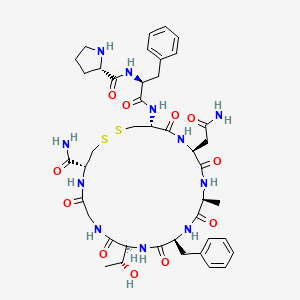
Crustacean Cardioactive Peptide
Overview
Description
Crustacean Cardioactive Peptide (CCAP) is a highly conserved cyclic nonapeptide (nine-amino-acid sequence: PFCNAFTGC-amide) with a disulfide bridge between two cysteine residues, forming a rigid bicyclic structure . It is encoded by a precursor protein of 141 amino acids (aa), which includes a 30-aa signal peptide and five distinct mature peptides . First isolated from the pericardial organs of the shore crab Carcinus maenas, CCAP regulates heartbeat in crustaceans and modulates neuronal activity across arthropods . Beyond cardiovascular functions, CCAP plays critical roles in molting (ecdysis) by coordinating motor programs and respiratory physiology . Its receptor is a G protein-coupled receptor (GPCR), activating intracellular pathways such as cAMP and calcium signaling .
Preparation Methods
Isolation from Natural Sources
The initial isolation of CCAP from crustacean tissues remains a foundational method for obtaining the native peptide. The pericardial organs (POs) of Carcinus maenas were identified as rich sources of CCAP due to their neurosecretory role in regulating cardiac activity .
Tissue Homogenization and Extraction
Pericardial organs were dissected from shore crabs and homogenized in an acidic methanol solution (80% methanol, 0.1% trifluoroacetic acid) to denature proteases and stabilize peptide bonds . The homogenate was centrifuged at 10,000 × g for 20 minutes, and the supernatant was lyophilized. Crude extracts were reconstituted in 0.1% heptafluorobutyric acid (HFBA) for subsequent purification .
Chromatographic Purification
A two-step reversed-phase high-performance liquid chromatography (RP-HPLC) protocol was employed:
-
First Dimension : A C₁₈ column (250 × 4.6 mm, 5 µm particle size) with a gradient of 10–60% acetonitrile in 0.1% HFBA over 40 minutes .
-
Second Dimension : A shallower gradient (20–40% acetonitrile) on the same column to resolve co-eluting contaminants .
Active fractions were identified using a semi-isolated crab heart bioassay, where CCAP induced dose-dependent increases in heart rate . The final yield from two pericardial organs averaged 30–40 pmol per animal, highlighting the labor-intensive nature of natural extraction .
Chemical Synthesis
Chemical synthesis has become the predominant method for producing CCAP, enabling scalable yields and structural modifications. Two approaches are widely used: solid-phase peptide synthesis (SPPS) and fragment condensation.
Solid-Phase Peptide Synthesis
The linear CCAP sequence was assembled using Fmoc (fluorenylmethyloxycarbonyl) chemistry on a Rink amide resin to ensure C-terminal amidation . Key steps included:
-
Resin Swelling : Dichloromethane (DCM) for 30 minutes.
-
Deprotection : 20% piperidine in dimethylformamide (DMF).
-
Coupling : Amino acids (4 equivalents) activated with HBTU (O-benzotriazole-N,N,N',N'-tetramethyluronium hexafluorophosphate) and DIEA (N,N-diisopropylethylamine) .
The disulfide bridge between Cys³ and Cys⁹ was formed post-synthesis via oxidative folding in 0.1 M ammonium bicarbonate (pH 8.5) with 5 mM oxidized glutathione . The cyclic structure was confirmed by mass spectrometry (MS) and co-elution with native CCAP on HPLC .
Table 1: Synthetic Parameters for CCAP
Parameter | Value | Source |
---|---|---|
Purity | ≥98% | |
Yield (Crude) | 60–70% | |
Oxidative Folding Time | 24–48 hours | |
Final Concentration | 5 × 10⁻⁶ M (in physiological saline) |
Recombinant Production
While recombinant methods for CCAP are less documented, advances in heterologous expression systems offer potential alternatives. A precursor mRNA encoding CCAP was identified in Scylla paramamosain, suggesting the possibility of bacterial or eukaryotic expression .
Cloning and Expression
The Sp-CCAP gene (GenBank accession: KT962314) was cloned into a pET-28a vector and expressed in Escherichia coli BL21(DE3) . Induction with 0.5 mM isopropyl β-D-1-thiogalactopyranoside (IPTG) at 16°C for 18 hours yielded inclusion bodies, which were solubilized in 8 M urea . Refolding and enzymatic processing (e.g., using furin-like proteases) remain challenges due to the small size and disulfide bond of CCAP .
Analytical Validation
Chromatographic Analysis
RP-HPLC with a C₁₈ column and linear acetonitrile gradient (10–60% in 0.1% HFBA) confirmed the purity of synthetic CCAP . Native and synthetic peptides exhibited identical retention times (14.2 minutes), validating structural fidelity .
