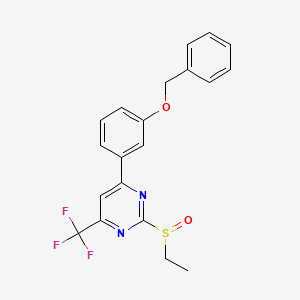
Betp
Overview
Description
BetP is a sodium (Na⁺)-coupled glycine betaine symporter from Corynebacterium glutamicum, functioning as a critical osmoregulatory transporter. It responds to hyperosmotic stress by accumulating the compatible solute glycine betaine, thereby restoring cellular water balance . Key features include:
- Dual Functionality: Combines transport catalysis (glycine betaine uptake) and osmosensing (via intracellular K⁺ concentration and membrane property changes) .
- Structural Properties: Forms a homotrimer, with each protomer independently transporting substrate. The C-terminal domain is essential for osmotic activation, while the N-terminal domain modulates activity .
- Activation Mechanism: Requires two stimuli—(i) elevated intracellular K⁺ and (ii) membrane alterations (e.g., lipid composition or amphipathic agents like tetracaine) .
- Conformational States: Captured in outward-open, inward-open, and occluded states, revealing a unique intermediate conformation during the alternating-access cycle .
Preparation Methods
Synthetic Routes and Reaction Conditions
BETP can be synthesized through a multi-step process involving the formation of the pyrimidine core, followed by the introduction of the benzyloxyphenyl, ethylsulfinyl, and trifluoromethyl groups. The synthesis typically involves:
Formation of the pyrimidine core: This can be achieved through the reaction of appropriate precursors under conditions that promote cyclization.
Introduction of the benzyloxyphenyl group: This step involves the coupling of a benzyloxyphenyl derivative with the pyrimidine core using a palladium-catalyzed cross-coupling reaction.
Introduction of the ethylsulfinyl group: This can be done through the oxidation of an ethylthio group to an ethylsulfinyl group using an oxidizing agent such as hydrogen peroxide.
Introduction of the trifluoromethyl group: This step involves the addition of a trifluoromethyl group to the pyrimidine core using a reagent such as trifluoromethyl iodide.
Industrial Production Methods
The industrial production of this compound follows similar synthetic routes but is optimized for large-scale production. This involves the use of high-throughput screening and optimization of reaction conditions to maximize yield and purity. The process may also involve the use of automated synthesis equipment and continuous flow reactors to improve efficiency and scalability .
Chemical Reactions Analysis
Types of Reactions
BETP undergoes various chemical reactions, including:
Oxidation: The ethylthio group can be oxidized to an ethylsulfinyl group.
Reduction: The ethylsulfinyl group can be reduced back to an ethylthio group.
Substitution: The trifluoromethyl group can be substituted with other functional groups under appropriate conditions.
Common Reagents and Conditions
Oxidation: Hydrogen peroxide is commonly used as an oxidizing agent.
Reduction: Reducing agents such as sodium borohydride can be used.
Substitution: Various nucleophiles can be used for substitution reactions, depending on the desired functional group.
Major Products
The major products formed from these reactions include derivatives of this compound with modified functional groups, such as ethylthio, ethylsulfinyl, and substituted trifluoromethyl groups .
Scientific Research Applications
BETP has several scientific research applications, including:
Chemistry: this compound is used as a tool compound to study the glucagon-like peptide-1 receptor and its role in glucose metabolism.
Biology: this compound is used to investigate the signaling pathways involved in insulin secretion and glucose homeostasis.
Medicine: this compound is being explored as a potential therapeutic agent for the treatment of type 2 diabetes mellitus and obesity.
Industry: This compound is used in the development of new drugs targeting the glucagon-like peptide-1 receptor.
Mechanism of Action
BETP exerts its effects by binding to the glucagon-like peptide-1 receptor, which is a G protein-coupled receptor. Upon binding, this compound acts as a positive allosteric modulator, enhancing the receptor’s response to its natural ligand, glucagon-like peptide-1. This leads to increased insulin secretion and improved glucose metabolism. The molecular targets involved include the receptor itself and downstream signaling pathways such as the cyclic adenosine monophosphate pathway .
