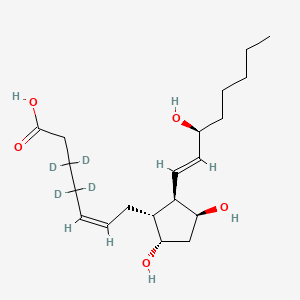
11beta-Prostaglandin F2alpha-d4
Overview
Description
11beta-Prostaglandin F2alpha-d4 (11β-PGF2α-d4) is a deuterium-labeled analog of endogenous 11β-PGF2α, a bioactive prostaglandin metabolite. Prostaglandins are lipid mediators derived from arachidonic acid, playing critical roles in inflammation, smooth muscle contraction, and reproductive processes . The deuterated form (d4) incorporates four deuterium atoms, typically replacing hydrogen at specific positions to enhance metabolic stability and enable precise quantification via mass spectrometry. While 11β-PGF2α is implicated in pathophysiological processes such as labor induction and cancer progression , 11β-PGF2α-d4 is primarily utilized as an internal standard in analytical workflows to measure endogenous prostaglandin levels in biological samples .
Preparation Methods
Synthesis Pathways for 11β-Prostaglandin F2α-d4
Enzymatic Synthesis via Aldo-Keto Reductases
The primary biosynthetic route for 11β-PGF2α involves the NADPH-dependent reduction of PGD2 catalyzed by aldo-keto reductase 1C3 (AKR1C3), also known as prostaglandin F synthase . This enzyme is expressed in human granulosa cells, liver, and lung tissues, where it facilitates the stereospecific conversion of PGD2 to 11β-PGF2α . The reaction mechanism proceeds via a ketoreduction at the C11 position, yielding the 11β-hydroxy configuration characteristic of this metabolite .
Bovine tissue studies demonstrate that 100,000 × g supernatants from liver, lung, and spleen exhibit high 11-ketoreductase activity, with kinetic parameters varying by tissue source. For example, liver homogenates show a Km of 6 µM for PGD2, while lung and spleen homogenates exhibit a Km of 120 µM . These differences suggest tissue-specific isoforms or cofactor availability influencing enzymatic efficiency.
Chemical Synthesis and Deuterium Labeling
11β-PGF2α-d4 is synthesized through selective deuterium incorporation at four hydrogen positions to serve as a stable isotope internal standard. The labeling process typically involves:
-
Protection of reactive groups : Hydroxyl and carboxyl moieties are protected using tert-butyldimethylsilyl (TBDMS) or methyl esters to prevent undesired side reactions.
-
Deuterium exchange : Catalytic deuteration under controlled pH and temperature conditions ensures site-specific labeling. For example, acidic deuteration (pH 2–3) in D2O facilitates H/D exchange at α-carbonyl positions .
-
Deprotection and purification : Final deprotection steps yield the deuterated product, which is purified via reversed-phase high-performance liquid chromatography (RP-HPLC) with UV detection at 210 nm .
The synthetic route is validated using liquid chromatography-tandem mass spectrometry (LC-MS/MS), confirming ≥98% isotopic purity and correct retention time alignment with non-deuterated 11β-PGF2α .
Enzymatic Preparation and Optimization
Tissue-Specific Enzyme Activity
Comparative studies of bovine tissues reveal significant variations in 11β-PGF2α synthesis capacity:
Tissue | 11-Ketoreductase Activity (nmol/h/mg protein) | Km for PGD2 (µM) |
---|---|---|
Liver | 51.09 | 6 |
Lung | 24.99 | 120 |
Spleen | 14.20 | 120 |
Heart | 3.61 | 15 |
Data derived from bovine tissue supernatants incubated with NADPH-generating systems .
Liver exhibits the highest activity due to abundant AKR1C3 expression, while lung and spleen rely on prostaglandin F synthetase isoforms with distinct kinetic properties . Immunotitration experiments confirm that >85% of lung and spleen reductase activity is attributable to prostaglandin F synthetase, whereas liver activity involves additional isoforms .