Mass Spectrometry
Matrix-assisted laser desorption/ionization time-of-flight (MALDI-TOF) MS confirmed the molecular weight of CCAP as 956.1 Da (calculated: 956.0 Da) . Post-source decay analysis verified the disulfide bond by detecting fragments at m/z 348.1 (Cys³–Cys⁹) .
Biological Activity Assays
Synthetic CCAP was tested on a semi-isolated crab heart preparation, eliciting a 2.5-fold increase in contraction rate at 10⁻⁹ M . In Manduca sexta, CCAP (10⁻⁷ M) increased heart rate by 40% and enhanced myogenic rhythm in leg muscles .
Comparative Analysis of Preparation Methods
Table 2: Method Comparison
Method | Yield | Purity | Time Required | Cost |
---|---|---|---|---|
Natural Extraction | 30–40 pmol | 95–98% | Weeks | High |
Chemical Synthesis | 10–50 mg | 98–99% | 5–7 days | Moderate |
Recombinant | 1–5 mg/L | 70–80% | 10–14 days | Low |
Natural extraction is limited by low yields but preserves post-translational modifications. Chemical synthesis offers high purity and scalability, while recombinant methods are cost-effective but require optimization .
Scientific Research Applications
Crustacean Cardioactive Peptide has a wide range of applications in scientific research:
Chemistry: Used as a model peptide for studying peptide synthesis and cyclization reactions.
Medicine: Potential therapeutic applications in regulating heart rate and treating cardiac disorders.
Industry: Used in aquaculture to study the physiological responses of crustaceans to environmental stressors.
Mechanism of Action
Crustacean Cardioactive Peptide exerts its effects by binding to specific G protein-coupled receptors (GPCRs) on target cells . This binding activates intracellular signaling pathways, leading to various physiological responses. For example, in the heart, it increases the heart rate by enhancing the influx of calcium ions into cardiac muscle cells . In the gut, it modulates the contraction of smooth muscles, facilitating digestion .
Comparison with Similar Compounds
Comparative Analysis with Similar Peptides
Ecdysis-Triggering Hormone (ETH) and Eclosion Hormone (EH)
Structural and Functional Overlap
- ETH : A 26-aa peptide that initiates ecdysis behavior in insects and crustaceans. ETH acts upstream of CCAP in the molting cascade, triggering the release of CCAP from neurons .
- EH : A 62-aa peptide that synergizes with ETH to activate CCAP-expressing neurons, facilitating cuticle shedding .
Key Differences
- Hierarchy: ETH is necessary for initiating ecdysis, while CCAP executes the motor phase.
- Expression : ETH is localized in epitracheal glands, while CCAP is expressed in central nervous system neurons .
FMRFamide-Related Peptides (FaRPs)
Functional Similarities
FaRPs, including F1 and F2, share a C-terminal RFamide motif and regulate cardiovascular activity in crustaceans. Like CCAP, they are stored in pericardial organs and modulate heart rate .
Divergent Effects
- In Vitro vs. In Vivo : In semi-isolated crab hearts, F1/F2 and CCAP are cardioexcitatory. However, in vivo, F1/F2 inhibit heart rate and stroke volume in Cancer magister, while CCAP exhibits minimal effects in this species .
- Specificity : CCAP is more potent in stimulating gut contractions and α-amylase activity in insects (e.g., Locusta migratoria), whereas FaRPs primarily target cardiovascular tissues .
Crustacean Female Sex Hormone (CFSH)
Functional Contrast
CFSH, a vitellogenesis-stimulating hormone, is structurally distinct from CCAP. While CCAP is a neuropeptide, CFSH is a large glycoprotein (>100 kDa) produced in the eyestalk and regulates ovarian maturation .
Gonadotropin-Releasing Hormone (GnRH)
Mechanistic Parallels Both CCAP and GnRH act via GPCRs and modulate physiological processes through cAMP pathways. However, GnRH regulates reproduction in vertebrates, whereas CCAP targets cardiovascular and nervous systems in invertebrates .
Biological Activity
Crustacean cardioactive peptide (CCAP) is a neuropeptide predominantly found in arthropods, notably crustaceans. It plays a crucial role in regulating various physiological functions, particularly cardiac activity. This article delves into the biological activity of CCAP, highlighting its effects on heart function, potential applications in pharmacology, and recent research findings.
Chemical Composition and Characteristics
CCAP is characterized by its cyclic structure and amidation. Its sequence is H-Pro-Phe-Cys-Asn-Ala-Phe-Thr-Gly-Cys-NH2, with a molecular weight of approximately 956.11 g/mol and a molecular formula of C42H56N10O12S2 . The peptide is synthesized in the pericardial organs of crustaceans and is released into the hemolymph, where it exerts its physiological effects.
