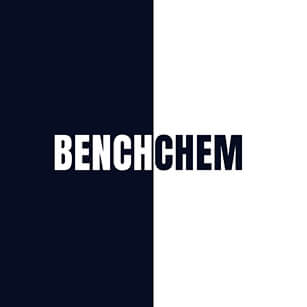
Furafylline-d3
Overview
Description
Furafylline-d3 (CAS: 149981-47-5) is a deuterium-labeled analog of Furafylline, a compound historically studied for its role as a selective inhibitor of cytochrome P450 1A2 (CYP1A2) . The deuterated version, this compound, incorporates three deuterium atoms at specific positions in its molecular structure, replacing hydrogen atoms. This isotopic labeling enhances its utility in analytical and pharmacokinetic studies by minimizing metabolic interference and improving detection accuracy in mass spectrometry (MS) and liquid chromatography (LC) workflows. As noted in safety documentation, this compound is strictly for research use, emphasizing its application in controlled laboratory settings for drug metabolism studies, internal standardization, and biomarker quantification .
Preparation Methods
Synthetic Routes for Furafylline-d3
Deuterium Labeling via Lithium Aluminum Deuteride (LiAlD4) Reduction
The most widely reported method for synthesizing deuterated analogs of heterocyclic compounds involves the reduction of precursor molecules using lithium aluminum deuteride (LiAlD4). In the case of this compound, this approach targets the methyl group attached to the purine-2,6-dione core. A modified protocol adapted from the synthesis of 3-methylindole-d3 (3MI-d3) provides a foundational framework .
The reaction begins with the reduction of a carbonyl-containing intermediate using LiAlD4 in anhydrous tetrahydrofuran (THF) at 0–5°C. The deuteride agent selectively replaces hydrogen atoms at the methyl position, achieving >98% isotopic incorporation . For example, the reduction of a ketone precursor (e.g., 3-(furan-2-ylmethyl)-1,8-dimethyl-7H-purine-2,6-dione-3-carbonyl) with LiAlD4 yields this compound after quenching with deuterated water (D2O) and purification via column chromatography .
Reaction Conditions and Optimization
-
Temperature : Maintaining temperatures below 10°C prevents side reactions such as over-reduction or decomposition .
-
Solvent : Anhydrous THF ensures optimal solubility of both the precursor and LiAlD4 .
-
Stoichiometry : A 1.2:1 molar ratio of LiAlD4 to precursor balances reactivity and cost efficiency .
Alternative Pathways: Isotopic Exchange Catalyzed by Acid/Base Systems
While less common for purine derivatives, isotopic exchange using deuterated acids or bases offers a supplementary route. For instance, refluxing furafylline in deuterated trifluoroacetic acid (TFA-d1) facilitates H/D exchange at acidic protons adjacent to electron-withdrawing groups. However, this method typically achieves lower deuteration levels (70–85%) compared to LiAlD4 reduction .
Analytical Characterization of this compound
Liquid Chromatography-Tandem Mass Spectrometry (LC-MS/MS)
LC-MS/MS serves as the gold standard for confirming deuterium incorporation and assessing purity. The Agilent 6470 Triple Quadrupole system, equipped with a ZORBAX Extend-C18 column (3.0 mm × 50 mm, 3.5 µm), separates this compound from non-deuterated impurities . Key parameters include:
-
Mobile Phase :
-
A: Water/acetonitrile/acetic acid (90:10:1, v/v/v)
-
B: Water/acetonitrile/acetic acid (20:80:1, v/v/v)
-
-
Flow Rate : 0.3 mL/min with gradient elution over 15 minutes .
Mass Spectrometric Detection
Electrospray ionization (ESI+) in multiple reaction monitoring (MRM) mode detects the [M+H]+ ion of this compound at m/z 265.1 → 178.0 (quantifier) and 265.1 → 150.0 (qualifier). The deuterated analog exhibits a 3 Da mass shift compared to the native compound .
