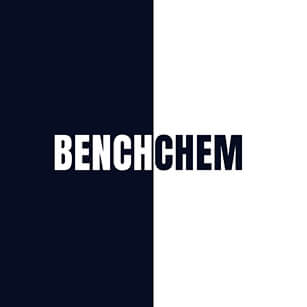
Bauxite
- Click on QUICK INQUIRY to receive a quote from our team of experts.
- With the quality product at a COMPETITIVE price, you can focus more on your research.
Overview
Description
Bauxite is a sedimentary rock composed primarily of aluminum hydroxide minerals, including gibbsite (γ-Al₂O₃·3H₂O), boehmite (γ-AlO(OH)), and diaspore (α-AlO(OH)), alongside impurities such as iron oxides (hematite, goethite), silica, and titanium dioxide . It forms through prolonged chemical weathering of aluminum-rich rocks in tropical or subtropical climates, resulting in residual accumulations of aluminum hydroxides.
Metallurgical-grade this compound, which accounts for ~85% of global production, is processed via the Bayer method to extract alumina (Al₂O₃), the precursor to aluminum metal . This compound is classified by application (abrasive, cement, refractory), with key deposits in Australia, Guinea, and Brazil . Its logging response includes high gamma, density, and neutron readings, and low resistivity, reflecting its porous, clastic structure .
Preparation Methods
Composition and Significance of Bauxite
This compound is a heterogeneous rock composed primarily of aluminum hydroxides (gibbsite, böhmite, diaspore), iron oxides (hematite, goethite), silicon dioxide (quartz, kaolinite), and titanium dioxide . The aluminum content varies between 30% and 60% by weight, necessitating purification to meet the >99% purity required for aluminum smelting . Deposits are classified based on dominant aluminum minerals: gibbsitic this compound (tropical regions) and böhmitic/diasporic this compound (subtropical zones) .
The Bayer Process: Industrial Framework
Ore Preparation
Raw this compound undergoes crushing and grinding to reduce particle size to <100 mesh (150 µm), enhancing surface area for subsequent reactions . Silica content, which interferes with caustic efficiency, is reduced from ~10% to <2% through size segregation, as finer fractions concentrate aluminum minerals . Modern facilities employ rod and ball mills to achieve optimal particle distribution, followed by slurry mixing in agitated tanks .
Table 1: Typical this compound Composition Pre- and Post-Beneficiation
Component | Raw this compound (%) | Processed this compound (%) |
---|---|---|
Al₂O₃ | 45–55 | 55–65 |
SiO₂ | 8–12 | 1–2 |
Fe₂O₃ | 15–25 | 15–25 |
TiO₂ | 2–4 | 2–4 |
Hydrothermal Digestion
Slurried this compound is mixed with concentrated sodium hydroxide (NaOH, 30–40% w/w) and heated to 150–250°C under pressures of 15–30 atm in tubular digesters . Amphoteric aluminum hydroxides dissolve as sodium aluminate:
Al(OH)3+NaOH→NaAlO2+2H2O
Böhmitic and diasporic ores require higher temperatures (220–250°C) due to their crystalline stability . Lime (CaO) is often added to mitigate silica interference by forming insoluble calcium silicates :
SiO2+2NaOH→Na2SiO3+H2O
Na2SiO3+CaO→CaSiO3↓+2NaOH
Residence times range from 30 minutes to 2 hours, with digestion efficiency exceeding 95% for gibbsitic ores .
Clarification and Red Mud Separation
The digested slurry is flash-cooled to 100°C, reducing pressure to atmospheric levels and precipitating dissolved impurities . Polyelectrolyte flocculants (e.g., starch derivatives) aggregate suspended solids, primarily iron oxides and silica residues (termed red mud), which settle in clarifiers . Countercurrent decantation in 6–8 stages recovers 90–95% of caustic soda, while red mud (1.5–2.5 tons per ton of alumina) is washed, filtered, and stored in this compound residue disposal areas (BRDAs) .
Precipitation of Aluminum Hydroxide
Sodium aluminate solution is seeded with gibbsite crystals (<10 µm) to initiate nucleation. Cooling to 50–70°C over 24–72 hours precipitates 50–60% of dissolved alumina as Al(OH)₃ :
NaAlO2+2H2O→Al(OH)3↓+NaOH
Seed recycling and supersaturation control optimize crystal growth, yielding particles of 75–150 µm suitable for calcination .
Calcination to Metallurgical-Grade Alumina
Aluminum hydroxide is calcined in rotary kilns or fluidized beds at 1,100–1,400°C to drive off chemically bound water :
2Al(OH)3ΔAl2O3+3H2O
Fluidized-bed calciners dominate modern installations due to 20–30% lower energy consumption compared to rotary kilns . The final product contains <0.1% SiO₂ and <0.02% Fe₂O₃, meeting specifications for electrolytic reduction .