Recombinant Enzyme Systems
Recombinant AKR1C3 expressed in E. coli or mammalian cell lines provides a standardized enzyme source for large-scale 11β-PGF2α-d4 production. Optimization parameters include:
Reaction progress is monitored by LC-MS, with typical conversions of 70–80% PGD2 to 11β-PGF2α-d4 within 2 hours under optimized conditions .
Chemical Synthesis and Isotope Incorporation
Deuterium Labeling Strategy
The four deuterium atoms in 11β-PGF2α-d4 are introduced at non-labile positions to ensure metabolic stability. Key steps include:
-
C9 and C11 positions : Deuteration via Pd/C-catalyzed exchange in deuterated methanol (CD3OD) under H2 atmosphere .
-
Side-chain positions : Selective deuteration at C13 and C14 using deuterated arachidonic acid precursors .
Reaction conditions are tightly controlled to prevent racemization, with optical rotation measurements confirming retention of the 11β-configuration ([α]D²⁵ = +23.5° ± 0.5° in methanol) .
Purification and Characterization
Crude synthesis products are purified using:
-
Solid-phase extraction (SPE) : C18 cartridges preconditioned with methanol/water (80:20, v/v), eluted with acetonitrile:0.1% formic acid (95:5) .
-
Preparative HPLC : XBridge BEH C18 column (250 × 10 mm, 5 µm), isocratic elution with acetonitrile:10 mM ammonium acetate (65:35) at 4 mL/min .
Final characterization includes:
-
High-resolution MS : m/z 357.2147 [M-H]⁻ (calculated for C20H29D4O5: 357.2149)
-
NMR : ¹H NMR (600 MHz, CD3OD) δ 5.48–5.32 (m, 4H, olefinic), 4.12 (dd, J = 8.4, 4.2 Hz, 1H, C11-Hβ)
Analytical Validation and Quantification
LC-MS/MS Method Development
The Mayo Clinic Laboratories protocol employs:
-
Column : Kinetex C18 (100 × 2.1 mm, 2.6 µm)
-
Mobile phase : A) 0.1% formic acid, B) acetonitrile
Deuterated internal standard (11β-PGF2α-d4) is added to urine samples prior to SPE extraction, compensating for matrix effects and ionization efficiency variations .
Method Validation Parameters
Parameter | 11β-PGF2α-d4 | LTE4-d5 (Co-analyte) |
---|---|---|
Linearity (pg/mL) | 9.8–5000 | 9.8–5000 |
Intra-day RSD (%) | 4.0–7.4 | 6.8–14.9 |
Inter-day RSD (%) | 4.2–8.4 | 4.9–8.8 |
Recovery (%) | 70–116 | 61–99 |
Matrix Effect (%) | 69–106 | 87–126 |
Data from validation across nine urine lots using deuterated standards .
Enzymatic hydrolysis with β-glucuronidase (500 U/mL, 37°C, 2 hours) is critical for quantifying total 11β-PGF2α, as 40–60% exists as glucuronide conjugates in urine .
Chemical Reactions Analysis
11beta-Prostaglandin F2alpha-d4 undergoes various chemical reactions, including:
Oxidation: It can be oxidized to form different prostaglandin derivatives.
Reduction: Reduction reactions can modify its functional groups.
Substitution: Various substitution reactions can occur, especially at the hydroxyl groups.
Common reagents used in these reactions include oxidizing agents like potassium permanganate and reducing agents like lithium aluminum hydride. The major products formed depend on the specific reaction conditions and reagents used .
Scientific Research Applications
Pharmacological Research
Prostaglandin Receptor Studies
11beta-Prostaglandin F2alpha-d4 serves as a valuable tool in pharmacological studies investigating prostaglandin receptors. Its deuterated form allows for precise tracking in metabolic studies and receptor binding assays. Researchers utilize this compound to elucidate the signaling pathways mediated by prostaglandins, particularly in inflammatory responses and reproductive physiology .
Drug Development
The compound is also used in the development of new drugs targeting prostaglandin receptors. By understanding how this compound interacts with these receptors, researchers can design more effective therapeutics for conditions such as asthma, cardiovascular diseases, and reproductive disorders .
