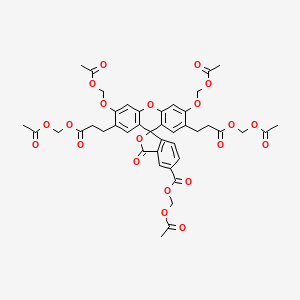
BCECF-acetoxymethyl
Overview
Description
BCECF-acetoxymethyl (BCECF-AM) is a cell-permeable, pH-sensitive fluorescent probe widely used to measure intracellular pH dynamics. Structurally, it is the acetoxymethyl ester derivative of 2',7'-bis-(2-carboxyethyl)-5-(and-6)-carboxyfluorescein (BCECF), a fluorescein-based dye. The esterification renders BCECF-AM membrane-permeable, allowing it to passively diffuse into cells. Once intracellular, endogenous esterases hydrolyze the acetoxymethyl groups, trapping the de-esterified BCECF within the cytoplasm or organelles .
BCECF exhibits ratiometric pH sensitivity, with dual excitation peaks at 440 nm (pH-insensitive) and 490 nm (pH-sensitive), and emission at ~530 nm. The ratio of fluorescence intensities at these excitation wavelengths correlates linearly with pH, minimizing artifacts from dye concentration, photobleaching, or uneven loading . Its pKa of ~7 makes it ideal for monitoring near-neutral pH ranges, such as cytosolic (pH 7.0–7.4) or vacuolar (pH 5.7–5.9) environments in plant and animal cells .
Key applications include:
Preparation Methods
Chemical Synthesis and Structural Optimization
The synthesis of BCECF-AM centers on modifying fluorescein derivatives with acetoxymethyl (AM) ether groups to enhance membrane permeability while maintaining pH-sensitive fluorescence.
Core Reaction Mechanism
BCECF-AM is synthesized through alkylation of 2',7'-bis(carboxyethyl)-5(6)-carboxyfluorescein with bromomethyl acetate. The reaction employs silver(I) oxide (Ag₂O) in acetonitrile under anhydrous conditions to minimize competing acylation reactions . This method yields a mixture of structural isomers (Forms II and III, Figure 2 in ), which are functionally equivalent upon intracellular hydrolysis.
Key synthetic parameters include:
-
Solvent System : Acetonitrile with molecular sieves to maintain fluorescein in its closed lactone form .
-
Base : Ag₂O facilitates Sₙ2 alkylation, achieving moderate yields (31% for fluorescein diacetoxymethyl ether) .
-
Temperature : Room temperature to prevent decomposition of AM groups .
Phase-Transfer Catalysis
Alternative synthesis routes using phase-transfer conditions (e.g., tetrabutylammonium bromide in dichloromethane/water) achieve comparable yields (31% for 4a ) but are less consistent for asymmetric derivatives like BCECF-AM .
Purification and Quality Control
Post-synthesis purification ensures the removal of unreacted precursors and byproducts, critical for minimizing background fluorescence.
High-Performance Liquid Chromatography (HPLC)
Commercial BCECF-AM batches (e.g., Enzo BML-144) are purified via reverse-phase HPLC to ≥95% purity . Specifications require the summation of all AM ester forms (Figure 2 in ) to exceed 90% of total product .
Table 1: Analytical Specifications of BCECF-AM
Parameter | Specification | Method | Source |
---|---|---|---|
Purity | ≥95% | HPLC | |
Fluorescence Spectrum | Excitation 505 nm | Fluorimetry | |
Solubility | Clear in DMSO | Visual | |
Moisture Sensitivity | Decomposes in H₂O | NMR/IR |
Protocol Optimization for Cellular Loading
BCECF-AM’s utility hinges on efficient intracellular delivery and esterase-mediated hydrolysis. Standardized protocols balance loading efficiency with cell viability.
Stock Solution Preparation
-
Concentration : 1–10 mM in anhydrous DMSO, stored at ≤–20°C .
-
Stability : Solutions remain viable for 6 months if protected from light and moisture .
Cell Loading Procedure
-
Cell Suspension : 4×10⁷ cells/mL in HEPES buffer (20 mM HEPES, 153 mM NaCl, 5 mM KCl, pH 7.4) .
-
AM Ester Addition : 1 mM BCECF-AM/DMSO diluted to 3 μM final concentration (1:300 v/v) .