Alternative and Emerging Processing Methods
Sintering Process for High-Silica Bauxites
Bauxites with >8% reactive silica undergo sintering with sodium carbonate (Na₂CO₃) at 1,200°C to form soluble sodium aluminate and insoluble calcium silicate :
Al2O3+Na2CO3→2NaAlO2+CO2
SiO2+CaCO3→CaSiO3+CO2
This method, though energy-intensive, achieves 85–90% alumina recovery from low-grade ores .
Acid Leaching for Non-Caustic Extraction
Hydrochloric or sulfuric acid leaching at 80–100°C extracts aluminum as Al³⁺ ions, bypassing silica dissolution :
Al(OH)3+3HCl→AlCl3+3H2O
Ion-exchange resins purify the leachate, but corrosion and acid regeneration costs limit commercial adoption .
Environmental and Operational Challenges
Red Mud Management
BRDAs store over 4 billion tons of red mud globally, posing risks of alkalinity (pH 10–13) and heavy metal leaching . Valorization efforts focus on using red mud in cement production (10–20% substitution) and rare earth element (REE) extraction, though economic viability remains limited .
Energy and Water Consumption
The Bayer process consumes 12–15 GJ/ton of alumina, primarily in digestion and calcination . Zero-liquid-discharge systems recycle 90% of process water, reducing freshwater intake to 2–3 m³/ton .
Chemical Reactions Analysis
Types of Reactions: Bauxite undergoes several types of chemical reactions, including:
Oxidation: The iron oxides in this compound can undergo oxidation reactions.
Reduction: In the Bayer process, the reduction of iron oxides to magnetite can occur under certain conditions.
Substitution: The aluminium hydroxides in this compound can undergo substitution reactions with other metal hydroxides.
Common Reagents and Conditions:
Sodium Hydroxide: Used in the Bayer process to dissolve aluminium hydroxides.
Lime: Sometimes added to precipitate silica as calcium silicate.
Major Products Formed:
Alumina (Aluminium Oxide): The primary product of the Bayer process.
Sodium Aluminate: An intermediate product in the Bayer process.
Scientific Research Applications
Metallurgical Applications
Aluminum Production
- Bauxite is the primary raw material for producing aluminum. The Bayer process is used to extract alumina from this compound, which is then reduced to aluminum through the Hall-Héroult process. Approximately 4 to 5 tonnes of this compound yield about 2 tonnes of alumina, which can produce nearly 1 tonne of aluminum .
Table 1: Aluminum Production from this compound
Material | Quantity |
---|---|
This compound | 4-5 tonnes |
Alumina | 2 tonnes |
Aluminum | ~1 tonne |
Refractory Industry
This compound is a key ingredient in the production of refractory materials due to its high melting point (1740-1820°C). High-alumina refractories (75-90% alumina) are used in blast furnaces and other high-temperature industrial processes .
Case Study: Refractory Applications
- In the steel industry, high-alumina bricks made from this compound are essential for lining furnaces, enhancing durability and thermal resistance.
Cement Industry
This compound is utilized in producing high-alumina cement, known for its rapid setting time and resistance to corrosion and acids. This cement is particularly useful in constructing tunnels and concrete piles .
Table 2: Properties of High-Alumina Cement
Property | Description |
---|---|
Setting Time | 3-4 hours |
Strength | Increases after 24 hours |
Resistance | Corrosion and sulfate resistant |
Chemical Industry
In the chemical sector, this compound serves as a source of aluminum compounds used in various applications, including water treatment and catalysts. It is processed into aluminum hydroxide, which is a precursor for aluminum-based coagulants used in water purification .
Environmental Applications
Recent research has highlighted the potential of this compound residue (a byproduct of alumina production) as a soil conditioner. This application not only helps in improving soil quality but also addresses waste management challenges associated with this compound processing .
Case Study: Soil Conditioning
- A study conducted by Hydro in Brazil demonstrated that this compound residue could enhance soil properties, promoting better water retention and nutrient utilization in agriculture.
Other Industrial Uses
This compound finds applications in various other industries:
Mechanism of Action
The mechanism by which bauxite exerts its effects is primarily through its chemical composition and reactivity. The aluminium hydroxides in this compound react with sodium hydroxide in the Bayer process to form soluble sodium aluminate, which is then precipitated as aluminium hydroxide and calcined to produce alumina . The molecular targets and pathways involved include the dissolution and precipitation reactions of aluminium hydroxides and the reduction of iron oxides .
Comparison with Similar Compounds
Comparison with Structurally Similar Aluminum Compounds
Bauxite’s primary minerals are compared below with other aluminum hydroxides/oxides:
Table 1: Structural and Industrial Comparison of Key Aluminum Compounds
Key Findings :
- Gibbsite is preferred in the Bayer process due to its lower energy requirements compared to boehmite and diaspore .