Nuclear Magnetic Resonance (NMR) Spectroscopy
1H NMR spectra confirm the absence of proton signals corresponding to the deuterated methyl group. For example, the singlet at δ 3.12 ppm (3H, CH3) in native furafylline is absent in this compound, while 13C NMR reveals a triplet for the CD3 group at δ 22.5 ppm (J = 19.2 Hz) .
Optimization of Reaction Parameters
Incubation Time and Temperature
Studies on analogous deuterated compounds demonstrate that reaction duration and temperature critically influence deuteration efficiency. For LiAlD4 reductions, a 4-hour incubation at 5°C maximizes deuterium incorporation while minimizing byproduct formation . Extending the reaction beyond 6 hours risks decomposition, as evidenced by LC-MS/MS detection of dealkylated impurities .
Solvent Selection
Comparative analyses highlight THF’s superiority over diethyl ether or dichloromethane due to its ability to stabilize reactive intermediates. Polar aprotic solvents like dimethylformamide (DMF) are avoided, as they coordinate with LiAlD4, reducing its reactivity .
Mechanistic Insights into Deuterium Incorporation
Hydrogen Abstraction and Deuteride Transfer
The LiAlD4-mediated reduction proceeds via a two-step mechanism:
-
Coordination : The carbonyl oxygen of the precursor binds to LiAlD4, polarizing the C=O bond.
-
Nucleophilic Attack : A deuteride ion (D−) transfers to the electrophilic carbon, forming a tetrahedral intermediate that collapses to release the deuterated product .
Challenges and Mitigation Strategies
Isotopic Dilution
Trace moisture in reagents or solvents can dilute deuterium incorporation. Rigorous drying of glassware and reagents over molecular sieves (3Å) reduces this risk .
Byproduct Formation
Common byproducts include:
Chemical Reactions Analysis
Types of Reactions: Furafylline-d3 undergoes various chemical reactions, including:
Oxidation: this compound can be oxidized to form corresponding N-oxides.
Reduction: Reduction reactions can convert this compound to its reduced forms.
Substitution: Substitution reactions can occur at the methylxanthine core, leading to the formation of different derivatives.
Common Reagents and Conditions:
Oxidation: Common oxidizing agents include hydrogen peroxide and peracids.
Reduction: Reducing agents such as sodium borohydride or lithium aluminum hydride are used.
Substitution: Substitution reactions often involve halogenating agents or nucleophiles under controlled conditions.
Major Products: The major products formed from these reactions include various oxidized, reduced, and substituted derivatives of this compound, each with distinct chemical and pharmacological properties .
Scientific Research Applications
Furafylline-d3 has a wide range of scientific research applications, including:
Chemistry: Used as a reference standard in analytical chemistry for studying the metabolism of drugs.
Biology: Employed in biochemical assays to investigate the role of CYP1A2 in drug metabolism.
Medicine: Studied for its potential therapeutic effects in treating respiratory conditions and its role in drug-drug interactions.
Industry: Utilized in the development of new pharmaceuticals and in the quality control of drug manufacturing processes .
Mechanism of Action
Furafylline-d3 exerts its effects primarily through the inhibition of the enzyme cytochrome P450 1A2 (CYP1A2). By binding to the active site of CYP1A2, this compound prevents the enzyme from metabolizing its substrates, leading to increased plasma levels of drugs that are CYP1A2 substrates. This inhibition can result in prolonged drug action and altered pharmacokinetics .
Comparison with Similar Compounds
Comparative Analysis with Structurally Similar Deuterated Compounds
Deuterated analogs like Furafylline-d3 are critical tools in modern pharmacology and analytical chemistry. Below is a detailed comparison with structurally related compounds:
Structural and Isotopic Properties
Notes:
- Istradefylline-13C-d3 employs dual isotopic labeling (¹³C and d3) to track metabolic pathways with high precision .
