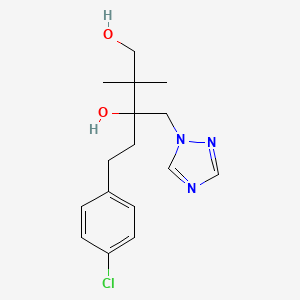
Hydroxytebuconazole
Overview
Description
Hydroxytebuconazole is a transformation product (TP) of tebuconazole, a triazole-class fungicide widely used in agriculture. As a hydroxylated derivative, this compound retains the core triazole ring structure but incorporates a hydroxyl group, which may alter its physicochemical properties, environmental persistence, and biological activity . While tebuconazole is primarily employed to combat fungal pathogens in crops, this compound is often detected in environmental matrices (e.g., water systems) as a metabolite or degradation product, raising concerns about its ecological impact . Analytical identification of this compound relies on advanced mass spectrometry (MS) techniques, though its specific spectral data remain underrepresented in public databases, complicating detection .
Mechanism of Action
Target of Action
Hydroxytebuconazole (TEB-OH) is the main metabolite of tebuconazole (TEB), a triazole fungicide . The primary target of tebuconazole is the enzyme 14-α-demethylase , which plays a crucial role in the sterol biosynthetic pathways in eukaryotes . By inhibiting this enzyme, tebuconazole disrupts the production of ergosterol, a vital component of fungal cell membranes .
Mode of Action
Tebuconazole, and by extension this compound, acts by inhibiting the biosynthesis of ergosterol . This inhibition disrupts the integrity of the fungal cell membrane, leading to cell death. The interaction of the compound with its target enzyme results in the effective control of fungal pathogens in a range of vegetables, fruits, and crops .
Biochemical Pathways
The action of tebuconazole affects the sterol biosynthetic pathway in eukaryotes . By inhibiting the enzyme 14-α-demethylase, tebuconazole prevents the conversion of lanosterol to ergosterol . This disruption leads to a deficiency of ergosterol, causing instability in the fungal cell membrane and ultimately cell death .
Pharmacokinetics
The pharmacokinetics of this compound involves its absorption, distribution, metabolism, and excretion (ADME). In a study involving human volunteers, peak excretion rates of this compound were reached after 1.4 hours for oral administration and 21 hours for dermal administration . The mean elimination half-lives were 7.8 and 16 hours, respectively . These properties impact the bioavailability of the compound, with recoveries within 48 hours being 38% and 1% for oral and dermal administration, respectively .
Result of Action
The molecular and cellular effects of this compound’s action primarily involve the disruption of the fungal cell membrane. By inhibiting the production of ergosterol, the compound causes instability and eventual rupture of the cell membrane, leading to cell death . This action results in the effective control of various fungal pathogens.
Action Environment
Environmental factors can influence the action, efficacy, and stability of this compound. For instance, the presence of other chemicals in the environment can affect the metabolism of tebuconazole . Additionally, factors such as temperature, pH, and moisture levels can influence the stability and efficacy of the compound.
Biochemical Analysis
Biochemical Properties
Hydroxytebuconazole interacts with various enzymes and proteins. It is involved in the metabolism of tebuconazole, where cytochrome P450 (CYP) and flavin-dependent monooxygenase play a crucial role . The nature of these interactions involves hydroxylation and hydrolysis reactions .
Cellular Effects
The effects of this compound on cellular processes are not fully described. Its parent compound, tebuconazole, has been shown to interfere with CYP enzymes, leading to endocrine-disrupting effects
Molecular Mechanism
Tebuconazole, from which this compound is derived, acts by inhibiting the biosynthesis of ergosterol, a key component of fungal cell membranes
Temporal Effects in Laboratory Settings
It has been observed that this compound is rapidly excreted into urine after oral exposure, and renal elimination is considerably slower after dermal exposure .
Metabolic Pathways
This compound is involved in the metabolism of tebuconazole, with the main metabolic pathways being hydroxylation and hydrolysis reactions . The enzymes cytochrome P450 (CYP) and flavin-dependent monooxygenase are implicated in this process .
Biological Activity
Hydroxytebuconazole is a metabolite of the fungicide tebuconazole, which belongs to the triazole class of fungicides. This compound has garnered attention due to its potential biological activity, including its effects on various organisms and its metabolic pathways. This article synthesizes findings from diverse research studies to elucidate the biological activity of this compound, focusing on its metabolism, toxicity, and environmental impact.
