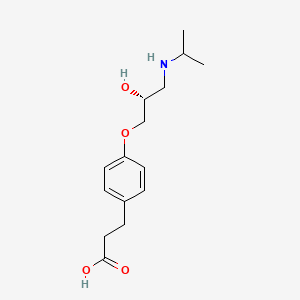
(R)-Esmolol Acid
Overview
Description
(R)-Esmolol Acid (CAS RN: 81148-15-4) is the primary acid metabolite of esmolol, an ultrashort-acting β1-adrenergic receptor antagonist. Esmolol is rapidly hydrolyzed by erythrocyte cytosolic esterases, primarily human carboxylesterase 1 (hCE1), into this compound via cleavage of its ester linkage . The metabolite has a molecular formula of $ \text{C}{15}\text{H}{23}\text{NO}_4 $ and a molecular weight of 281.35 g/mol, with a melting point of 200°C . Unlike esmolol, this compound exhibits negligible β-blocking activity (<0.1% of esmolol’s potency) and is considered pharmacologically inactive in clinical settings .
Preparation Methods
Chemical Synthesis Routes
Chiral Glycidol Derivative Condensation
The foundational chemical route involves a three-step process starting from para-hydroxybenzene methyl propionate (I). Key stages include:
-
Phenoxide Formation : Reaction of (I) with NaH in anhydrous aprotic solvents (e.g., DMF or DMSO) generates a phenoxide intermediate .
-
Condensation with (R)-Glycidol Derivatives : The phenoxide reacts with (R)-glycidyl tosylate or m-nitrobenzenesulfonate at 20–30°C to form (R)-4-glycidoxy methyl phenylpropionate (IIIb) .
-
Aminolysis and Salification : Intermediate IIIb undergoes aminolysis with isopropylamine in methanol or THF, followed by HCl-mediated salification in methyl acetate to yield (R)-esmolol hydrochloride. Acid hydrolysis then converts the hydrochloride to the free acid form .
Key Data :
Racemic Resolution via Chiral Carboxylic Acids
An alternative approach resolves racemic esmolol using chiral carboxylic acids (e.g., tartaric acid derivatives). However, this method suffers from low yields (<40%) and high reagent costs, limiting industrial adoption .
Chemo-Enzymatic Synthesis
Lipase-Catalyzed Kinetic Resolution
A biocatalytic route employs lipases to resolve racemic intermediates. The process involves:
-
Synthesis of Racemic Alcohol : Epoxide opening of (RS)-glycidol derivatives yields racemic 3-(4-(3-chloro-2-hydroxypropoxy)phenyl)propanoate .
-
Enzymatic Resolution : Lipase B from Candida antarctica selectively acylates the (S)-enantiomer, leaving the (R)-alcohol enantioenriched (98% ee) .
-
Downstream Processing : The resolved (R)-alcohol undergoes aminolysis and acidification to produce (R)-Esmolol Acid .
Key Data :
Comparative Analysis of Methods
Parameter | Chemical Synthesis | Chemo-Enzymatic Synthesis |
---|---|---|
Yield | 54.1% | 45–50% |
Enantiomeric Excess | >99% | 98% |
Reaction Conditions | 20–30°C, anhydrous | Ambient, aqueous-organic |
Scalability | Industrial | Lab-scale |
Environmental Impact | Moderate (aprotic solvents) | Low (biodegradable catalysts) |
Advantages of Chemical Synthesis :
Advantages of Chemo-Enzymatic Synthesis :
Optimization Challenges and Innovations
Solvent Selection and Reaction Kinetics
DMF and DMSO enhance phenoxide stability but require stringent drying. Substituting with cyclopentyl methyl ether (CPME) improved sustainability in recent trials, though yields dropped to 48% .
Enzymatic Process Engineering
Immobilizing lipase on silica gel increased catalyst reusability (5 cycles) while maintaining 95% ee, addressing cost barriers in biocatalysis .
Scientific Research Applications
®-Esmolol Acid has a wide range of applications in scientific research:
Chemistry: Used as a reference standard in analytical chemistry for the quantification of Esmolol and its metabolites.
Biology: Studied for its effects on beta-adrenergic receptors in various biological systems.