Clinical Diagnostics
Biomarker for Mast Cell Activation Syndrome
In clinical settings, this compound is utilized as a biomarker for mast cell activation syndrome (MCAS). Elevated levels of its metabolites, such as 2,3-dinor-11beta-prostaglandin F2alpha, are indicative of mast cell activation and have been correlated with symptoms like flushing and anaphylaxis . This application is crucial for diagnosing patients who may not exhibit typical markers like tryptase.
Urinary Excretion Studies
The quantification of this compound metabolites in urine samples provides insights into systemic mast cell disease and other related conditions. Liquid chromatography-tandem mass spectrometry (LC-MS/MS) is employed to measure these metabolites accurately, aiding in the assessment of patient risk profiles and treatment efficacy .
Research on Eicosanoids
Role in Inflammatory Diseases
Research indicates that this compound plays a significant role in understanding the pathophysiology of inflammatory diseases. Its analogs are studied for their potential to modulate inflammatory responses through various pathways involving eicosanoids. This research contributes to developing anti-inflammatory therapies and understanding chronic conditions such as arthritis and cardiovascular diseases .
Data Table: Summary of Applications
Application Area | Specific Use | Methodology/Technique |
---|---|---|
Pharmacological Research | Prostaglandin receptor studies | Binding assays, metabolic tracking |
Drug Development | Therapeutic design targeting prostaglandin receptors | Pharmacological profiling |
Clinical Diagnostics | Biomarker for mast cell activation syndrome | Urinary metabolite quantification |
Eicosanoid Research | Understanding inflammatory disease mechanisms | Eicosanoid pathway analysis |
Case Studies
- Mast Cell Activation Syndrome : A study demonstrated that patients with MCAS showed significantly elevated levels of urinary 2,3-dinor-11beta-prostaglandin F2alpha compared to healthy controls. This finding supports its use as a diagnostic marker for MCAS .
- Inflammatory Response Modulation : Research involving animal models indicated that administration of this compound could attenuate inflammatory responses in models of asthma, highlighting its potential therapeutic applications .
- Prostaglandin Receptor Interaction : A pharmacological study assessed the binding affinity of this compound to various prostaglandin receptors, revealing its high affinity for FP receptors and suggesting its utility in drug development targeting these pathways .
Mechanism of Action
The mechanism of action of 11beta-Prostaglandin F2alpha-d4 involves binding to the prostaglandin F2alpha receptor. This binding triggers a cascade of intracellular events, including the activation of G-proteins and subsequent signaling pathways. These pathways regulate various physiological processes such as inflammation, smooth muscle contraction, and reproductive functions .
Comparison with Similar Compounds
Structural Analogs and Isotopologues
The table below highlights key structural and functional differences between 11β-PGF2α-d4 and related prostaglandins:
Receptor Binding and Signaling
- 11β-PGF2α : Binds the FP receptor (PTGFR) with high affinity, activating intracellular calcium mobilization and PKC pathways to regulate cortisol synthesis and uterine contraction .
- 9α,11β-PGF2 : Exhibits overlapping activity with 11β-PGF2α but is distinctively elevated in aspirin-induced asthma, suggesting a role in mast-cell activation .
- PGF2α (Dinoprost): The parent compound shows stronger FP receptor activation compared to 11β-PGF2α, making it a preferred therapeutic agent .
- 11β-PGF2α-d4: While structurally identical to 11β-PGF2α, its deuterium labeling minimizes metabolic degradation, allowing accurate measurement of endogenous levels without interfering with receptor activity .
Metabolic Stability and Analytical Utility
- Deuterated Analogs (e.g., 11β-PGF2α-d4, PGE2-d4): Serve as stable isotope-labeled standards in mass spectrometry. For example, 11β-PGF2α-d4 co-elutes with endogenous 11β-PGF2α, correcting for matrix effects and ion suppression during LC-MS/MS analysis .
- Non-Deuterated Analogs (e.g., 15-keto-PGF2α, 9α,11β-PGF2): Rapidly metabolized in vivo, limiting their utility in long-term studies without stabilization agents .
Research and Clinical Relevance
- 11β-PGF2α-d4 : Critical for quantifying prostaglandins in studies exploring cancer biology (e.g., breast cancer Slug expression ) and reproductive physiology .