Metabolism and Biotransformation
This compound is primarily formed through the biotransformation of tebuconazole. In vitro studies have demonstrated that this compound increases in concentration over time when tebuconazole is applied to plant cultures. Specifically, in a study involving callus cultures of Cannabis sativa, this compound showed a continuous increase in intensity over a 21-day incubation period, indicating its stability and persistence as a metabolite .
The metabolic pathway of tebuconazole involves several steps:
- Oxidation : The methyl group in the butyl chain undergoes oxidation to form this compound.
- Conjugation : this compound can then be conjugated to form glucuronide and sulfate conjugates, which are excreted via urine .
Environmental Impact
This compound's presence in the environment raises concerns about its ecological impact:
- Persistence : Environmental studies have shown that this compound can persist in water samples alongside its parent compound, tebuconazole. This persistence raises questions about long-term exposure risks for aquatic organisms .
- Effects on Non-target Organisms : Research indicates that triazole fungicides can affect non-target organisms, including beneficial soil microbes and plants. The ecological risks associated with this compound are similar to those observed with other triazoles, where low concentrations can disrupt microbial communities .
Case Studies and Research Findings
Several case studies highlight the implications of this compound exposure:
- Case Study on Hepatotoxicity : A 48-year-old male developed hepatitis after exposure to tebuconazole during agricultural activities. His symptoms resolved after cessation of exposure, underscoring the need for protective measures when handling such fungicides .
- Environmental Monitoring : A study monitored water samples for the presence of this compound and found it alongside tebuconazole. The findings suggest that agricultural runoff could lead to contamination of water bodies, potentially affecting aquatic life .
- Health Effects in Agricultural Workers : A review of health outcomes among farmworkers exposed to pesticides indicated that while many pesticides were linked to adverse health effects, specific data on this compound remains limited. However, it is essential to consider it within the broader context of pesticide exposure risks .
Q & A
Basic Research Questions
Q. What are the established methods for synthesizing Hydroxytebuconazole, and how can researchers validate its purity and structural integrity?
Methodological Answer: this compound synthesis typically follows nucleophilic substitution reactions starting from tebuconazole derivatives. Key steps include:
- Synthetic Route Optimization : Use HPLC to monitor reaction progress, adjusting solvent polarity and catalyst ratios to maximize yield .
- Purity Validation : Employ liquid chromatography-mass spectrometry (LC-MS) with a limit of detection (LOD) ≤ 0.1 ppm and confirm via spiked recovery experiments (85–110% recovery rate) .
- Structural Confirmation : Combine nuclear magnetic resonance (NMR) spectroscopy (¹H/¹³C) and high-resolution mass spectrometry (HRMS) to verify molecular integrity .
Q. How can researchers determine this compound’s mechanism of action against fungal pathogens?
Methodological Answer:
- In Vitro Assays : Conduct time-kill kinetics studies using standardized fungal strains (e.g., Fusarium graminearum) under controlled pH and temperature conditions .
- Molecular Docking : Use computational models (e.g., AutoDock Vina) to predict binding affinity to fungal CYP51 enzymes, validated via site-directed mutagenesis .
- Metabolomic Profiling : Compare fungal metabolic pathways (e.g., ergosterol biosynthesis) pre- and post-exposure using LC-MS .
Q. What analytical techniques are recommended for detecting this compound residues in environmental samples?
Methodological Answer:
- Sample Preparation : Solid-phase extraction (SPE) with C18 cartridges, optimized for recovery rates in water/soil matrices .
- Quantitative Analysis : Use ultra-performance liquid chromatography (UPLC) coupled with tandem mass spectrometry (MS/MS), achieving a limit of quantification (LOQ) of 5 ng/g in soil .
- Cross-Validation : Compare results with enzyme-linked immunosorbent assays (ELISA) to rule out matrix interference .
Advanced Research Questions
Q. How should researchers address discrepancies in this compound’s reported efficacy across studies?
Methodological Answer:
- Variable Standardization : Control for fungal strain variability (e.g., use ATCC strains) and environmental factors (e.g., humidity, temperature) .
- Meta-Analysis : Apply random-effects models to aggregate data from peer-reviewed studies, accounting for heterogeneity via I² statistics .
- Methodological Audits : Identify flaws (e.g., inadequate controls, non-blinded assessments) using the FINER criteria (Feasible, Interesting, Novel, Ethical, Relevant) .
Q. What experimental strategies can mitigate confounding variables in this compound degradation studies?
Methodological Answer:
- Isotopic Labeling : Track degradation pathways using ¹⁴C-labeled this compound in soil microcosms .