Biological Functions
The primary biological activities of CCAP include:
- Cardiac Regulation : CCAP is well-known for its ino- (force of contraction) and chrono- (heart rate) tropic effects. It enhances cardiac output by increasing the frequency and strength of heart contractions in semi-isolated crab heart preparations .
- Regulation of Other Physiological Processes : Beyond cardiac function, CCAP influences:
Research Findings and Case Studies
Recent studies have expanded our understanding of CCAP's functions:
- Expression Patterns : A study on Scylla paramamosain identified a CCAP precursor that could be processed into multiple peptides, indicating its complex regulatory roles across different tissues. The expression of the CCAP receptor was found in various tissues, including the hepatopancreas, suggesting a broader physiological impact .
- Immunoregulatory Roles : Novel findings suggest that CCAP may be involved in immune responses. The mRNA expression of the CCAP receptor significantly increased following immune challenges, indicating a potential role in modulating immune functions .
- Comparative Studies : Research comparing various arthropods has shown that CCAP is highly conserved across species, reinforcing its fundamental role in arthropod physiology. For instance, studies have documented its presence and effects in crabs, crayfish, and insects .
Potential Applications in Pharmacology
Given its potent biological activities, CCAP is being explored for therapeutic applications:
- Cardiovascular Research : The mechanisms by which CCAP influences cardiac function could inform the development of new treatments for heart diseases. Researchers are investigating analogs that might mimic or modulate CCAP's effects to create novel cardiovascular therapies .
- Aquaculture : Understanding CCAP's role in molting and immune responses can lead to improved management strategies for aquaculture species, enhancing growth rates and disease resistance .
Data Summary Table
Q & A
Basic Research Questions
Q. What are the primary physiological roles of CCAP in arthropods, and what experimental approaches validate these functions?
CCAP is a conserved cyclic nonapeptide regulating heartbeat in crustaceans (e.g., Carcinus maenas) and modulating neuronal activity in insects. Key methodologies include:
- Immunohistochemistry to localize CCAP in pericardial organs or neuronal tissues .
- RNA interference (RNAi) to silence CCAP expression and observe phenotypic changes in cardiac or locomotor activity .
- In vivo bioassays measuring heart rate or gut contraction in response to CCAP administration .
Q. Which model organisms are optimal for studying CCAP signaling pathways?
- Crustaceans (Carcinus maenas, crabs): Ideal for cardiac regulation studies due to CCAP's origin in pericardial organs .
- Insects (Locusta migratoria, locusts): Suitable for neuronal modulation research, leveraging genetic tools (e.g., CRISPR/Cas9) to probe receptor interactions .
- Cross-species comparisons (e.g., crabs vs. insects) to assess evolutionary conservation of CCAP functions .
Q. What techniques are used to analyze CCAP's structural and functional conservation?
- Sequence alignment : Compare CCAP variants across species using databases like GenBank (e.g., Accession MN923209) .
- Mass spectrometry (MS) and nuclear magnetic resonance (NMR) to confirm cyclic structure and post-translational modifications (e.g., amidation) .
Advanced Research Questions
Q. How can researchers resolve contradictory data on CCAP's effects across species (e.g., cardiac vs. neuronal modulation)?
- Comparative receptor profiling : Identify CCAP receptor isoforms in divergent species using radioligand binding assays or cryo-EM .
- Context-dependent assays : Test CCAP under varying physiological conditions (e.g., stress, molting) to explain species-specific responses .
- Systems biology approaches : Integrate transcriptomic and proteomic data to map CCAP signaling networks in different tissues .
Q. What methodologies ensure batch-to-batch consistency in synthetic CCAP for sensitive bioassays?
- HPLC purity analysis (>99.5%) and MS verification to confirm peptide integrity .
- Peptide content quantification (via amino acid analysis) to standardize concentrations across batches .
- Solubility testing in buffers (e.g., PBS with 0.1% BSA) to minimize variability in biological assays .
Q. How to design experiments investigating CCAP's immunomodulatory roles in hepatopancreas?
- RNAi knockdown : Suppress CCAP expression in crabs and measure immune markers (e.g., antimicrobial peptides) via qPCR .
- Co-stimulation assays : Combine CCAP with pathogens (e.g., Vibrio spp.) to assess immune response amplification .
- Ethical controls : Include sham-operated animals and sample size calculations approved by institutional ethics committees (e.g., Xiamen University protocols) .
Q. What interdisciplinary frameworks (e.g., PICO/FINER) apply to CCAP hypothesis development?
- PICO framework : Define Population (e.g., Carcinus maenas), Intervention (CCAP administration), Comparison (wild-type vs. CCAP-deficient models), and Outcome (heart rate variability) .