-
Incubation : 37°C for 30 minutes, avoiding serum or amine-containing buffers .
-
Washing : Three cycles with HEPES saline to remove extracellular dye .
Table 2: Troubleshooting Common Loading Issues
Analytical Validation of Functional Performance
Post-preparation validation ensures BCECF-AM’s efficacy in pH sensing.
Ratiometric Calibration
Cell Viability Assays
Esterase-mediated hydrolysis of BCECF-AM serves as a viability marker; non-fluorescent AM converts to fluorescent acid only in live cells .
Supplier | Catalog No. | Purity | Unit Size | Price (USD) |
---|---|---|---|---|
Thermo Fisher | B1170 | >90% | 20×50 μg | 358.65 |
Dojindo | B031-10 | ≥85% | 5 mg | N/A |
Enzo | BML-144 | ≥95% | 5 mg | N/A |
Emerging Innovations and Limitations
Modified AM Esters
Recent work explores tert-butyloxycarbonyl (Boc) groups to enhance plasma stability, though cellular uptake efficiency remains inferior to AM .
Limitations in Multicellular Systems
Chemical Reactions Analysis
Types of Reactions
BCECF-acetoxymethyl primarily undergoes hydrolysis reactions. Upon entering the cell, intracellular esterases hydrolyze the acetoxymethyl ester groups, converting this compound into its fluorescent form, BCECF .
Common Reagents and Conditions
Hydrolysis: Intracellular esterases are the primary reagents that catalyze the hydrolysis of this compound. .
Major Products Formed
Scientific Research Applications
BCECF-acetoxymethyl is extensively used in various scientific research fields due to its unique properties:
Chemistry: Used as a pH indicator in various chemical assays and experiments.
Biology: Widely employed in cell biology to measure intracellular pH changes, study cell viability, and monitor cellular processes.
Medicine: Utilized in medical research to investigate cellular responses to different treatments and conditions.
Industry: Applied in the development of diagnostic tools and assays for monitoring cellular health and function
Mechanism of Action
BCECF-acetoxymethyl exerts its effects through a mechanism involving hydrolysis by intracellular esterases. Once inside the cell, the acetoxymethyl ester groups are cleaved, releasing the fluorescent BCECF. This fluorescent form can then be excited at specific wavelengths to emit fluorescence, which is used to measure intracellular pH. The molecular targets are primarily the intracellular esterases that catalyze the hydrolysis reaction .
Comparison with Similar Compounds
While BCECF-AM is specialized for pH sensing, other fluorescent probes share structural or functional similarities but differ in target analytes, sensitivity, or experimental utility. Below is a detailed comparison based on the provided evidence and related analogs:
Table 1: Comparison of BCECF-AM with Other Fluorescent Probes
Functional and Structural Insights
Target Specificity: BCECF-AM is uniquely optimized for pH due to its ratiometric design and pKa near physiological cytosolic pH. In contrast, H2DCF and diaminofluorescein detect ROS and NO, respectively, with emission intensity changes unrelated to pH .
Loading Mechanisms: All acetoxymethyl (AM) ester probes (BCECF-AM, H2DCF diacetate) rely on esterase hydrolysis for activation. However, BCECF-AM requires careful solvent controls (e.g., ethanol) to avoid artifacts from incomplete de-esterification .
Spectral Advantages :
- BCECF-AM’s dual-excitation ratiometry reduces variability caused by uneven dye distribution, unlike intensity-based probes like H2DCF . Fluorescein-AM, while pH-sensitive, lacks ratiometric capability and has a lower pKa (~6.4), limiting its utility in neutral pH environments .
Compartmentalization :
- BCECF-AM can accumulate in vacuoles or other organelles, necessitating validation of subcellular localization. This contrasts with cytosolic-localized probes like Fura-2 (Ca²⁺ indicator), which exhibit minimal organellar retention .
Research Findings and Experimental Considerations
- Plant Biology : BCECF-AM revealed vacuolar pH stabilization (~5.7–5.9) in Arabidopsis roots under nutrient stress, a finding critical for understanding ion homeostasis .
- Mammalian Systems : In HEK-293 cells, BCECF-AM enabled precise measurement of proton flux during osmotic swelling, with a permeability coefficient (Pf) of ~0.01 cm/s .