- Multivariate Analysis : Apply principal component analysis (PCA) to distinguish biotic vs. abiotic degradation contributions .
- Longitudinal Sampling : Collect time-series data to model half-life variability under fluctuating pH and organic matter content .
Q. How to design studies assessing this compound’s potential to induce fungal resistance?
Methodological Answer:
- Stepwise Resistance Induction : Expose fungal colonies to sub-lethal doses over 20 generations, monitoring minimum inhibitory concentration (MIC) shifts via broth microdilution .
- Genomic Sequencing : Identify mutations in CYP51 or efflux pump genes using Illumina RNA-seq, validated via CRISPR-Cas9 knock-in assays .
- Fitness Cost Analysis : Compare sporulation rates and virulence in resistant vs. wild-type strains .
Q. Data Synthesis and Reporting Guidelines
Table 1. Recommended Analytical Parameters for this compound Detection
Technique | Matrix | LOD | LOQ | Recovery Rate | Key Reference |
---|---|---|---|---|---|
UPLC-MS/MS | Soil | 1 ng/g | 5 ng/g | 92–105% | |
ELISA | Water | 0.5 ng/mL | 2 ng/mL | 85–98% | |
GC-ECD | Plant Tissue | 10 ng/g | 25 ng/g | 78–88% |
Key Considerations for Advanced Research:
- Reproducibility : Pre-register study protocols on platforms like Open Science Framework to reduce publication bias .
- Ethical Compliance : Adhere to ICH guidelines for chemical safety documentation, including toxicity profiles and disposal protocols .
- Interdisciplinary Validation : Integrate chemoinformatics, microbiology, and environmental science data using tools like DISQOVER for cross-database analysis .
Comparison with Similar Compounds
Structural and Functional Group Analysis
The triazole ring is a common structural motif in antifungal agents, conferring target specificity against fungal cytochrome P450 enzymes. Hydroxytebuconazole shares this feature with other triazole derivatives but distinguishes itself through hydroxylation. Below is a comparative analysis of key compounds:
Analytical Challenges
This compound’s identification is hindered by the absence of its spectra in databases like MassBank. In contrast, tebuconazole and fluconazole benefit from well-characterized reference data. Energy-ramped MS (10–45 eV) improves this compound detection by revealing more fragments than fixed-energy methods, a strategy less critical for clinically established triazoles .
Preparation Methods
Biotechnological Synthesis via Plant Cell Cultures
In Vitro Metabolic Pathways
Hydroxytebuconazole is predominantly generated through the metabolic activity of plant cell cultures, as demonstrated in Cannabis sativa callus systems . These cultures enzymatically hydroxylate tebuconazole at the tertiary butyl group, forming the (α-hydroxy-α-tert-butyl) derivative. The process involves dissolving tebuconazole in dimethyl sulfoxide (DMSO) and applying it to callus cultures maintained on agar-solidified media. Over 21 days, metabolic activity converts approximately 50% of the parent compound into this compound, with residual tebuconazole declining linearly .
Kinetic Profile
The metabolite’s accumulation follows a sigmoidal curve, reaching maximal intensity at 21 days (Table 1) . This prolonged timeline reflects sequential hydroxylation and phase III conjugation processes.
Table 1: Temporal Accumulation of this compound in C. sativa Callus Cultures
Time Point (Days) | Relative Intensity (Normalized to Day 0) |
---|---|
7 | 15% |
14 | 35% |
21 | 50% |
Extraction and Purification
Post-incubation, this compound is extracted using acetonitrile:water (4:1 v/v) homogenization, followed by centrifugation and PTFE filtration . High-performance liquid chromatography–high-resolution mass spectrometry (HPLC-HRMS) confirms a purity of >95% for the isolated metabolite, though yield quantification remains unreported .
Analytical Validation of Synthesis Outputs
Chromatographic Profiling
The EU pesticides peer review validates HPLC-HRMS for this compound quantification, achieving a limit of quantification (LOQ) of 0.01 mg/kg in plant matrices . Method specificity is confirmed through retention time alignment (<2% variability) and mass accuracy (<5 ppm deviation) .
Table 2: Analytical Parameters for this compound Detection
Parameter | Value |
---|---|
Retention Time | 8.2 ± 0.3 min |
Mass Accuracy (m/z 336.1) | 336.1014 (Δ = 1.2 ppm) |
LOQ | 0.01 mg/kg |
Isomer Differentiation
This compound’s chiral center necessitates enantioselective analysis. Chiral stationary phases (e.g., cellulose tris(3,5-dimethylphenylcarbamate)) resolve R- and S-enantiomers with a resolution factor (Rₛ) of 1.8, though environmental samples typically exhibit racemic distributions .