Comparison with Similar Compounds
Comparison with Similar Transporters
Structural Comparison
Key Insights :
- This compound’s trimerization is unique among LeuT-fold transporters and is critical for regulatory communication between protomers .
- Unlike this compound, ProP lacks a K⁺-sensing domain and responds directly to membrane tension .
Functional and Mechanistic Comparison
Transport Activity and Kinetics
Key Insights :
- This compound exhibits positive cooperativity for Na⁺ binding (Hill coefficient = 1.9), suggesting sequential ion binding .
- Mhp1 and LeuT lack osmotic regulation, relying solely on substrate and ion gradients .
Regulatory Mechanisms
Key Insights :
- This compound’s activation requires both K⁺-sensing and membrane-mediated stimuli, whereas ProP responds to physical membrane changes .
- Trimer disruption in this compound abolishes osmoregulation but retains basal transport activity, highlighting the structural basis of regulation .
Substrate and Ion Coupling
- This compound : Strict specificity for glycine betaine; two Na⁺ ions bind sequentially to sites Na1 and Na2 .
- vSGLT : Transports galactose with a 2:1 Na⁺:substrate stoichiometry; Na⁺ binding precedes substrate .
Key Research Findings
Conformational Dynamics : this compound adopts inward-facing states in high Na⁺ and mixed states with betaine, as shown by pulsed EPR and molecular simulations .
Membrane Lipid Dependency : this compound activity in proteoliposomes shifts with phosphatidylglycerol content, mimicking host-specific regulation in E. coli vs. C. glutamicum .
Biological Activity
Introduction
BetP is a trimeric betaine symporter found in Corynebacterium glutamicum, playing a crucial role in osmoregulation by transporting betaine across the cell membrane. This compound is particularly interesting due to its unique mechanism of action, which is influenced by lipid-protein interactions and ionic conditions within the cellular environment. This article explores the biological activity of this compound, focusing on its structural properties, regulatory mechanisms, and implications for cellular function.
Structural Characteristics
This compound is characterized as a sodium-coupled transporter that operates with a stoichiometry of 2 Na ions per betaine molecule transported. The structural integrity of this compound is significantly influenced by its interaction with membrane lipids, particularly negatively charged phospholipids such as phosphatidylglycerol. Recent studies have utilized techniques like X-ray crystallography and Fourier transform infrared (FTIR) spectroscopy to elucidate these interactions.
Key Findings
- Lipid-Protein Interactions : this compound requires negatively charged lipids for activation and regulation. The presence of these lipids stabilizes the transporter in its functional state, while changes in membrane composition can lead to alterations in transport activity .
- Osmotic Sensing : The C-terminal domain of this compound acts as an osmosensor, detecting changes in osmotic pressure and modulating transport activity accordingly. This domain undergoes conformational changes in response to potassium ion (K) binding, which is essential for the activation of the transporter under hyperosmotic stress conditions .
Mechanisms of Regulation
This compound's activity is tightly regulated by both lipid composition and ionic concentrations.
Regulation by Lipids
- Role of Phospholipids : Studies indicate that this compound's interaction with phosphatidylglycerol is critical for its function. When reconstituted in lipid bilayers devoid of negatively charged lipids, this compound exhibits reduced transport activity, highlighting the importance of lipid composition in maintaining its functional state .
- FTIR Spectroscopy Insights : FTIR studies have shown that K binding weakens interactions between this compound and anionic lipid head groups, suggesting a dynamic regulatory mechanism where lipid interactions can modulate the transport state of the protein .
Regulation by Potassium Ions
- K Binding Sites : Research has identified specific K binding sites within the C-terminal domains of this compound. The presence of K induces a partial unfolding of these domains, transitioning this compound from a downregulated to an activated state. This process is cooperative, with Hill coefficients indicating strong interaction dynamics .
- Activation Thresholds : The half-activation constants for K have been determined to be approximately 220 mM and 242 mM under different experimental conditions, indicating that this compound requires relatively high concentrations of K for optimal activation .