- 9α,11β-PGF2 : Identified as a biomarker in aspirin-exacerbated respiratory disease, with omalizumab therapy shown to reduce its overproduction .
- 15-keto-PGF2α : Used to assess oxidative stress in chronic inflammatory conditions due to its stability as a terminal metabolite .
Key Research Findings
11β-PGF2α in Labor Induction : 11β-PGF2α enhances cortisol production via 11β-HSD1 activation in chorion trophoblast cells, creating a feed-forward loop that amplifies prostaglandin synthesis during parturition .
Analytical Workflows : Deuterated standards like 11β-PGF2α-d4 enable precise measurement of prostaglandins in conditions such as overactive bladder and asthma .
Cancer Signaling: Non-deuterated 11β-PGF2α stimulates Slug expression in breast cancer via AKR1C3-mediated synthesis, highlighting its pro-metastatic role .
Biological Activity
11beta-Prostaglandin F2alpha-d4 (11β-PGF2α-d4) is a deuterated analog of the naturally occurring prostaglandin F2alpha (PGF2α). Prostaglandins are lipid compounds that play crucial roles in various physiological processes, including inflammation, reproduction, and vascular function. This article explores the biological activity of 11β-PGF2α-d4, focusing on its mechanisms of action, effects on different biological systems, and relevant research findings.
11β-PGF2α-d4 primarily exerts its effects through the activation of specific prostaglandin receptors, particularly the FP receptor. Upon binding to the FP receptor, it initiates a cascade of intracellular signaling pathways that influence cellular functions such as proliferation, apoptosis, and differentiation.
Key Pathways
- ERK and CREB Phosphorylation : In breast cancer cell lines expressing FP receptors, treatment with 11β-PGF2α-d4 has been shown to phosphorylate extracellular signal-regulated kinase (ERK) and cAMP response element-binding protein (CREB), leading to increased expression of Slug, a transcription factor associated with epithelial-mesenchymal transition (EMT) and cancer progression .
- Smooth Muscle Contraction : 11β-PGF2α-d4 induces contraction in various smooth muscle tissues, including myometrial and vascular smooth muscle. This effect is mediated through calcium mobilization and activation of myosin light chain kinase (MLCK) pathways .
Biological Activities
The biological activities of 11β-PGF2α-d4 encompass several physiological and pathological processes:
- Reproductive Physiology : It plays a significant role in luteolysis, facilitating the regression of the corpus luteum in females. This is critical for regulating menstrual cycles and reproductive health .
- Inflammation : As a pro-inflammatory mediator, it contributes to the inflammatory response by promoting vasodilation and increasing vascular permeability. It can also modulate immune cell activity .
- Cancer Biology : The compound has been implicated in cancer progression, particularly in breast cancer. Its interaction with FP receptors can enhance cell survival and resistance to chemotherapy .
Research Findings
Recent studies have highlighted various aspects of 11β-PGF2α-d4's biological activity:
- Breast Cancer Studies : In vitro studies using MCF-7 breast cancer cells demonstrated that 11β-PGF2α-d4 promotes cell survival through FP receptor activation. The correlation between FP receptor expression and poor clinical outcomes underscores its potential as a therapeutic target .
- Mast Cell Activation : Elevated levels of urinary 2,3-Dinor-11beta-prostaglandin F2alpha (a metabolite of 11β-PGF2α-d4) have been linked to systemic mastocytosis. This suggests that monitoring its levels could aid in diagnosing mast cell disorders .
- Vascular Effects : The compound has been shown to induce vasoconstriction in certain vascular beds, which may have implications for conditions such as hypertension or cardiovascular diseases .
Data Table: Biological Effects of this compound
Case Study 1: Breast Cancer
A study involving breast cancer patients examined the expression levels of FP receptors and their correlation with clinical outcomes. Patients with higher FP receptor expression showed increased tumor aggressiveness and decreased chemosensitivity when treated with standard therapies.
Case Study 2: Systemic Mastocytosis
In patients diagnosed with systemic mastocytosis, elevated levels of urinary 2,3-Dinor-11beta-prostaglandin F2alpha were observed. This finding was utilized as a biomarker for disease diagnosis alongside other mast cell mediators .