Medicine: Investigated for its potential therapeutic effects in cardiovascular diseases, particularly in controlling heart rate and blood pressure.
Industry: Utilized in the development of new beta-blocker drugs and in the study of drug metabolism and pharmacokinetics.
Mechanism of Action
®-Esmolol Acid exerts its effects by selectively binding to beta-1 adrenergic receptors, inhibiting the action of endogenous catecholamines such as adrenaline and noradrenaline. This inhibition leads to a decrease in heart rate and myocardial contractility, making it effective in the management of tachycardia and hypertension. The molecular targets include the beta-1 adrenergic receptors located primarily in the heart, and the pathways involved include the cyclic adenosine monophosphate (cAMP) signaling pathway.
Comparison with Similar Compounds
Esmolol vs. (R)-Esmolol Acid
Key Insight: The ultrashort action of esmolol is due to rapid hydrolysis to this compound, unlike long-acting β-blockers like atenolol .
Comparison with Other Beta-Blocker Metabolites
Atenolol Acid Metabolite
- Synthesis : Derived via sodium hydroxide-catalyzed deprotonation and epichlorohydrin addition, similar to esmolol’s synthesis but with distinct by-products .
- Activity : Retains partial β-blocking activity, contrasting with this compound’s inactivity .
Procainamide Metabolites
- N-Acetylprocainamide (NAPA) : Active metabolite with antiarrhythmic properties, unlike this compound .
Physicochemical and Pharmacokinetic Comparisons
Lipophilicity (log D)
Key Insight: Lower lipophilicity of this compound reduces central nervous system penetration compared to lipophilic β-blockers like propranolol .
Enzymatic Metabolism Pathways
Contrast : Unlike opioids metabolized by cytochrome P450, esmolol’s hydrolysis is esterase-driven, enabling rapid inactivation independent of hepatic function .
Clinical Outcomes in Specific Populations
- Sepsis-Induced Cardiomyopathy : Esmolol improves hemodynamics (e.g., SVRI: 287.71 vs. 251.17 kPa·s·L$^{-1}$) without affecting 28-day mortality, highlighting its safety over longer-acting β-blockers .
Biological Activity
Introduction
(R)-Esmolol acid is a metabolite of esmolol, a short-acting beta-1 adrenergic antagonist primarily used in clinical settings for managing tachycardia and hypertension. Understanding the biological activity of this compound is crucial for elucidating its pharmacological effects, mechanisms of action, and potential therapeutic applications. This article reviews the biological activity of this compound, focusing on its enzymatic hydrolysis, anti-inflammatory properties, and implications in clinical studies.
Enzymatic Hydrolysis
Esmolol undergoes rapid hydrolysis in the body, predominantly facilitated by carboxylesterases (CES). Research indicates that the hydrolysis rate varies significantly between different biological environments:
- Half-life in Human Blood : The half-life of (R)-Esmolol in human red blood cells (RBCs) is approximately 26.07 minutes, while the half-life in plasma is about 29.91 minutes .
- Enzyme Activity : A study demonstrated that human CES1 (hCE1) exhibits a high hydrolytic activity towards esmolol, with a rate of 255.0 ± 17.4 nmol/min/mg protein, while hCE2 showed negligible activity . This suggests that hCE1 plays a critical role in the metabolism of esmolol.
Table 1: Hydrolysis Rates of Esmolol by Different Enzymes
Enzyme | Hydrolytic Activity (nmol/min/mg protein) |
---|---|
hCE1 | 255.0 ± 17.4 |
hCE2 | Not Detected |
ESD*1 | 7.4 ± 4.3 |
ESD*2 | 8.2 ± 5.6 |
APT1 | 111.2 ± 2.9 |
Anti-inflammatory Properties
Recent studies have highlighted the anti-inflammatory effects of esmolol, particularly its ability to modulate immune responses and reduce inflammation in various conditions:
- Sepsis Model : In a rat model of sepsis, esmolol treatment resulted in decreased levels of inflammatory markers such as IL-6 and increased levels of protective factors like IL-10 and DAO. This suggests that esmolol may protect intestinal mucosa by regulating inflammatory responses .
- Mechanism of Action : The anti-inflammatory effects are mediated through the inhibition of NF-κB signaling pathways, which are crucial for inflammatory responses. Esmolol treatment was associated with increased expression of NF-κB-p65 and Bcl-2, while cleaved caspase-3 levels were reduced, indicating decreased apoptosis .