- Artifact Mitigation : Studies using BCECF-AM for Na+/H+ exchanger activity in NRK cells emphasized the need for parallel solvent controls to exclude dye-loading variability .
Limitations vs. Alternatives :
- While BCECF-AM excels in pH 6.5–7.5, carboxy-SNARF (pKa ~7.5) is preferable for alkaline environments. However, SNARF requires more complex calibration and is absent from the provided evidence.
- Non-ratiometric probes (e.g., H2DCF) remain popular for ROS but lack BCECF-AM’s quantitative precision for pH .
Biological Activity
BCECF-acetoxymethyl (BCECF-AM) is a widely utilized fluorescent probe, particularly known for its role in measuring intracellular pH (pH_i) across various biological systems. This article explores the biological activity of BCECF-AM, highlighting its mechanisms, applications, and relevant research findings.
BCECF-AM is an acetoxymethyl ester derivative of BCECF, which enhances its membrane permeability, allowing for non-invasive loading into cells. Upon entering the cell, intracellular esterases cleave the acetoxymethyl groups, converting it into the fluorescent form of BCECF. This transformation enables the compound to retain within the cell, making it suitable for various fluorescence-based assays .
Key Properties:
- Molecular Formula: C42H40O21
- Fluorescence Characteristics: BCECF exhibits pH-dependent fluorescence, where its emission intensity varies with changes in pH. This property is crucial for accurately measuring pH_i in living cells .
Applications in Biological Research
BCECF-AM has been employed in numerous studies to investigate cellular functions and dynamics, particularly regarding pH regulation. Below are some notable applications:
- Intracellular pH Measurement:
- High-Throughput Screening:
- Cell Viability and Cytotoxicity Assays:
Case Study 1: V-ATPase Inhibition
A study conducted on yeast cells demonstrated that BCECF-AM could effectively monitor changes in vacuolar pH due to V-ATPase inhibition. The fluorescence intensity was significantly higher in V-ATPase-deficient mutants compared to wild-type cells, indicating a more alkaline environment due to impaired proton transport .
Cell Type | Fluorescence Intensity | Vacuolar pH Change |
---|---|---|
Wild-Type | Baseline | Lower |
V-ATPase Mutant | Increased | Higher |
Case Study 2: pH Measurement in Rabbit Cortical Collecting Tubules
In another study using BCECF-AM, researchers found that apical incubation led to intense uptake in intercalated cells, revealing specific differences in pH_i between cell populations. The minority intercalated cells exhibited a pH_i of 7.34 ± 0.06 compared to 7.41 ± 0.05 in majority cells .
Limitations and Considerations
While BCECF-AM is a powerful tool for studying intracellular dynamics, certain limitations must be acknowledged:
- Phototoxicity: Prolonged excitation can lead to photobleaching and alterations in apparent pH_i readings due to changes in fluorescence sensitivity .
- Cell Type Variability: The efficiency of loading BCECF-AM can vary significantly among different cell types; for example, yeast cells often show poor uptake due to insufficient hydrolysis by intracellular esterases .
Q & A
Basic Research Questions
Q. What are the optimal protocols for loading BCECF-AM into plant or mammalian cells while minimizing compartmentalization artifacts?
BCECF-AM, a membrane-permeable ester, hydrolyzes intracellularly to release the pH-sensitive BCECF. To minimize vacuolar/lysosomal sequestration (common in plant root cells ):
- Use low dye concentrations (1–5 µM) and short incubation times (15–30 mins) at room temperature.
- Include pluronic acid (0.02%) to enhance dye solubility and ensure uniform cellular distribution.
- Validate loading efficiency via fluorescence microscopy, comparing cytosolic vs. vacuolar signals .
- Reference: Krebs et al. (2010) for Arabidopsis root tip protocols .
Q. How should researchers calibrate BCECF fluorescence ratios for accurate intracellular pH measurements?
Calibration requires a ratiometric approach (excitation: 440 nm/495 nm; emission: 535 nm):
- Generate a pH calibration curve using high-K⁺ buffers (pH 4.5–8.0) with nigericin (10 µM) to equilibrate intracellular and extracellular pH .
- Account for autofluorescence by measuring unloaded cells under identical conditions.
- Validate linearity using internal controls (e.g., NH₄Cl pulses for alkaline shifts) .