Case Studies and Experimental Evidence
Several experimental studies have provided insights into the biological activity of this compound:
- Biochemical Assays : In vitro assays demonstrated that this compound can transport betaine against a concentration gradient when activated by sodium ions and K, confirming its role as an osmoregulatory transporter.
- Structural Analysis : Cryo-electron microscopy (CryoEM) studies have visualized the conformational states of this compound in various ionic environments, providing evidence for its dynamic structural adaptations during transport cycles .
- Functional Studies : Experiments using proteoliposomes have shown that altering lipid composition significantly affects this compound’s transport efficiency, reinforcing the concept that lipid-protein interactions are critical for its biological function .
Data Table: Summary of Key Findings on this compound
Q & A
Basic Research Questions
Q. What experimental methodologies are critical for studying BetP’s conformational dynamics under varying substrate and ion concentrations?
Answer: Spectroscopic techniques like PELDOR (Pulsed Electron-Electron Double Resonance) combined with molecular simulations (e.g., EBMetaD) are essential. These methods allow precise mapping of this compound’s structural changes in response to sodium (Na⁺) and betaine binding. For example, PELDOR measurements on spin-labeled this compound in proteoliposomes reveal distance distributions between residues under different ionic conditions, while EBMetaD simulations rank conformational states based on experimental data .
Q. How does this compound distinguish between osmotic stress and ionic stimuli in its activation mechanism?
Answer: this compound activation requires two stimuli: (1) intracellular K⁺ concentration and (2) membrane property changes induced by osmotic stress or amphiphilic agents like tetracaine. In Corynebacterium glutamicum, K⁺ alone is insufficient for full activation; membrane tension or lipid composition alterations are necessary. Experimental validation involves comparing this compound activity in liposomes (pre-stimulated by K⁺) versus cells (requiring osmotic stress) .
Q. What in vitro assays are used to quantify this compound’s substrate transport efficiency?
Answer: Radiolabeled betaine uptake assays in proteoliposomes or cell-based systems are standard. Key parameters include:
Advanced Research Questions
Q. How do conflicting spectroscopic and simulation data on this compound’s conformational states inform model refinement?
Answer: Discrepancies arise when experimental PELDOR distance distributions (e.g., dual peaks in betaine-bound states) cannot be replicated by simulating single X-ray structures. Advanced strategies include:
- Multi-walker simulations to explore mixed conformational ensembles.
- Free-energy calculations (e.g., EBMetaD) to quantify work required to match experimental data. For example, simulations of this compound’s Na⁺-bound inward-facing conformation required less work to match PELDOR data than outward-facing states, suggesting a dominant population under specific conditions .
Q. What mechanisms underlie this compound’s role as a GLP-1 receptor (GLP-1R) allosteric modulator, and how can this be exploited in drug discovery?
Answer: this compound covalently binds GLP-1R at Cys346, lowering the activation energy for agonist-induced conformational changes. Methodological insights include:
- cAMP washout assays to confirm covalent modulation (e.g., sustained activity post-BETP removal).
- High-throughput screening (HTS) in this compound-sensitized receptor systems to identify weak agonists (e.g., Danuglipron).
Assay Type | Key Finding | Reference |
---|---|---|
HTS with this compound | 280,000 compounds screened; Cmpd 2 EC₅₀ = 10 μM | |
β-Arrestin recruitment | This compound enhances βArr Emax by 40% |
Q. How do contradictory findings about this compound’s activation in liposomes versus cellular systems impact transport mechanism models?
Answer: In liposomes, this compound achieves full activity with K⁺ alone, whereas cells require additional osmotic stress. This discrepancy suggests:
- Membrane context dependence : Native membranes may impose kinetic barriers absent in reconstituted systems.
- Stimulus hierarchy : K⁺ primes this compound, while membrane tension fine-tunes activity. Experimental validation involves comparing molecular activity (turnover number) in liposomes vs. cells using fluorescence-based transport assays .
Q. Data Contradiction Analysis
Q. Why do some studies report this compound’s insensitivity to anion type, while others highlight ion-specific effects?
Answer: Apparent contradictions arise from experimental conditions:
- Anion insensitivity : Observed in K⁺-activation studies, where Cl⁻ vs. glutamate had no effect on this compound activity .
