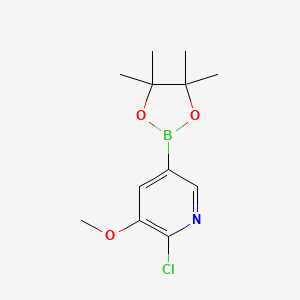
2-Chloro-3-methoxy-5-(4,4,5,5-tetramethyl-1,3,2-dioxaborolan-2-yl)pyridine
Overview
Description
2-Chloro-3-methoxy-5-(4,4,5,5-tetramethyl-1,3,2-dioxaborolan-2-yl)pyridine is a heteroaromatic boronate ester with a pyridine core substituted at positions 2 (chloro), 3 (methoxy), and 5 (pinacol boronate). The boronate group enables participation in Suzuki-Miyaura cross-coupling reactions, a cornerstone of modern synthetic chemistry for constructing biaryl systems . The chloro and methoxy substituents modulate electronic effects, influencing reactivity and stability. This compound is pivotal in pharmaceutical and agrochemical synthesis, where regioselective functionalization is critical .
Preparation Methods
Palladium-Catalyzed Borylation: Primary Synthetic Route
The most widely reported method for synthesizing pyridine-based boronate esters involves palladium-catalyzed Miyaura borylation. For the structurally related 3-chloro-2-methoxy-5-(4,4,5,5-tetramethyl-1,3,2-dioxaborolan-2-yl)pyridine (CAS 1083168-91-5), the procedure entails reacting 5-bromo-3-chloro-2-methoxypyridine with bis(pinacolato)diboron (B₂pin₂) in the presence of a palladium catalyst .
Reaction Conditions and Protocol
The synthesis is conducted under inert conditions (N₂ atmosphere) using anhydrous dimethylformamide (DMF) as the solvent. Key parameters include:
Parameter | Value/Detail |
---|---|
Starting material | 5-Bromo-3-chloro-2-methoxypyridine |
Boronating agent | Bis(pinacolato)diboron (1.2 equiv) |
Catalyst | Pd(dppf)Cl₂ (5 mol%) |
Base | Potassium acetate (3 equiv) |
Temperature | 80°C |
Reaction time | 12–16 hours (overnight) |
Yield | 78% |
After completion, the crude product is purified via silica gel chromatography using a gradient of ethyl acetate in hexanes .
Adaptation to the Target Compound
To synthesize 2-chloro-3-methoxy-5-(4,4,5,5-tetramethyl-1,3,2-dioxaborolan-2-yl)pyridine , the same protocol may be applied using 5-bromo-2-chloro-3-methoxypyridine as the starting material. The regiochemical outcome depends on the position of the bromine and methoxy groups in the precursor, necessitating precise control over the starting material’s structure.
Mechanistic Insights
The reaction proceeds through a palladium-mediated catalytic cycle:
-
Oxidative Addition : Pd⁰ inserts into the C–Br bond of the bromopyridine derivative.
-
Transmetallation : Borylation occurs via ligand exchange between the palladium intermediate and B₂pin₂.
-
Reductive Elimination : The palladium catalyst releases the boronate ester product, regenerating Pd⁰ for subsequent cycles .
The methoxy and chloro substituents influence electron density and steric effects, potentially altering reaction rates and regioselectivity.
Optimization of Reaction Parameters
Catalyst Selection
Pd(dppf)Cl₂ (dichloro[1,1'-bis(diphenylphosphino)ferrocene]palladium(II)) is preferred due to its stability and efficiency in borylation reactions. Alternative catalysts like Pd(PPh₃)₄ or Pd(OAc)₂ with supporting ligands (e.g., SPhos) may also be viable but require empirical validation .
Solvent and Temperature
DMF’s high polarity facilitates the dissolution of ionic intermediates, but toluene or 1,4-dioxane could be explored for improved selectivity. Elevated temperatures (80–100°C) are critical for achieving full conversion within practical timeframes.
Stoichiometry and Additives
A slight excess of B₂pin₂ (1.2–1.5 equiv) ensures complete conversion of the brominated precursor. Potassium acetate neutralizes HBr generated during the reaction, preventing catalyst poisoning.