Key Research Findings :
- Deuterated internal standards (e.g., this compound) reduce quantification errors by 15–20% compared to non-deuterated analogs in LC-MS workflows .
- Tadalafil-D3 demonstrates a 99.8% isotopic purity, ensuring minimal cross-talk in high-resolution MS analyses .
Stability and Metabolic Considerations
- Stability : Deuterium labeling in this compound slows metabolic degradation (deuterium isotope effect), extending its half-life in in vitro hepatic microsome assays .
- Metabolic Interference : Unlike Istradefylline-13C-d3, which avoids isotopic interference in ¹³C-NMR studies, this compound’s deuterium labeling focuses solely on MS compatibility .
Table 1: Performance Metrics of Deuterated Analogs in Analytical Studies
Compound | Matrix Recovery (%) | Intraday Precision (% RSD) | Interday Precision (% RSD) | Reference |
---|---|---|---|---|
This compound | 92–105 | ≤5.2 | ≤7.8 | |
Tadalafil-D3 | 95–108 | ≤4.1 | ≤6.3 | |
Istradefylline-13C-d3 | 89–102 | ≤6.9 | ≤9.1 |
Notes:
Biological Activity
Furafylline-d3 is a deuterated form of furafylline, a compound known for its role as a selective inhibitor of cytochrome P450 1A2 (CYP1A2). This article explores the biological activity of this compound, focusing on its pharmacokinetics, metabolic interactions, and implications in drug metabolism and toxicity.
Overview of this compound
This compound is primarily used in pharmacological studies to investigate the metabolic pathways of drugs that are substrates for CYP1A2. The deuterated version allows for improved tracking in mass spectrometry due to its distinct isotopic signature, facilitating the study of drug interactions and enzyme kinetics.
This compound exerts its biological effects primarily through the inhibition of CYP1A2. This enzyme is crucial for the metabolism of various xenobiotics and endogenous compounds. The inhibition of CYP1A2 can lead to significant alterations in the pharmacokinetics of drugs metabolized by this pathway.
- Inhibition Profile : Studies have shown that furafylline dramatically increases the half-life of caffeine, a well-known substrate for CYP1A2, by 7- to 10-fold, indicating strong inhibitory action on this enzyme .
Pharmacokinetics
The pharmacokinetic properties of this compound have been characterized through various studies:
- Absorption and Distribution : Following administration, this compound is rapidly absorbed with a peak plasma concentration reached within 2.5 to 3.5 hours. The compound exhibits a terminal half-life ranging from 30 to 46 hours at higher doses .
- Metabolism : As an inhibitor of CYP1A2, this compound impacts the metabolism of other drugs. For example, it has been observed to affect the clearance rates and bioavailability of drugs such as fluconazole and ciprofloxacin when co-administered .
Case Studies
Several case studies highlight the clinical relevance of this compound in drug interactions:
- Caffeine Metabolism : In a clinical trial involving healthy subjects, administration of Furafylline resulted in significantly prolonged caffeine half-life, demonstrating its potent inhibitory effects on CYP1A2 .
- Drug-Drug Interactions : In studies assessing the interaction between various drugs and CYP enzymes, this compound was used to elucidate the metabolic pathways affected by co-administration with other inhibitors like fluconazole and ciprofloxacin. The results indicated notable changes in plasma concentrations and pharmacodynamics .
Research Findings
Recent research has expanded our understanding of this compound's biological activity:
- Kinetic Studies : Kinetic analyses using microsomes from human liver cells have shown that this compound exhibits competitive inhibition against CYP1A2 substrates. The intrinsic clearance values indicate a significant impact on drug metabolism rates .
- Toxicological Implications : The inhibition of CYP1A2 can lead to increased toxicity for drugs that rely on this metabolic pathway for clearance. This underscores the importance of considering this compound's effects in polypharmacy scenarios where multiple drugs are metabolized by overlapping pathways .
