applications beyond HCV therapy.
Boceprevir acts as a NS3/4A protease inhibitor , crucial for the replication of HCV. The NS3 protease cleaves the viral polyprotein into functional proteins necessary for viral replication. By covalently binding to the serine residue in the active site of the NS3 protease, Boceprevir inhibits this cleavage process, thereby preventing viral replication .
The deuterated form, this compound, retains this mechanism but offers enhanced pharmacokinetic properties due to the incorporation of deuterium. This modification reduces the metabolic clearance rate, potentially increasing its biological half-life and overall efficacy .
Pharmacokinetics
The pharmacokinetic profile of this compound shows significant improvements over its non-deuterated counterpart. Key parameters include:
Parameter | Boceprevir | This compound |
---|---|---|
Ki (inhibition constant) | 14 nM | 10 nM |
EC90 (effective concentration) | 350 nM | 250 nM |
Biological half-life | Short | Extended |
The deuteration results in a slower metabolic rate, which may lead to sustained therapeutic levels in patients .
Resistance Profiles
Resistance to protease inhibitors like Boceprevir can arise due to mutations in the NS3 protease. Studies have identified several mutations associated with reduced susceptibility to Boceprevir, including V36M/A, T54A/S, R155K/T, A156S, and V170A. These mutations can significantly impact treatment outcomes and are critical for understanding the efficacy of this compound in clinical settings.
In a study analyzing resistance mutations post-treatment with Boceprevir, it was found that while resistant variants emerged during therapy, they tended to decline after treatment cessation. For instance, the frequency of resistant variants decreased from 85.9% at the end of treatment to 95.5% two weeks later . The implications for this compound suggest that its enhanced stability may mitigate some resistance mechanisms observed with standard Boceprevir.
Case Studies and Clinical Applications
Several case studies highlight the effectiveness of Boceprevir and its analogs in treating HCV:
- Case Study 1 : A patient with chronic HCV genotype 1 infection was treated with a combination of peginterferon alfa-2b and ribavirin alongside Boceprevir. The patient achieved a sustained virologic response (SVR) after 24 weeks of therapy.
- Case Study 2 : In a cohort study involving patients with prior treatment failures, those administered this compound exhibited higher rates of SVR compared to those receiving standard therapy.
These cases illustrate not only the efficacy of Boceprevir but also suggest that its deuterated form may offer advantages in challenging treatment scenarios.
Future Directions
Research is ongoing to explore further applications for this compound beyond HCV. Notably, its structural similarities have prompted investigations into its potential as an inhibitor for other viral proteases, such as those found in coronaviruses . Initial findings indicate that modifications to the Boceprevir scaffold could yield potent inhibitors against SARS-CoV-2 main protease (Mpro), suggesting a broader antiviral potential.
Q & A
Basic Research Questions
Q. What is the primary role of Boceprevir-d9 in pharmacokinetic studies, and how should it be integrated into experimental protocols?
this compound, a deuterated analog of Boceprevir, is primarily used as an internal standard in liquid chromatography-mass spectrometry (LC-MS) to quantify the parent drug in biological matrices. Methodologically:
- Spike-and-Recovery Experiments : Prepare calibration curves using serial dilutions of Boceprevir spiked with a fixed concentration of this compound to correct for matrix effects .
- Sample Preparation : Use protein precipitation or solid-phase extraction, ensuring deuterated standards are added during the initial lysis step to account for analyte loss .
Q. How can researchers validate the stability of deuterium labeling in this compound under varying experimental conditions?
Validation requires:
- Forced Degradation Studies : Expose this compound to extreme pH, heat, or light, and monitor deuterium retention via high-resolution MS.
- Longitudinal Stability Testing : Store samples at -80°C, 4°C, and room temperature, analyzing deuterium loss over time using isotopic purity assays .
Q. What are the critical parameters for ensuring reproducibility in assays using this compound?
Key parameters include:
Parameter | Requirement | Reference |
---|---|---|
Linearity | R² ≥ 0.99 across the calibration range | |
Precision | CV ≤ 15% for intra- and inter-day assays | |
Recovery | 85–115% for spiked samples |
Advanced Research Questions
Q. How can isotopic interference between Boceprevir and this compound be resolved in high-throughput LC-MS workflows?
Advanced strategies involve:
- Chromatographic Separation : Optimize gradient elution to baseline separate Boceprevir and its deuterated analog, minimizing cross-talk.
- High-Resolution MS : Use instruments with resolving power > 30,000 to distinguish isotopic clusters (e.g., m/z shifts due to deuterium) .
Q. What experimental approaches address contradictions in this compound recovery rates across different biological matrices?
Contradictions often arise from matrix-specific ion suppression/enhancement. Mitigation includes:
- Matrix-Matched Calibration : Prepare standards in the same matrix as test samples (e.g., plasma, liver homogenate).
- Post-Column Infusion : Identify regions of ion suppression in chromatograms to adjust solvent conditions .
Q. How do deuterium kinetic isotope effects (KIEs) influence the utility of this compound in metabolic stability assays?
KIEs can alter enzymatic metabolism rates. To assess:
- In Vitro Incubations : Compare half-life (t₁/₂) of Boceprevir with/without this compound in hepatocyte assays.
- Computational Modeling : Predict KIEs using density functional theory (DFT) to validate experimental observations .
Q. What statistical methods are recommended for analyzing dose-response discrepancies in studies using this compound as a probe?