Case Study: Esmolol in Sepsis
In a controlled study involving sepsis-induced rats:
- Groups : Rats were divided into three groups: sham operation with normal saline (SNS), cecal ligation and puncture with normal saline (CNS), and cecal ligation and puncture with esmolol treatment (CE).
- Findings : The CE group showed significantly lower intestinal damage compared to the CNS group, as evidenced by histopathological examinations .
Clinical Applications
Esmolol's unique pharmacokinetic properties make it an attractive option for various clinical applications beyond its traditional use:
- Diabetic Foot Ulcers : A recent trial investigated topical esmolol hydrochloride for treating diabetic foot ulcers (DFUs). Results indicated that patients receiving esmolol alongside standard care achieved higher rates of ulcer closure within 12 weeks compared to those receiving standard care alone .
Table 2: Efficacy of Esmolol in Diabetic Foot Ulcers
Treatment Group | Ulcer Closure Rate (%) | Odds Ratio (95% CI) |
---|---|---|
Esmolol + Standard Care | 60.3 | 2.13 (1.08-4.17) |
Standard Care Only | 41.7 | - |
Q & A
Basic Research Questions
Q. What are the key considerations for synthesizing and characterizing (R)-Esmolol Acid in laboratory settings?
- Methodology : Synthesis typically involves stereoselective routes to preserve the (R)-enantiomer configuration. Characterization requires chiral HPLC or LC-MS to confirm enantiomeric purity (>98%) and structural integrity. UV-Vis spectroscopy (λmax: 222 nm) and nuclear magnetic resonance (NMR) are critical for verifying functional groups and molecular structure . Stability studies under -20°C storage conditions are essential to ensure long-term viability (>4 years).
Q. How does this compound interact with β1-adrenergic receptors in standard experimental models?
- Methodology : Use isolated guinea pig right atrial preparations to assess dose-dependent inhibition of isoproterenol-induced chronotropy. Calculate pA2 values (e.g., 3.73) to quantify antagonistic potency. In vivo models, such as anesthetized dogs, can evaluate hemodynamic responses (e.g., prevention of tachycardia) via continuous arterial pressure monitoring .
Q. What analytical techniques are recommended for quantifying this compound and its impurities in research samples?
- Methodology : Reverse-phase HPLC with UV detection (220–230 nm) is standard for purity assessment. For impurity profiling (e.g., residual esmolol or degradation products), tandem mass spectrometry (LC-MS/MS) coupled with ion-pair chromatography provides high sensitivity. Reference standards should align with ICH guidelines for validation (linearity, LOD/LOQ) .
Advanced Research Questions
Q. How can researchers address contradictions in reported β1-selectivity of this compound across different experimental systems?
- Methodology : Conduct comparative studies using tissue-specific models (e.g., atrial vs. ventricular cardiomyocytes) to assess receptor subtype affinity. Employ radioligand binding assays (e.g., -CGP 12177) to quantify binding kinetics (Kd, Bmax) under varying physiological conditions (pH, temperature). Meta-analyses of historical data may resolve discrepancies by identifying confounding variables (e.g., esterase activity in blood samples) .
Q. What experimental designs are optimal for studying the pharmacokinetic-pharmacodynamic (PK-PD) relationship of this compound in preclinical models?
- Methodology : Use compartmental modeling in rodents to correlate plasma concentration-time profiles (via LC-MS/MS) with hemodynamic effects (e.g., heart rate reduction). Incorporate enzyme kinetic assays to measure erythrocyte esterase activity, which governs esmolol-to-acid conversion rates. Non-linear mixed-effects modeling (NONMEM) can account for inter-species variability .
Q. How can impurity profiling of this compound enhance reproducibility in pharmacological studies?
- Methodology : Structural elucidation of synthesis-related impurities (e.g., diastereomers, hydrolytic byproducts) via high-resolution NMR and X-ray crystallography. Establish impurity thresholds using accelerated stability studies (40°C/75% RH) and correlate impurity levels with bioactivity changes in receptor-binding assays .
Q. What strategies mitigate confounding factors when evaluating this compound’s cardioprotective effects in sepsis models?