Q & A
Basic Research Questions
Q. What are the standard protocols for using 11β-Prostaglandin F2α-d4 as an internal standard in mass spectrometry (MS)-based quantification?
- Methodological Answer : Deuterated analogs like 11β-Prostaglandin F2α-d4 are used to correct for matrix effects and ion suppression in MS. Prepare a calibration curve by spiking known concentrations of the analyte into biological matrices (e.g., plasma, urine) alongside a fixed amount of the deuterated standard. Quantify via peak area ratios (analyte vs. internal standard) and validate using precision (CV <15%) and accuracy (85–115% recovery) criteria. Ensure chromatographic separation to avoid isotopic interference .
Q. How can researchers validate the specificity of 11β-Prostaglandin F2α-d4 in complex biological samples?
- Methodological Answer : Perform selectivity tests by analyzing blank matrices from ≥6 sources to confirm the absence of endogenous interference at the analyte’s retention time. Cross-validate with alternative detection methods (e.g., ELISA or LC-MS/MS with different ionization modes) to rule out matrix-specific biases. Use stable isotope dilution to enhance specificity, as deuterated standards co-elute with analytes but are distinguishable via mass shifts .
Q. What are the critical storage conditions for 11β-Prostaglandin F2α-d4 to ensure stability?
- Methodological Answer : Store lyophilized standards at −80°C under inert gas (e.g., argon) to prevent oxidation. For working solutions, use organic solvents (e.g., methanol) at −20°C and avoid freeze-thaw cycles >3×. Validate stability under intended storage conditions via repeated analysis over 2–4 weeks and compare degradation rates against freshly prepared standards .
Advanced Research Questions
Q. How should researchers resolve discrepancies between observed and expected concentrations of 11β-Prostaglandin F2α-d4 in longitudinal studies?
- Methodological Answer : Investigate potential sources of variability:
- Pre-analytical factors : Check sample collection protocols (e.g., anticoagulants, time-to-processing) and storage conditions.
- Instrumental drift : Recalibrate MS systems daily and use quality control (QC) samples interspersed with study samples.
- Statistical analysis : Apply mixed-effects models to account for intra- and inter-subject variability. Cross-check findings with orthogonal assays (e.g., immunoassays) to confirm trends .
Q. What strategies optimize the detection of low-abundance 11β-Prostaglandin F2α-d4 in lipidomic profiling?
- Methodological Answer : Enhance sensitivity via:
- Derivatization : Use pentafluorobenzyl esters or other electron-capturing groups to improve ionization efficiency.
- Microextraction : Solid-phase microextraction (SPME) or liquid-liquid extraction (LLE) with high recovery solvents (e.g., methyl tert-butyl ether).
- Data-independent acquisition (DIA) : Use MS/MSALL or SWATH to capture fragment ions of co-eluting lipids, reducing false negatives .
Q. How can contradictions between in vitro and in vivo data involving 11β-Prostaglandin F2α-d4 be addressed?
- Methodological Answer :
- Dose-response alignment : Ensure in vitro doses reflect physiologically relevant concentrations (e.g., nM–μM ranges).
- Temporal dynamics : Compare time-course data (e.g., prostaglandin half-life in cell media vs. plasma).
- Pathway context : Integrate metabolomic or transcriptomic datasets to identify compensatory mechanisms in vivo. Use knockout models (e.g., COX-1/2 inhibitors) to isolate specific pathways .
Q. What ethical and reporting standards apply when publishing data on 11β-Prostaglandin F2α-d4 in clinical research?
- Methodological Answer :
- Ethical compliance : Obtain institutional review board (IRB) approval for human studies and document informed consent. Adhere to FAIR principles (Findable, Accessible, Interoperable, Reusable) for data sharing.
- Transparency : Disclose all analytical parameters (e.g., LOD, LOQ, CVs) and raw data in supplementary materials. Follow CONSORT guidelines for randomized trials or STROBE for observational studies .
Q. Methodological Notes
- Data Presentation : Use tables to summarize validation parameters (e.g., linearity, precision) and figures for chromatographic traces or dose-response curves. Avoid duplicating data in text and visuals .
- Conflict Resolution : For contradictory findings, employ triangulation (e.g., combine MS, ELISA, and functional assays) and meta-analysis to identify consensus patterns .