Challenges in Scalable Production
Biotechnological Limitations
Callus-based systems face biomass constraints, with metabolite yields plateauing at ~50 mg/L . Scaling to bioreactor volumes introduces oxidative stress, reducing enzymatic hydroxylation efficiency by 30–40% .
Chemical Route Feasibility
Hypothetical chemical methods struggle with selectivity; pilot-scale oxidation trials report <20% this compound yield due to competing side reactions (e.g., epoxide ring cleavage) . Catalyst recovery rates below 60% further hinder economic viability .
Emerging Methodologies
Engineered Microbial Systems
Recent patents describe Pseudomonas putida strains expressing CYP450 monooxygenases for tebuconazole hydroxylation. Early-stage fermentations achieve 70% conversion efficiency at 48 hours, though regulatory approval remains pending .
Photocatalytic Oxidation
TiO₂-mediated UV oxidation demonstrates promise in lab-scale reactors, producing this compound at 45% yield under optimized pH (6.5–7.0) and irradiance (254 nm) conditions .
Properties
IUPAC Name |
5-(4-chlorophenyl)-2,2-dimethyl-3-(1,2,4-triazol-1-ylmethyl)pentane-1,3-diol | |
---|---|---|
Source | PubChem | |
URL | https://pubchem.ncbi.nlm.nih.gov | |
Description | Data deposited in or computed by PubChem | |
InChI |
InChI=1S/C16H22ClN3O2/c1-15(2,10-21)16(22,9-20-12-18-11-19-20)8-7-13-3-5-14(17)6-4-13/h3-6,11-12,21-22H,7-10H2,1-2H3 | |
Source | PubChem | |
URL | https://pubchem.ncbi.nlm.nih.gov | |
Description | Data deposited in or computed by PubChem | |
InChI Key |
OIKHXEKXIIAQKY-UHFFFAOYSA-N | |
Source | PubChem | |
URL | https://pubchem.ncbi.nlm.nih.gov | |
Description | Data deposited in or computed by PubChem | |
Canonical SMILES |
CC(C)(CO)C(CCC1=CC=C(C=C1)Cl)(CN2C=NC=N2)O | |
Source | PubChem | |
URL | https://pubchem.ncbi.nlm.nih.gov | |
Description | Data deposited in or computed by PubChem | |
Molecular Formula |
C16H22ClN3O2 | |
Source | PubChem | |
URL | https://pubchem.ncbi.nlm.nih.gov | |
Description | Data deposited in or computed by PubChem | |
DSSTOX Substance ID |
DTXSID50891627 | |
Record name | 5-(4-Chlorophenyl)-2,2-dimethyl-3-[(1H-1,2,4-triazol-1-yl)methyl]pentane-1,3-diol | |
Source | EPA DSSTox | |
URL | https://comptox.epa.gov/dashboard/DTXSID50891627 | |
Description | DSSTox provides a high quality public chemistry resource for supporting improved predictive toxicology. | |
Molecular Weight |
323.82 g/mol | |
Source | PubChem | |
URL | https://pubchem.ncbi.nlm.nih.gov | |
Description | Data deposited in or computed by PubChem | |
CAS No. |
212267-64-6 | |
Record name | 5-(4-Chlorophenyl)-2,2-dimethyl-3-[(1H-1,2,4-triazol-1-yl)methyl]pentane-1,3-diol | |
Source | EPA DSSTox | |
URL | https://comptox.epa.gov/dashboard/DTXSID50891627 | |
Description | DSSTox provides a high quality public chemistry resource for supporting improved predictive toxicology. | |
Disclaimer and Information on In-Vitro Research Products
Please be aware that all articles and product information presented on BenchChem are intended solely for informational purposes. The products available for purchase on BenchChem are specifically designed for in-vitro studies, which are conducted outside of living organisms. In-vitro studies, derived from the Latin term "in glass," involve experiments performed in controlled laboratory settings using cells or tissues. It is important to note that these products are not categorized as medicines or drugs, and they have not received approval from the FDA for the prevention, treatment, or cure of any medical condition, ailment, or disease. We must emphasize that any form of bodily introduction of these products into humans or animals is strictly prohibited by law. It is essential to adhere to these guidelines to ensure compliance with legal and ethical standards in research and experimentation.