Advanced Research Questions
Q. How can cell type variability in BCECF-AM esterase activity confound pH measurements, and what controls are essential?
Esterase activity varies across cell types (e.g., mammalian vs. plant cells), affecting hydrolysis rates:
- Pre-test esterase activity using fluorescein diacetate (FDA) as a positive control.
- Use BCECF-free acid controls to confirm intracellular trapping vs. passive leakage .
- For low-activity cells (e.g., certain cancer lines), extend loading times or use modified AM esters .
Q. What methodologies resolve conflicting pH data from BCECF and alternative probes (e.g., SNARF-1) in the same experimental system?
Discrepancies arise from differences in pKa, subcellular localization, or photobleaching rates:
- Perform parallel measurements with BCECF and SNARF-1, using identical calibration buffers.
- Apply ionophores (e.g., monensin) to equalize organellar pH gradients.
- Analyze photostability: BCECF degrades 20% faster than SNARF-1 under continuous illumination; adjust imaging intervals accordingly .
Q. How can researchers design experiments to distinguish between cytosolic and organellar pH dynamics using BCECF-AM?
BCECF’s partial sequestration in vacuoles/lysosomes complicates cytosolic-specific readings:
- Use organelle-targeted pH probes (e.g., pHluorin for Golgi) alongside BCECF.
- Apply compartment-specific inhibitors (e.g., bafilomycin A1 for vacuolar H⁺-ATPase).
- Deconvolute signals via confocal microscopy with region-of-interest (ROI) analysis .
Q. What statistical approaches are recommended for analyzing time-dependent BCECF fluorescence data in live-cell imaging studies?
Time-series data require normalization and drift correction:
- Normalize ratios to baseline (F/F₀) to account for photobleaching.
- Apply moving-average smoothing or Gaussian filters to reduce noise.
- Use non-parametric tests (e.g., Wilcoxon for paired samples) if data are non-normal .
Q. Methodological Best Practices
Q. How should researchers address BCECF-AM batch variability in longitudinal studies?
- Obtain CAS-registered BCECF-AM (CAS 117123-51-8) from suppliers with lot-specific HPLC purity reports (>90%).
- Pre-test each batch in a standardized cell system (e.g., HEK293) with known pH perturbations.
- Store aliquots at -20°C under argon to prevent ester hydrolysis .
Q. What steps ensure reproducibility when publishing BCECF-based pH measurements?
Follow ICMJE and ACS Style Guide standards:
- Report dye concentration, loading time, calibration buffers, and instrumentation (e.g., filter sets, objective NA).
- Deposit raw fluorescence images and calibration curves in public repositories (e.g., Zenodo) with CC-BY licenses .
- Disclose conflicts (e.g., vendor collaborations) per journal policies .
Q. Troubleshooting Data Contradictions
Q. Why might BCECF report alkaline cytosolic pH in hypoxia studies despite metabolic acidosis expectations?
Potential artifacts include:
- Dye leakage: Verify membrane integrity with propidium iodide.
- ROS interference: Use antioxidants (e.g., Trolox) in imaging buffers.
- pH-independent quenching: Confirm ratio changes with NH₄Cl/NaAcid pulses .