Properties
IUPAC Name |
(Z)-3,3,4,4-tetradeuterio-7-[(1R,2R,3S,5S)-3,5-dihydroxy-2-[(E,3S)-3-hydroxyoct-1-enyl]cyclopentyl]hept-5-enoic acid | |
---|---|---|
Source | PubChem | |
URL | https://pubchem.ncbi.nlm.nih.gov | |
Description | Data deposited in or computed by PubChem | |
InChI |
InChI=1S/C20H34O5/c1-2-3-6-9-15(21)12-13-17-16(18(22)14-19(17)23)10-7-4-5-8-11-20(24)25/h4,7,12-13,15-19,21-23H,2-3,5-6,8-11,14H2,1H3,(H,24,25)/b7-4-,13-12+/t15-,16+,17+,18-,19-/m0/s1/i5D2,8D2 | |
Source | PubChem | |
URL | https://pubchem.ncbi.nlm.nih.gov | |
Description | Data deposited in or computed by PubChem | |
InChI Key |
PXGPLTODNUVGFL-SGSFECARSA-N | |
Source | PubChem | |
URL | https://pubchem.ncbi.nlm.nih.gov | |
Description | Data deposited in or computed by PubChem | |
Canonical SMILES |
CCCCCC(C=CC1C(CC(C1CC=CCCCC(=O)O)O)O)O | |
Source | PubChem | |
URL | https://pubchem.ncbi.nlm.nih.gov | |
Description | Data deposited in or computed by PubChem | |
Isomeric SMILES |
[2H]C([2H])(CC(=O)O)C([2H])([2H])/C=C\C[C@H]1[C@H](C[C@@H]([C@@H]1/C=C/[C@H](CCCCC)O)O)O | |
Source | PubChem | |
URL | https://pubchem.ncbi.nlm.nih.gov | |
Description | Data deposited in or computed by PubChem | |
Molecular Formula |
C20H34O5 | |
Source | PubChem | |
URL | https://pubchem.ncbi.nlm.nih.gov | |
Description | Data deposited in or computed by PubChem | |
Molecular Weight |
358.5 g/mol | |
Source | PubChem | |
URL | https://pubchem.ncbi.nlm.nih.gov | |
Description | Data deposited in or computed by PubChem | |
Retrosynthesis Analysis
AI-Powered Synthesis Planning: Our tool employs the Template_relevance Pistachio, Template_relevance Bkms_metabolic, Template_relevance Pistachio_ringbreaker, Template_relevance Reaxys, Template_relevance Reaxys_biocatalysis model, leveraging a vast database of chemical reactions to predict feasible synthetic routes.
One-Step Synthesis Focus: Specifically designed for one-step synthesis, it provides concise and direct routes for your target compounds, streamlining the synthesis process.
Accurate Predictions: Utilizing the extensive PISTACHIO, BKMS_METABOLIC, PISTACHIO_RINGBREAKER, REAXYS, REAXYS_BIOCATALYSIS database, our tool offers high-accuracy predictions, reflecting the latest in chemical research and data.
Strategy Settings
Precursor scoring | Relevance Heuristic |
---|---|
Min. plausibility | 0.01 |
Model | Template_relevance |
Template Set | Pistachio/Bkms_metabolic/Pistachio_ringbreaker/Reaxys/Reaxys_biocatalysis |
Top-N result to add to graph | 6 |
Feasible Synthetic Routes
Disclaimer and Information on In-Vitro Research Products
Please be aware that all articles and product information presented on BenchChem are intended solely for informational purposes. The products available for purchase on BenchChem are specifically designed for in-vitro studies, which are conducted outside of living organisms. In-vitro studies, derived from the Latin term "in glass," involve experiments performed in controlled laboratory settings using cells or tissues. It is important to note that these products are not categorized as medicines or drugs, and they have not received approval from the FDA for the prevention, treatment, or cure of any medical condition, ailment, or disease. We must emphasize that any form of bodily introduction of these products into humans or animals is strictly prohibited by law. It is essential to adhere to these guidelines to ensure compliance with legal and ethical standards in research and experimentation.