Comparison with Functionally Similar Aluminum Sources
This compound is contrasted with alternative alumina sources below:
Table 2: Functional Comparison of Aluminum Ores
Key Findings :
- This compound’s higher alumina content and established extraction methods make it economically superior to laterite and nepheline syenite .
- Alunite’s sulfate content complicates processing, limiting its industrial adoption .
Unique Properties of this compound and Residues
This compound residues (red mud) from the Bayer process contain rare earth elements (REEs) like lanthanum, scandium, and yttrium, immobilized by phases such as Al-hematite and sodalite . These residues also exhibit environmental utility:
- Soil Remediation : this compound residues increase phosphorus retention in sandy soils and adsorb toxic metals (e.g., lead, cadmium) via iron/aluminum oxides .
- Contrast with Zeolites : While zeolites have higher cation-exchange capacity, this compound residues offer cost advantages due to large-scale availability .
Table 3: REE Content in Sierra Leone this compound Residues
Sample | La (ppm) | Ce (ppm) | Y (ppm) | Sc (ppm) |
---|---|---|---|---|
BR1 | 320 | 650 | 110 | 85 |
BR2 | 290 | 600 | 95 | 72 |
Biological Activity
Bauxite, primarily composed of aluminum oxides, iron oxides, and silica, is the principal ore for aluminum production. Its biological activity, particularly in the context of environmental impacts and soil health post-mining, is a critical area of study. This article explores the biological characteristics of this compound, focusing on its effects on microbial communities, soil quality, and plant growth.
Composition and Properties of this compound
This compound mainly consists of:
- Aluminum Oxide (Al₂O₃) : 30-60%
- Iron Oxide (Fe₂O₃) : 10-30%
- Silica (SiO₂) : 5-20%
- Titanium Dioxide (TiO₂) : 1-5%
These components significantly influence the biological activity of this compound in soil environments.
Microbial Activity in this compound-Affected Soils
Research indicates that this compound mining alters the microbial community structure and function in surrounding soils. A study conducted in Romania assessed microbial abundance in post-bauxite mining land and revealed significant findings regarding microbial diversity and activity.
Microbial Diversity Analysis
The following table summarizes the microbial counts in various experimental variants from the study:
Experimental Variant | Heterotrophic Bacteria | Fungi | Ammonifying Bacteria | Nitrifying Bacteria | Denitrifying Bacteria |
---|---|---|---|---|---|
BlF0 | 7.09 | 6.57 | 2.56 | 3.44 | 2 |
BlF60 | 5.73 | 5.63 | 2.96 | 3.89 | 2.07 |
BlF120 | 7.25 | 6.30 | 2.30 | 2.38 | 1.56 |
Ns10%F0 | 5.23 | 6.47 | 2.74 | - | 2.83 |
Ns40%F0 | 6.54 | 5.82 | 2.78 | - | 2.30 |
This data indicates that different fertilization treatments affect microbial populations significantly, with higher fertilization correlating with increased heterotrophic bacteria and fungi counts .
Impact on Soil Quality
This compound mining can lead to soil degradation characterized by reduced nutrient availability and altered pH levels. The presence of high aluminum concentrations often results in acidic soils that restrict microbial activity and nutrient release necessary for plant growth . However, reclamation efforts using limestone can mitigate these effects by increasing soil alkalinity, promoting healthier microbial communities and facilitating nutrient cycling.
Case Studies on Reclamation Efforts
- Post-Mining Land Restoration : A study evaluated the restoration of this compound-mined lands by comparing reclaimed soils to unmined lands. Findings indicated that reclaimed areas, when treated with appropriate amendments, showed improved soil structure and increased microbial diversity compared to untreated mined soils .
- Health Impacts on Local Flora : Research has shown that plants growing in this compound-affected soils exhibit stunted growth due to nutrient deficiencies caused by high aluminum toxicity and low microbial activity . In contrast, reclaimed areas demonstrated significant recovery in plant health when enriched with organic matter.
Environmental Health Considerations
The biological activity of this compound also extends to its environmental health implications:
- Toxicity : High aluminum levels can be toxic to both flora and fauna, leading to reduced biodiversity in affected ecosystems.
- Dust Exposure : Workers involved in this compound mining are at risk for respiratory issues due to dust exposure, which contains fine particles from this compound processing . Studies have reported increased prevalence of respiratory symptoms among workers exposed to high levels of this compound dust.
Q & A
Basic Research Questions
Q. What methodologies are recommended for characterizing the mineralogical composition of bauxite samples?
- Methodological Answer : Use X-ray diffraction (XRD) to identify crystalline phases (e.g., gibbsite, boehmite) and X-ray fluorescence (XRF) for elemental composition. Supplement with scanning electron microscopy (SEM-EDS) for spatial distribution of minerals. Ensure sample preparation follows standardized protocols to avoid contamination .