Q. How to validate BCECF pH measurements when extracellular acidification (e.g., Warburg effect) complicates readings?
Properties
IUPAC Name |
acetyloxymethyl 3',6'-bis(acetyloxymethoxy)-2',7'-bis[3-(acetyloxymethoxy)-3-oxopropyl]-3-oxospiro[2-benzofuran-1,9'-xanthene]-5-carboxylate | |
---|---|---|
Source | PubChem | |
URL | https://pubchem.ncbi.nlm.nih.gov | |
Description | Data deposited in or computed by PubChem | |
InChI |
InChI=1S/C42H40O21/c1-22(43)52-17-57-34-15-36-32(13-27(34)7-10-38(48)59-19-54-24(3)45)42(31-9-6-29(12-30(31)41(51)63-42)40(50)61-21-56-26(5)47)33-14-28(8-11-39(49)60-20-55-25(4)46)35(16-37(33)62-36)58-18-53-23(2)44/h6,9,12-16H,7-8,10-11,17-21H2,1-5H3 | |
Source | PubChem | |
URL | https://pubchem.ncbi.nlm.nih.gov | |
Description | Data deposited in or computed by PubChem | |
InChI Key |
NTECHUXHORNEGZ-UHFFFAOYSA-N | |
Source | PubChem | |
URL | https://pubchem.ncbi.nlm.nih.gov | |
Description | Data deposited in or computed by PubChem | |
Canonical SMILES |
CC(=O)OCOC1=CC2=C(C=C1CCC(=O)OCOC(=O)C)C3(C4=C(C=C(C=C4)C(=O)OCOC(=O)C)C(=O)O3)C5=C(O2)C=C(C(=C5)CCC(=O)OCOC(=O)C)OCOC(=O)C | |
Source | PubChem | |
URL | https://pubchem.ncbi.nlm.nih.gov | |
Description | Data deposited in or computed by PubChem | |
Molecular Formula |
C42H40O21 | |
Source | PubChem | |
URL | https://pubchem.ncbi.nlm.nih.gov | |
Description | Data deposited in or computed by PubChem | |
DSSTOX Substance ID |
DTXSID90922429 | |
Record name | (Acetyloxy)methyl 3',6'-bis[(acetyloxy)methoxy]-2',7'-bis{3-[(acetyloxy)methoxy]-3-oxopropyl}-3-oxo-3H-spiro[2-benzofuran-1,9'-xanthene]-5-carboxylate | |
Source | EPA DSSTox | |
URL | https://comptox.epa.gov/dashboard/DTXSID90922429 | |
Description | DSSTox provides a high quality public chemistry resource for supporting improved predictive toxicology. | |
Molecular Weight |
880.8 g/mol | |
Source | PubChem | |
URL | https://pubchem.ncbi.nlm.nih.gov | |
Description | Data deposited in or computed by PubChem | |
CAS No. |
117464-70-7 | |
Record name | (Acetyloxy)methyl 3',6'-bis[(acetyloxy)methoxy]-2',7'-bis{3-[(acetyloxy)methoxy]-3-oxopropyl}-3-oxo-3H-spiro[2-benzofuran-1,9'-xanthene]-5-carboxylate | |
Source | EPA DSSTox | |
URL | https://comptox.epa.gov/dashboard/DTXSID90922429 | |
Description | DSSTox provides a high quality public chemistry resource for supporting improved predictive toxicology. | |
Record name | Spiro[isobenzofuran-1(3H),9'-[9H]xanthene]-2',7'-dipropanoic acid, 3',6'-bis(acetyloxy)-5(or 6)-[[(acetyloxy)methoxy]carbonyl]-3-oxo-, 2',7'-bis[(acetyloxy)methyl] ester | |
Source | European Chemicals Agency (ECHA) | |
URL | https://echa.europa.eu/substance-information/-/substanceinfo/100.109.830 | |
Description | The European Chemicals Agency (ECHA) is an agency of the European Union which is the driving force among regulatory authorities in implementing the EU's groundbreaking chemicals legislation for the benefit of human health and the environment as well as for innovation and competitiveness. | |
Explanation | Use of the information, documents and data from the ECHA website is subject to the terms and conditions of this Legal Notice, and subject to other binding limitations provided for under applicable law, the information, documents and data made available on the ECHA website may be reproduced, distributed and/or used, totally or in part, for non-commercial purposes provided that ECHA is acknowledged as the source: "Source: European Chemicals Agency, http://echa.europa.eu/". Such acknowledgement must be included in each copy of the material. ECHA permits and encourages organisations and individuals to create links to the ECHA website under the following cumulative conditions: Links can only be made to webpages that provide a link to the Legal Notice page. | |
Disclaimer and Information on In-Vitro Research Products
Please be aware that all articles and product information presented on BenchChem are intended solely for informational purposes. The products available for purchase on BenchChem are specifically designed for in-vitro studies, which are conducted outside of living organisms. In-vitro studies, derived from the Latin term "in glass," involve experiments performed in controlled laboratory settings using cells or tissues. It is important to note that these products are not categorized as medicines or drugs, and they have not received approval from the FDA for the prevention, treatment, or cure of any medical condition, ailment, or disease. We must emphasize that any form of bodily introduction of these products into humans or animals is strictly prohibited by law. It is essential to adhere to these guidelines to ensure compliance with legal and ethical standards in research and experimentation.