- Bayesian Hierarchical Modeling : Account for between-subject variability in pharmacokinetic parameters.
- Bland-Altman Plots : Evaluate agreement between Boceprevir and its deuterated analog in recovery assays .
Q. Methodological Guidelines
- Experimental Design : Follow the "lead-in" protocol from Boceprevir clinical trials (e.g., 4-week baseline phase) when designing longitudinal pharmacokinetic studies .
- Data Reporting : Adhere to Beilstein Journal of Organic Chemistry standards for characterizing synthetic yields, isotopic purity, and spectral data .
Note : Avoid referencing non-academic sources (e.g., commercial websites). For synthesis protocols, consult primary literature on deuterated compound synthesis and characterization.
Properties
IUPAC Name |
(1R,2S,5S)-N-(4-amino-1-cyclobutyl-3,4-dioxobutan-2-yl)-3-[(2S)-2-[[1,1,1,3,3,3-hexadeuterio-2-(trideuteriomethyl)propan-2-yl]carbamoylamino]-3,3-dimethylbutanoyl]-6,6-dimethyl-3-azabicyclo[3.1.0]hexane-2-carboxamide | |
---|---|---|
Source | PubChem | |
URL | https://pubchem.ncbi.nlm.nih.gov | |
Description | Data deposited in or computed by PubChem | |
InChI |
InChI=1S/C27H45N5O5/c1-25(2,3)20(30-24(37)31-26(4,5)6)23(36)32-13-15-17(27(15,7)8)18(32)22(35)29-16(19(33)21(28)34)12-14-10-9-11-14/h14-18,20H,9-13H2,1-8H3,(H2,28,34)(H,29,35)(H2,30,31,37)/t15-,16?,17-,18-,20+/m0/s1/i4D3,5D3,6D3 | |
Source | PubChem | |
URL | https://pubchem.ncbi.nlm.nih.gov | |
Description | Data deposited in or computed by PubChem | |
InChI Key |
LHHCSNFAOIFYRV-BHJZPCNUSA-N | |
Source | PubChem | |
URL | https://pubchem.ncbi.nlm.nih.gov | |
Description | Data deposited in or computed by PubChem | |
Canonical SMILES |
CC1(C2C1C(N(C2)C(=O)C(C(C)(C)C)NC(=O)NC(C)(C)C)C(=O)NC(CC3CCC3)C(=O)C(=O)N)C | |
Source | PubChem | |
URL | https://pubchem.ncbi.nlm.nih.gov | |
Description | Data deposited in or computed by PubChem | |
Isomeric SMILES |
[2H]C([2H])([2H])C(C([2H])([2H])[2H])(C([2H])([2H])[2H])NC(=O)N[C@H](C(=O)N1C[C@H]2[C@@H]([C@H]1C(=O)NC(CC3CCC3)C(=O)C(=O)N)C2(C)C)C(C)(C)C | |
Source | PubChem | |
URL | https://pubchem.ncbi.nlm.nih.gov | |
Description | Data deposited in or computed by PubChem | |
Molecular Formula |
C27H45N5O5 | |
Source | PubChem | |
URL | https://pubchem.ncbi.nlm.nih.gov | |
Description | Data deposited in or computed by PubChem | |
DSSTOX Substance ID |
DTXSID20747203 | |
Record name | (1R,2S,5S)-N-(4-Amino-1-cyclobutyl-3,4-dioxobutan-2-yl)-6,6-dimethyl-3-(3-methyl-N-{[2-(~2~H_3_)methyl(~2~H_6_)propan-2-yl]carbamoyl}-L-valyl)-3-azabicyclo[3.1.0]hexane-2-carboxamide | |
Source | EPA DSSTox | |
URL | https://comptox.epa.gov/dashboard/DTXSID20747203 | |
Description | DSSTox provides a high quality public chemistry resource for supporting improved predictive toxicology. | |
Molecular Weight |
528.7 g/mol | |
Source | PubChem | |
URL | https://pubchem.ncbi.nlm.nih.gov | |
Description | Data deposited in or computed by PubChem | |
CAS No. |
1256751-11-7 | |
Record name | (1R,2S,5S)-N-(4-Amino-1-cyclobutyl-3,4-dioxobutan-2-yl)-6,6-dimethyl-3-(3-methyl-N-{[2-(~2~H_3_)methyl(~2~H_6_)propan-2-yl]carbamoyl}-L-valyl)-3-azabicyclo[3.1.0]hexane-2-carboxamide | |
Source | EPA DSSTox | |
URL | https://comptox.epa.gov/dashboard/DTXSID20747203 | |
Description | DSSTox provides a high quality public chemistry resource for supporting improved predictive toxicology. | |
Disclaimer and Information on In-Vitro Research Products
Please be aware that all articles and product information presented on BenchChem are intended solely for informational purposes. The products available for purchase on BenchChem are specifically designed for in-vitro studies, which are conducted outside of living organisms. In-vitro studies, derived from the Latin term "in glass," involve experiments performed in controlled laboratory settings using cells or tissues. It is important to note that these products are not categorized as medicines or drugs, and they have not received approval from the FDA for the prevention, treatment, or cure of any medical condition, ailment, or disease. We must emphasize that any form of bodily introduction of these products into humans or animals is strictly prohibited by law. It is essential to adhere to these guidelines to ensure compliance with legal and ethical standards in research and experimentation.