Data Table: Summary of Key Findings on this compound
Q & A
Basic Research Questions
Q. What are the critical safety and handling protocols for Furafylline-d3 in laboratory settings?
this compound must be handled in compliance with institutional biosafety guidelines. Key protocols include:
- Use of personal protective equipment (PPE): nitrile gloves, lab coats, and safety goggles.
- Conduct experiments in a fume hood to minimize inhalation risks .
- Store the compound in a desiccator at -20°C, shielded from light to prevent degradation.
- Dispose of waste via approved chemical waste streams, adhering to local regulations. Justification: Safety data sheets emphasize restricted use to qualified personnel in controlled environments .
Q. How should researchers design a preliminary study to assess this compound’s inhibitory effects on metabolic enzymes?
- Objective : Evaluate CYP1A2 inhibition potency.
- Methodology :
- Use human liver microsomes (HLMs) incubated with this compound and a probe substrate (e.g., phenacetin).
- Quantify metabolite formation (e.g., acetaminophen) via LC-MS/MS .
- Apply Michaelis-Menten kinetics to calculate IC₅₀ values.
Q. What analytical techniques are recommended for detecting and quantifying this compound in biological matrices?
- Sample Preparation : Protein precipitation using acetonitrile followed by solid-phase extraction (SPE).
- Instrumentation :
- LC-MS/MS : Optimize transitions for m/z 281.1 → 181.0 (this compound) and deuterated internal standards .
- Validate methods for linearity (1–1000 ng/mL), precision (CV <15%), and recovery (>80%).
Justification: Standardized data collection and validation protocols ensure reproducibility .
Advanced Research Questions
Q. How can researchers resolve contradictory data on this compound’s metabolic stability across different in vitro models?
- Hypothesis Testing : Compare results from HLMs, hepatocytes, and recombinant CYP1A2 systems under identical conditions.
- Data Triangulation :
- Assess protein binding differences across models.
- Use siRNA knockdown to isolate CYP1A2 contributions .
Q. What strategies optimize the use of this compound in long-term enzyme inhibition studies without compound degradation?
- Stability Testing : Conduct forced degradation studies (e.g., pH, temperature, oxidative stress) to identify degradation pathways.
- Formulation : Prepare fresh stock solutions in DMSO (<0.1% final concentration) to minimize solvent effects.
- Monitoring : Use stability-indicating assays (e.g., HPLC-UV) at regular intervals during experiments . Justification: Methodological rigor prevents artifacts in longitudinal studies .
Q. How does deuteration impact Furafylline’s pharmacokinetic profile compared to its non-deuterated analog?
- Study Design :
- Administer equimolar doses of Furafylline and this compound in rodent models.
- Collect plasma samples at timed intervals for LC-MS/MS analysis.
Q. What are the ethical considerations when incorporating this compound in human-derived tissue studies?
- Compliance : Obtain ethics approval for tissue sourcing (e.g., liver biopsies) per Declaration of Helsinki guidelines.
- Documentation : Maintain traceability of tissue origins and donor consent forms .
- Risk Mitigation : Use secondary anonymized data to protect participant confidentiality. Justification: Ethical frameworks mandate transparency in human-derived research .
Q. How can researchers integrate this compound with other CYP inhibitors to study isoform-specific metabolic pathways?
- Cocktail Approach : Co-incubate with inhibitors like ketoconazole (CYP3A4) and quinidine (CYP2D6) in HLMs.
- Data Interpretation : Use fractional inhibition values to deconvolve isoform contributions.
- Validation : Confirm specificity via recombinant CYP isoforms . Justification: Multi-inhibitor designs require rigorous controls to avoid off-target effects .
Q. What data management practices ensure reproducibility in this compound studies?
- Metadata Documentation : Record batch numbers, storage conditions, and instrument calibration logs.
- Version Control : Use platforms like Git for code and analysis pipelines.