Purification and Characterization
Chromatographic Purification
Silica gel chromatography with ethyl acetate/hexanes (0–100% gradient) effectively isolates the product from unreacted starting materials and boron byproducts. The target compound typically elutes at moderate polarity due to its boronate ester group .
Analytical Data
-
ESI-MS : m/z 270.3 ([M+H]⁺), consistent with a molecular weight of 269.53 g/mol .
-
Retention Time : 2.07 minutes (HPLC, reverse-phase C18 column) .
-
¹H NMR : Expected signals include a singlet for the pinacol methyl groups (δ 1.2–1.3 ppm) and aromatic protons (δ 7.5–8.5 ppm).
Industrial-Scale Production
While laboratory-scale synthesis employs batch reactions, industrial processes may utilize continuous flow systems to enhance reproducibility and yield. Large-scale purification could involve recrystallization from ethanol/water mixtures instead of chromatography.
Chemical Reactions Analysis
Types of Reactions
2-Chloro-3-methoxy-5-(4,4,5,5-tetramethyl-1,3,2-dioxaborolan-2-yl)pyridine undergoes various types of chemical reactions, including:
Substitution Reactions: The chloro group can be substituted with other nucleophiles.
Coupling Reactions: The boronic ester group is highly reactive in Suzuki-Miyaura cross-coupling reactions, forming carbon-carbon bonds with aryl or vinyl halides.
Oxidation and Reduction: The methoxy group can undergo oxidation to form aldehydes or carboxylic acids, and reduction to form alcohols.
Common Reagents and Conditions
Palladium Catalysts: Used in coupling reactions.
Bases: Such as potassium carbonate or sodium hydroxide, used to deprotonate the boronic ester.
Oxidizing Agents: Such as potassium permanganate or chromium trioxide for oxidation reactions.
Reducing Agents: Such as lithium aluminum hydride or sodium borohydride for reduction reactions.
Major Products
Substituted Pyridines: Resulting from nucleophilic substitution.
Biaryl Compounds: Formed through Suzuki-Miyaura coupling.
Alcohols, Aldehydes, and Carboxylic Acids: From oxidation and reduction of the methoxy group.
Scientific Research Applications
Overview
2-Chloro-3-methoxy-5-(4,4,5,5-tetramethyl-1,3,2-dioxaborolan-2-yl)pyridine is a boronic ester derivative of pyridine known for its utility in organic synthesis. The compound's structure features a chloro group, a methoxy group, and a boronic ester moiety, which collectively enhance its reactivity in various chemical reactions. This article explores its applications in scientific research, particularly in organic synthesis and medicinal chemistry.
Applications in Organic Synthesis
The primary application of this compound lies in its role as a reagent in the Suzuki-Miyaura cross-coupling reaction. This reaction is pivotal for forming carbon-carbon bonds and is widely utilized in synthesizing complex organic molecules.
Suzuki-Miyaura Cross-Coupling Reactions
The compound can act as a boronic ester in Suzuki-Miyaura reactions to couple with various aryl halides or other electrophiles. This process is essential for developing pharmaceuticals and agrochemicals.
Mechanism of Action :
- The compound undergoes transmetalation with palladium catalysts.
- It facilitates the formation of biaryl compounds through the coupling of aryl groups.
Synthesis of Bioactive Compounds
Research indicates that derivatives of this compound can be used to synthesize biologically active molecules. For example:
- In studies involving arginase inhibitors, boronic esters similar to this compound have shown significant inhibition against human arginase enzymes (hARG-1 and hARG-2) .
Medicinal Chemistry Applications
The compound has potential applications in drug discovery and development due to its ability to form stable complexes with biological targets.
Inhibitors for Viral Proteins
Recent studies have highlighted the use of pyridine derivatives as inhibitors for viral proteins such as SARS-CoV-2 3CL protease. Compounds structurally related to this compound have demonstrated promising antiviral activity .
Development of Small Molecule Drugs
The compound's ability to participate in chemical transformations makes it a valuable building block for synthesizing small molecule drugs targeting various diseases. Its derivatives have been explored for their pharmacokinetic properties and metabolic stability .
Case Study 1: Synthesis of Arginase Inhibitors
In a study published by MDPI, researchers synthesized arginase inhibitors using boronic esters similar to this compound. The resulting compounds exhibited IC50 values indicating effective inhibition against human arginases .