- FINER criteria : Ensure hypotheses are Feasible (e.g., using CRISPR-edited models), Novel (addressing CCAP-immune crosstalk gaps), and Ethical (adhering to animal welfare guidelines) .
Q. How do CCAP salt forms (e.g., acetate) influence experimental outcomes?
- Bioactivity assays : Compare CCAP acetate vs. free peptide in receptor-binding studies (e.g., AVPR1B affinity tests) .
- Solubility optimization : Test acetate formulations in physiological buffers to enhance stability for long-term experiments .
Q. What strategies validate CCAP's role in modulating adipokinetic hormone (AKH) release?
- In vitro neurosecretory assays : Incubate insect corpora cardiaca with CCAP and measure AKH secretion via ELISA .
- Sugar inhibition studies : Administer glucose/fructose to test CCAP-AKH crosstalk in energy homeostasis .
Q. How to integrate CCAP research with neuroendocrinology and ecotoxicology?
Properties
IUPAC Name |
(4R,10S,13S,16S,19S,22R)-19-(2-amino-2-oxoethyl)-13-benzyl-10-[(1R)-1-hydroxyethyl]-16-methyl-6,9,12,15,18,21-hexaoxo-22-[[(2S)-3-phenyl-2-[[(2S)-pyrrolidine-2-carbonyl]amino]propanoyl]amino]-1,2-dithia-5,8,11,14,17,20-hexazacyclotricosane-4-carboxamide | |
---|---|---|
Source | PubChem | |
URL | https://pubchem.ncbi.nlm.nih.gov | |
Description | Data deposited in or computed by PubChem | |
InChI |
InChI=1S/C42H57N11O11S2/c1-22-36(58)49-28(17-25-12-7-4-8-13-25)40(62)53-34(23(2)54)42(64)46-19-33(56)48-30(35(44)57)20-65-66-21-31(41(63)51-29(18-32(43)55)38(60)47-22)52-39(61)27(16-24-10-5-3-6-11-24)50-37(59)26-14-9-15-45-26/h3-8,10-13,22-23,26-31,34,45,54H,9,14-21H2,1-2H3,(H2,43,55)(H2,44,57)(H,46,64)(H,47,60)(H,48,56)(H,49,58)(H,50,59)(H,51,63)(H,52,61)(H,53,62)/t22-,23+,26-,27-,28-,29-,30-,31-,34-/m0/s1 | |
Source | PubChem | |
URL | https://pubchem.ncbi.nlm.nih.gov | |
Description | Data deposited in or computed by PubChem | |
InChI Key |
KUAKWTJOHSDJOA-HNGYRCOKSA-N | |
Source | PubChem | |
URL | https://pubchem.ncbi.nlm.nih.gov | |
Description | Data deposited in or computed by PubChem | |
Canonical SMILES |
CC1C(=O)NC(C(=O)NC(C(=O)NCC(=O)NC(CSSCC(C(=O)NC(C(=O)N1)CC(=O)N)NC(=O)C(CC2=CC=CC=C2)NC(=O)C3CCCN3)C(=O)N)C(C)O)CC4=CC=CC=C4 | |
Source | PubChem | |
URL | https://pubchem.ncbi.nlm.nih.gov | |
Description | Data deposited in or computed by PubChem | |
Isomeric SMILES |
C[C@H]1C(=O)N[C@H](C(=O)N[C@H](C(=O)NCC(=O)N[C@@H](CSSC[C@@H](C(=O)N[C@H](C(=O)N1)CC(=O)N)NC(=O)[C@H](CC2=CC=CC=C2)NC(=O)[C@@H]3CCCN3)C(=O)N)[C@@H](C)O)CC4=CC=CC=C4 | |
Source | PubChem | |
URL | https://pubchem.ncbi.nlm.nih.gov | |
Description | Data deposited in or computed by PubChem | |
Molecular Formula |
C42H57N11O11S2 | |
Source | PubChem | |
URL | https://pubchem.ncbi.nlm.nih.gov | |
Description | Data deposited in or computed by PubChem | |
Molecular Weight |
956.1 g/mol | |
Source | PubChem | |
URL | https://pubchem.ncbi.nlm.nih.gov | |
Description | Data deposited in or computed by PubChem | |
Disclaimer and Information on In-Vitro Research Products
Please be aware that all articles and product information presented on BenchChem are intended solely for informational purposes. The products available for purchase on BenchChem are specifically designed for in-vitro studies, which are conducted outside of living organisms. In-vitro studies, derived from the Latin term "in glass," involve experiments performed in controlled laboratory settings using cells or tissues. It is important to note that these products are not categorized as medicines or drugs, and they have not received approval from the FDA for the prevention, treatment, or cure of any medical condition, ailment, or disease. We must emphasize that any form of bodily introduction of these products into humans or animals is strictly prohibited by law. It is essential to adhere to these guidelines to ensure compliance with legal and ethical standards in research and experimentation.