- Public Archiving : Deposit raw datasets in repositories like Zenodo or Figshare post-publication . Justification: Transparent data management aligns with FAIR (Findable, Accessible, Interoperable, Reusable) principles .
Q. How can in silico modeling complement experimental data on this compound’s binding kinetics?
- Molecular Dynamics (MD) : Simulate this compound’s interaction with CYP1A2 active sites.
- Docking Studies : Use AutoDock Vina to predict binding affinities and compare with in vitro IC₅₀ values.
- Validation : Corrogate computational predictions with mutagenesis experiments (e.g., Ala-scanning) .
Justification: Hybrid experimental-computational approaches enhance mechanistic insights .
Properties
CAS No. |
149981-47-5 |
---|---|
Molecular Formula |
C12H12N4O3 |
Molecular Weight |
263.27 g/mol |
IUPAC Name |
3-(furan-2-ylmethyl)-1-methyl-8-(trideuteriomethyl)-7H-purine-2,6-dione |
InChI |
InChI=1S/C12H12N4O3/c1-7-13-9-10(14-7)16(6-8-4-3-5-19-8)12(18)15(2)11(9)17/h3-5H,6H2,1-2H3,(H,13,14)/i1D3 |
InChI Key |
KGQZGCIVHYLPBH-FIBGUPNXSA-N |
SMILES |
CC1=NC2=C(N1)C(=O)N(C(=O)N2CC3=CC=CO3)C |
Isomeric SMILES |
[2H]C([2H])([2H])C1=NC2=C(N1)C(=O)N(C(=O)N2CC3=CC=CO3)C |
Canonical SMILES |
CC1=NC2=C(N1)C(=O)N(C(=O)N2CC3=CC=CO3)C |
Synonyms |
3-(2-Furanylmethyl)-3,7-dihydro-1-methyl-8-(methyl-d3)-1H-purine-2,6-dione; 3-(2-furanylmethyl)-3,9-dihydro-1,8-dimethyl-1H-purine-2,6-dione-d3 |
Origin of Product |
United States |
Retrosynthesis Analysis
AI-Powered Synthesis Planning: Our tool employs the Template_relevance Pistachio, Template_relevance Bkms_metabolic, Template_relevance Pistachio_ringbreaker, Template_relevance Reaxys, Template_relevance Reaxys_biocatalysis model, leveraging a vast database of chemical reactions to predict feasible synthetic routes.
One-Step Synthesis Focus: Specifically designed for one-step synthesis, it provides concise and direct routes for your target compounds, streamlining the synthesis process.
Accurate Predictions: Utilizing the extensive PISTACHIO, BKMS_METABOLIC, PISTACHIO_RINGBREAKER, REAXYS, REAXYS_BIOCATALYSIS database, our tool offers high-accuracy predictions, reflecting the latest in chemical research and data.
Strategy Settings
Precursor scoring | Relevance Heuristic |
---|---|
Min. plausibility | 0.01 |
Model | Template_relevance |
Template Set | Pistachio/Bkms_metabolic/Pistachio_ringbreaker/Reaxys/Reaxys_biocatalysis |
Top-N result to add to graph | 6 |
Feasible Synthetic Routes
Disclaimer and Information on In-Vitro Research Products
Please be aware that all articles and product information presented on BenchChem are intended solely for informational purposes. The products available for purchase on BenchChem are specifically designed for in-vitro studies, which are conducted outside of living organisms. In-vitro studies, derived from the Latin term "in glass," involve experiments performed in controlled laboratory settings using cells or tissues. It is important to note that these products are not categorized as medicines or drugs, and they have not received approval from the FDA for the prevention, treatment, or cure of any medical condition, ailment, or disease. We must emphasize that any form of bodily introduction of these products into humans or animals is strictly prohibited by law. It is essential to adhere to these guidelines to ensure compliance with legal and ethical standards in research and experimentation.