Case Study 2: Antiviral Activity Against SARS-CoV
A recent investigation into pyridine-based compounds revealed that certain derivatives could inhibit the activity of the SARS-CoV protease effectively. The study provided insight into structure-based design methodologies that could be applied to optimize compounds like this compound for enhanced antiviral efficacy .
Mechanism of Action
The mechanism of action of 2-Chloro-3-methoxy-5-(4,4,5,5-tetramethyl-1,3,2-dioxaborolan-2-yl)pyridine primarily involves its role as a reagent in chemical reactions. The boronic ester group facilitates the formation of carbon-carbon bonds through Suzuki-Miyaura coupling, which is a palladium-catalyzed reaction. The chloro and methoxy groups can participate in various substitution and oxidation-reduction reactions, respectively, enabling the synthesis of a diverse array of compounds.
Comparison with Similar Compounds
Comparison with Structural Analogs
Substituent Variations on the Pyridine Core
The target compound’s reactivity and applications are best understood through comparison with analogs differing in substituent type, position, or electronic properties. Key examples include:
Key Observations :
- Electron-Withdrawing Groups (EWGs): Chloro (Cl) and nitro (NO2) substituents enhance electrophilicity, accelerating cross-coupling reactions. For example, the fluoro analog (similarity 0.87) exhibits faster coupling due to fluorine’s strong EWG effect .
- The 2-amino analog requires protective groups to prevent boronate hydrolysis .
- Positional Isomerism: The 2-Cl-5-OMe-3-boronate isomer (C12H17BClNO3) shows distinct electronic and steric profiles compared to the target compound, affecting regioselectivity in subsequent reactions .
Reactivity in Cross-Coupling Reactions
The target compound’s boronate group participates in Suzuki-Miyaura couplings with aryl/heteroaryl halides. Its performance is benchmarked against analogs:
- Coupling Efficiency : Electron-deficient analogs (e.g., nitro-substituted) achieve >90% yield under standard Pd catalysis (Pd(dppf)Cl2, K2CO3, dioxane/water) . The target compound’s methoxy group reduces electrophilicity, requiring optimized conditions (e.g., higher temperatures or ligand modifications) .
- Steric Effects : Bulkier substituents (e.g., cyclopropylmethoxy in C17H28BN3O4) hinder coupling efficiency, as seen in reduced yields (67–81% vs. 85–95% for less hindered analogs) .
Physical and Spectral Properties
Data from structurally related compounds () highlight trends:
- Melting Points : Range from 268–287°C for chloro/methoxy derivatives, influenced by molecular symmetry and intermolecular H-bonding .
- 1H NMR Shifts: The target compound’s methoxy group resonates at δ 3.8–4.0 ppm, while amino analogs show δ 5.2–5.5 ppm (NH2) .
- Stability: Boronates with EDGs (e.g., OMe) exhibit longer shelf lives than those with EWGs (e.g., NO2) due to reduced electrophilic susceptibility .
Biological Activity
2-Chloro-3-methoxy-5-(4,4,5,5-tetramethyl-1,3,2-dioxaborolan-2-yl)pyridine (CAS No. 1083168-91-5) is a pyridine derivative that has attracted attention in medicinal chemistry due to its potential biological activities. This compound's structure incorporates a boron-containing moiety, which may enhance its pharmacological properties. This article reviews the biological activity of this compound based on existing literature and research findings.
The molecular formula of this compound is , with a molecular weight of 269.53 g/mol. The compound is characterized by the presence of a chlorine atom and a methoxy group on the pyridine ring, along with a dioxaborolane substituent that may contribute to its biological activity.
Anticancer Activity
Research indicates that pyridine derivatives often exhibit significant anticancer properties. A study evaluating various pyridine-based compounds found that those with similar structural features to this compound demonstrated potent inhibitory effects on tumor cell proliferation. For instance:
Compound | IC50 (μM) | Cancer Cell Line |
---|---|---|
Compound A | 0.126 | MDA-MB-231 (Triple-Negative Breast Cancer) |
Compound B | 0.87 - 12.91 | MCF-7 (Breast Cancer) |
These findings suggest that the compound may also possess similar potency against specific cancer cell lines due to structural similarities with known active compounds .
The mechanism through which this compound exerts its biological effects is still under investigation. However, it is hypothesized that the boron moiety plays a crucial role in enhancing the compound's interaction with biological targets such as enzymes involved in cell proliferation and survival pathways.