- Methodology : In septic shock models (e.g., LPS-induced rats), standardize endpoints (e.g., cardiac output, cytokine levels) and control for variables like fluid resuscitation protocols. Dose-response studies with low-dose esmolol acid (0.5–1 mg/kg) can isolate β1-blockade effects from systemic vasodilation. Blinded, randomized allocation reduces bias in outcome assessment .
Q. Methodological Guidance
Q. How should researchers design dose-escalation studies for this compound while ensuring ethical compliance?
- Methodology : Follow the 3Rs principle (Replacement, Reduction, Refinement) for animal studies. Use pilot studies to determine the maximum tolerated dose (MTD) via telemetric monitoring of vital signs. Submit protocols to institutional review boards (IRBs) with detailed harm-benefit analyses, including humane endpoints (e.g., >20% weight loss) .
Q. What statistical approaches are robust for analyzing contradictory outcomes in this compound trials?
- Methodology : Apply Bayesian hierarchical models to pool data from heterogeneous studies (e.g., varying species, doses). Sensitivity analyses can test the influence of outlier datasets. For small-sample studies, non-parametric tests (e.g., Wilcoxon signed-rank) reduce type I/II error risks .
Q. How can systematic reviews improve evidence synthesis for this compound’s therapeutic potential?
- Methodology : Use PRISMA guidelines to search databases (MEDLINE, Embase) and registries (ClinicalTrials.gov ) without language restrictions. Two independent reviewers should screen studies using predefined PICOT criteria (Population, Intervention, Comparison, Outcome, Timeframe). GRADE assessments evaluate evidence quality, highlighting gaps for future research .
Properties
IUPAC Name |
3-[4-[(2R)-2-hydroxy-3-(propan-2-ylamino)propoxy]phenyl]propanoic acid | |
---|---|---|
Source | PubChem | |
URL | https://pubchem.ncbi.nlm.nih.gov | |
Description | Data deposited in or computed by PubChem | |
InChI |
InChI=1S/C15H23NO4/c1-11(2)16-9-13(17)10-20-14-6-3-12(4-7-14)5-8-15(18)19/h3-4,6-7,11,13,16-17H,5,8-10H2,1-2H3,(H,18,19)/t13-/m1/s1 | |
Source | PubChem | |
URL | https://pubchem.ncbi.nlm.nih.gov | |
Description | Data deposited in or computed by PubChem | |
InChI Key |
ILSCWPMGTDPATI-CYBMUJFWSA-N | |
Source | PubChem | |
URL | https://pubchem.ncbi.nlm.nih.gov | |
Description | Data deposited in or computed by PubChem | |
Canonical SMILES |
CC(C)NCC(COC1=CC=C(C=C1)CCC(=O)O)O | |
Source | PubChem | |
URL | https://pubchem.ncbi.nlm.nih.gov | |
Description | Data deposited in or computed by PubChem | |
Isomeric SMILES |
CC(C)NC[C@H](COC1=CC=C(C=C1)CCC(=O)O)O | |
Source | PubChem | |
URL | https://pubchem.ncbi.nlm.nih.gov | |
Description | Data deposited in or computed by PubChem | |
Molecular Formula |
C15H23NO4 | |
Source | PubChem | |
URL | https://pubchem.ncbi.nlm.nih.gov | |
Description | Data deposited in or computed by PubChem | |
Molecular Weight |
281.35 g/mol | |
Source | PubChem | |
URL | https://pubchem.ncbi.nlm.nih.gov | |
Description | Data deposited in or computed by PubChem | |
Disclaimer and Information on In-Vitro Research Products
Please be aware that all articles and product information presented on BenchChem are intended solely for informational purposes. The products available for purchase on BenchChem are specifically designed for in-vitro studies, which are conducted outside of living organisms. In-vitro studies, derived from the Latin term "in glass," involve experiments performed in controlled laboratory settings using cells or tissues. It is important to note that these products are not categorized as medicines or drugs, and they have not received approval from the FDA for the prevention, treatment, or cure of any medical condition, ailment, or disease. We must emphasize that any form of bodily introduction of these products into humans or animals is strictly prohibited by law. It is essential to adhere to these guidelines to ensure compliance with legal and ethical standards in research and experimentation.