- Ion-specific effects : Reported in Na⁺-coupled transport assays, where anion size influences betaine binding kinetics. Resolution requires standardizing buffer compositions (e.g., ionic strength, osmolality) across studies .
Q. Methodological Recommendations
- For conformational studies : Combine PELDOR with multi-walker EBMetaD simulations to resolve mixed-state ensembles .
- For drug discovery : Use this compound-sensitized GLP-1R systems in HTS to amplify weak agonist signals .
- For transport assays : Validate findings in both liposomes and native membranes to account for contextual activation .
Properties
IUPAC Name |
2-ethylsulfinyl-4-(3-phenylmethoxyphenyl)-6-(trifluoromethyl)pyrimidine | |
---|---|---|
Source | PubChem | |
URL | https://pubchem.ncbi.nlm.nih.gov | |
Description | Data deposited in or computed by PubChem | |
InChI |
InChI=1S/C20H17F3N2O2S/c1-2-28(26)19-24-17(12-18(25-19)20(21,22)23)15-9-6-10-16(11-15)27-13-14-7-4-3-5-8-14/h3-12H,2,13H2,1H3 | |
Source | PubChem | |
URL | https://pubchem.ncbi.nlm.nih.gov | |
Description | Data deposited in or computed by PubChem | |
InChI Key |
NTDFYGSSDDMNHI-UHFFFAOYSA-N | |
Source | PubChem | |
URL | https://pubchem.ncbi.nlm.nih.gov | |
Description | Data deposited in or computed by PubChem | |
Canonical SMILES |
CCS(=O)C1=NC(=CC(=N1)C(F)(F)F)C2=CC(=CC=C2)OCC3=CC=CC=C3 | |
Source | PubChem | |
URL | https://pubchem.ncbi.nlm.nih.gov | |
Description | Data deposited in or computed by PubChem | |
Molecular Formula |
C20H17F3N2O2S | |
Source | PubChem | |
URL | https://pubchem.ncbi.nlm.nih.gov | |
Description | Data deposited in or computed by PubChem | |
Molecular Weight |
406.4 g/mol | |
Source | PubChem | |
URL | https://pubchem.ncbi.nlm.nih.gov | |
Description | Data deposited in or computed by PubChem | |
CAS No. |
1371569-69-5 | |
Record name | 1371569-69-5 | |
Source | European Chemicals Agency (ECHA) | |
URL | https://echa.europa.eu/information-on-chemicals | |
Description | The European Chemicals Agency (ECHA) is an agency of the European Union which is the driving force among regulatory authorities in implementing the EU's groundbreaking chemicals legislation for the benefit of human health and the environment as well as for innovation and competitiveness. | |
Explanation | Use of the information, documents and data from the ECHA website is subject to the terms and conditions of this Legal Notice, and subject to other binding limitations provided for under applicable law, the information, documents and data made available on the ECHA website may be reproduced, distributed and/or used, totally or in part, for non-commercial purposes provided that ECHA is acknowledged as the source: "Source: European Chemicals Agency, http://echa.europa.eu/". Such acknowledgement must be included in each copy of the material. ECHA permits and encourages organisations and individuals to create links to the ECHA website under the following cumulative conditions: Links can only be made to webpages that provide a link to the Legal Notice page. | |
Disclaimer and Information on In-Vitro Research Products
Please be aware that all articles and product information presented on BenchChem are intended solely for informational purposes. The products available for purchase on BenchChem are specifically designed for in-vitro studies, which are conducted outside of living organisms. In-vitro studies, derived from the Latin term "in glass," involve experiments performed in controlled laboratory settings using cells or tissues. It is important to note that these products are not categorized as medicines or drugs, and they have not received approval from the FDA for the prevention, treatment, or cure of any medical condition, ailment, or disease. We must emphasize that any form of bodily introduction of these products into humans or animals is strictly prohibited by law. It is essential to adhere to these guidelines to ensure compliance with legal and ethical standards in research and experimentation.