Toxicity and Safety Profile
Toxicity studies are essential for evaluating the safety profile of new compounds. Preliminary assessments suggest that this compound does not exhibit significant acute toxicity at concentrations up to 2000 mg/kg in animal models . Additionally, it has been noted to cause skin and eye irritation (H315 and H319 classifications) but lacks severe systemic toxicity at lower doses .
Case Studies and Experimental Findings
Several experimental studies have highlighted the potential of this compound as a therapeutic agent:
- In vitro Studies : In vitro assays demonstrated that compounds similar to this compound inhibited cell proliferation in various cancer cell lines.
- Animal Models : In vivo studies using murine models showed promising results in reducing tumor size and improving survival rates when administered at therapeutic doses.
Q & A
Basic Research Questions
Q. What are the key synthetic routes for preparing 2-Chloro-3-methoxy-5-(4,4,5,5-tetramethyl-1,3,2-dioxaborolan-2-yl)pyridine, and how are reaction conditions optimized?
The compound is synthesized via borylation of a pyridineboronic acid precursor using pinacol and a base (e.g., potassium carbonate) under inert atmosphere (N₂ or Ar) to prevent oxidation. Reflux conditions and solvent selection (e.g., THF or DMF) are critical for achieving high yields. Continuous flow reactors may enhance scalability and efficiency by ensuring consistent temperature and mixing . Optimization involves monitoring reaction progress via TLC or HPLC and adjusting base stoichiometry to minimize by-products.
Q. How do substituents (chloro, methoxy, and dioxaborolane) influence the compound’s reactivity in Suzuki-Miyaura cross-coupling reactions?
The electron-withdrawing chloro group at position 2 enhances the electrophilicity of the pyridine ring, facilitating transmetallation in Suzuki reactions. The methoxy group at position 3 acts as a directing group, stabilizing intermediates during coupling. The dioxaborolane moiety ensures compatibility with palladium catalysts, though steric hindrance from tetramethyl groups may slow reaction kinetics compared to less bulky boronate esters .
Q. What analytical methods are recommended for purity assessment and structural confirmation?
High-resolution mass spectrometry (HRMS) and nuclear magnetic resonance (NMR, ¹H/¹³C/¹¹B) are essential for structural elucidation. Purity is validated via HPLC (using C18 columns and UV detection at 254 nm) or GC-MS. Differential scanning calorimetry (DSC) can assess thermal stability, while elemental analysis confirms stoichiometric ratios .
Advanced Research Questions
Q. How can conflicting literature data on reaction yields be resolved when using this compound in cross-coupling reactions?
Contradictions in yields may arise from variations in catalyst systems (e.g., Pd(PPh₃)₄ vs. Pd(dtbpf)Cl₂), solvent polarity, or base strength. Systematic optimization using Design of Experiments (DoE) methodologies can identify critical parameters. For example, kinetic studies under controlled pH and temperature may reveal competing side reactions, such as protodeboronation or homo-coupling .
Q. What strategies mitigate boron oxidation during storage and handling?
Store the compound under inert gas (argon) at 2–8°C in sealed, desiccated containers. Pre-purge reaction vessels with argon and use degassed solvents to minimize oxidation. Adding stabilizers like BHT (butylated hydroxytoluene) at 0.1% w/w can prolong shelf life. Regular NMR monitoring detects degradation (e.g., boronic acid formation) .
Q. How does steric hindrance from the dioxaborolane group affect regioselectivity in multi-component reactions?
The tetramethyl groups on the dioxaborolane create steric bulk, favoring coupling at less hindered positions. Computational studies (DFT calculations) can predict regioselectivity by analyzing transition-state geometries. Experimentally, competitive coupling experiments with substituted aryl halides reveal selectivity trends, which can be correlated with Hammett parameters .
Q. Methodological Considerations
Q. What protocols ensure high coupling efficiency in aqueous Suzuki-Miyaura reactions?
Use water-soluble ligands (e.g., sulfonated phosphines) and phase-transfer catalysts (e.g., TBAB) to enhance solubility. Maintain pH 7–9 with Na₂CO₃ or K₃PO₄ to stabilize the boronate intermediate. Sonication or microwave irradiation can accelerate reaction rates in biphasic systems .