- Example Table :
Technique | Parameters Analyzed | Detection Limits | Key Applications |
---|---|---|---|
XRD | Crystalline phases | >1% abundance | Mineral identification |
XRF | Major elements (Al, Fe, Si) | 10–100 ppm | Bulk composition |
SEM-EDS | Micro-scale elemental mapping | 0.1–1 wt% | Texture and mineral associations |
Q. How can researchers design statistically robust sampling strategies for heterogeneous this compound deposits?
- Methodological Answer : Implement grid-based or stratified random sampling to account for spatial variability. Use geostatistical tools (e.g., kriging) to interpolate data and validate representativeness. Sample size should adhere to Central Limit Theorem principles to minimize bias .
Q. What experimental parameters are critical for assessing Bayer process efficiency in laboratory settings?
- Methodological Answer : Control variables include temperature (200–300°C), NaOH concentration (140–300 g/L), and particle size (<100 µm). Use factorial design experiments to isolate effects of variables on alumina yield. Report reaction kinetics and residue composition for reproducibility .
Q. How should purity and phase transitions in calcined this compound-derived alumina be validated?
- Methodological Answer : Combine thermogravimetric analysis (TGA) with in-situ XRD to monitor phase changes (e.g., gibbsite → γ-Al₂O₃). Cross-validate purity using inductively coupled plasma mass spectrometry (ICP-MS) for trace elements .
Advanced Research Questions
Q. How can contradictions in reported alumina recovery rates from different this compound processing methods be resolved?
- Methodological Answer : Conduct meta-analysis to identify confounding variables (e.g., ore mineralogy, pretreatment methods). Use multivariate regression to quantify the impact of factors like Fe₂O₃/SiO₂ ratios on leaching efficiency. Replicate experiments under standardized conditions to isolate discrepancies .
Q. What advanced spectroscopic techniques enhance trace element analysis in this compound?
- Methodological Answer : Synchrotron-based X-ray absorption spectroscopy (XAS) provides speciation data for trace metals (e.g., Ga, V). Pair with laser ablation ICP-MS for spatially resolved trace element mapping at µm-scale resolution .
Q. How can geochemical and mineralogical data be integrated for predictive modeling of this compound formation?
- Methodological Answer : Apply machine learning algorithms (e.g., random forest) to correlate lithological logs with geochemical proxies (e.g., Al/Si ratios). Validate models using field data from known this compound profiles .
Q. What methodologies address discrepancies between laboratory and field-scale this compound beneficiation results?
- Methodological Answer : Conduct pilot-scale trials with continuous flow reactors to simulate industrial conditions. Use scaling laws (e.g., dimensionless numbers) to adjust parameters like residence time and agitation intensity. Compare energy consumption and yield metrics across scales .
Q. How can real-time monitoring improve the efficiency of this compound calcination processes?
- Methodological Answer : Deploy in-situ Fourier-transform infrared spectroscopy (FTIR) to track hydroxyl group removal during calcination. Integrate with process control systems to dynamically adjust temperature profiles .
Q. Methodological Best Practices
- Data Presentation : Follow journal guidelines (e.g., Beilstein Journal) to limit main text tables to critical findings. Use appendices for raw datasets and auxiliary analyses .
- Reproducibility : Document experimental protocols in detail, including reagent sources and instrument calibration procedures. Share synthetic pathways for novel compounds as supplementary information .
Properties
CAS No. |
1318-16-7 |
---|---|
Molecular Formula |
Al2H2O4 |
Molecular Weight |
119.977 g/mol |
IUPAC Name |
oxo(oxoalumanyloxy)alumane;hydrate |
InChI |
InChI=1S/2Al.H2O.3O/h;;1H2;;; |
InChI Key |
XXHQVTGCFGYKNL-UHFFFAOYSA-N |
SMILES |
O.O=[Al]O[Al]=O |
Canonical SMILES |
O.O=[Al]O[Al]=O |
physical_description |
Dark brown odorless solid; [Halliburton MSDS] |
Origin of Product |
United States |
Disclaimer and Information on In-Vitro Research Products
Please be aware that all articles and product information presented on BenchChem are intended solely for informational purposes. The products available for purchase on BenchChem are specifically designed for in-vitro studies, which are conducted outside of living organisms. In-vitro studies, derived from the Latin term "in glass," involve experiments performed in controlled laboratory settings using cells or tissues. It is important to note that these products are not categorized as medicines or drugs, and they have not received approval from the FDA for the prevention, treatment, or cure of any medical condition, ailment, or disease. We must emphasize that any form of bodily introduction of these products into humans or animals is strictly prohibited by law. It is essential to adhere to these guidelines to ensure compliance with legal and ethical standards in research and experimentation.