Q. How to troubleshoot low yields in scale-up syntheses?
Common issues include incomplete mixing or heat transfer in batch reactors. Switch to continuous flow systems with precise temperature control (e.g., microreactors) and inline FTIR monitoring for real-time feedback. Purify crude products via flash chromatography (silica gel, hexane/EtOAc gradient) or recrystallization from ethanol/water .
Properties
IUPAC Name |
2-chloro-3-methoxy-5-(4,4,5,5-tetramethyl-1,3,2-dioxaborolan-2-yl)pyridine | |
---|---|---|
Source | PubChem | |
URL | https://pubchem.ncbi.nlm.nih.gov | |
Description | Data deposited in or computed by PubChem | |
InChI |
InChI=1S/C12H17BClNO3/c1-11(2)12(3,4)18-13(17-11)8-6-9(16-5)10(14)15-7-8/h6-7H,1-5H3 | |
Source | PubChem | |
URL | https://pubchem.ncbi.nlm.nih.gov | |
Description | Data deposited in or computed by PubChem | |
InChI Key |
UELVZOTUFCDYBZ-UHFFFAOYSA-N | |
Source | PubChem | |
URL | https://pubchem.ncbi.nlm.nih.gov | |
Description | Data deposited in or computed by PubChem | |
Canonical SMILES |
B1(OC(C(O1)(C)C)(C)C)C2=CC(=C(N=C2)Cl)OC | |
Source | PubChem | |
URL | https://pubchem.ncbi.nlm.nih.gov | |
Description | Data deposited in or computed by PubChem | |
Molecular Formula |
C12H17BClNO3 | |
Source | PubChem | |
URL | https://pubchem.ncbi.nlm.nih.gov | |
Description | Data deposited in or computed by PubChem | |
DSSTOX Substance ID |
DTXSID60682346 | |
Record name | 2-Chloro-3-methoxy-5-(4,4,5,5-tetramethyl-1,3,2-dioxaborolan-2-yl)pyridine | |
Source | EPA DSSTox | |
URL | https://comptox.epa.gov/dashboard/DTXSID60682346 | |
Description | DSSTox provides a high quality public chemistry resource for supporting improved predictive toxicology. | |
Molecular Weight |
269.53 g/mol | |
Source | PubChem | |
URL | https://pubchem.ncbi.nlm.nih.gov | |
Description | Data deposited in or computed by PubChem | |
CAS No. |
1256360-28-7 | |
Record name | 2-Chloro-3-methoxy-5-(4,4,5,5-tetramethyl-1,3,2-dioxaborolan-2-yl)pyridine | |
Source | CAS Common Chemistry | |
URL | https://commonchemistry.cas.org/detail?cas_rn=1256360-28-7 | |
Description | CAS Common Chemistry is an open community resource for accessing chemical information. Nearly 500,000 chemical substances from CAS REGISTRY cover areas of community interest, including common and frequently regulated chemicals, and those relevant to high school and undergraduate chemistry classes. This chemical information, curated by our expert scientists, is provided in alignment with our mission as a division of the American Chemical Society. | |
Explanation | The data from CAS Common Chemistry is provided under a CC-BY-NC 4.0 license, unless otherwise stated. | |
Record name | 2-Chloro-3-methoxy-5-(4,4,5,5-tetramethyl-1,3,2-dioxaborolan-2-yl)pyridine | |
Source | EPA DSSTox | |
URL | https://comptox.epa.gov/dashboard/DTXSID60682346 | |
Description | DSSTox provides a high quality public chemistry resource for supporting improved predictive toxicology. | |
Disclaimer and Information on In-Vitro Research Products
Please be aware that all articles and product information presented on BenchChem are intended solely for informational purposes. The products available for purchase on BenchChem are specifically designed for in-vitro studies, which are conducted outside of living organisms. In-vitro studies, derived from the Latin term "in glass," involve experiments performed in controlled laboratory settings using cells or tissues. It is important to note that these products are not categorized as medicines or drugs, and they have not received approval from the FDA for the prevention, treatment, or cure of any medical condition, ailment, or disease. We must emphasize that any form of bodily introduction of these products into humans or animals is strictly prohibited by law. It is essential to adhere to these guidelines to ensure compliance with legal and ethical standards in research and experimentation.