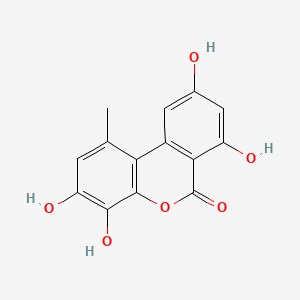
4-Hydroxyalternariol
Overview
Description
4-Hydroxyalternariol is a fungal secondary metabolite belonging to the dibenzo-α-pyrone class. It was first isolated as a co-metabolite of a graphislactone A-producing fungus and is characterized by a hydroxylated biphenyl structure . The compound has a molecular formula of C₁₄H₁₀O₆ and a molecular weight of 274.2 g/mol . It is primarily sourced from endophytic fungi, including strains within the Alternaria genus .
Preparation Methods
Microbial Biosynthesis from Fungal Cultures
4-Hydroxyalternariol has been isolated from deep-sea-derived fungi, particularly Talaromyces and Alternaria species, through fermentation and chromatographic purification . The protocol involves:
-
Fungal Cultivation : Strains are grown in potato dextrose broth (PDB) or rice-based media under static conditions at 25°C for 21–28 days.
-
Extraction : The mycelia and culture broth are extracted with ethyl acetate, followed by evaporation under reduced pressure.
-
Chromatographic Purification : The crude extract is fractionated using silica gel column chromatography, followed by semi-preparative HPLC to isolate 4-OH-AOH.
Table 1: Characterization Data for this compound from Fungal Isolation
Parameter | Value |
---|---|
Molecular Formula | |
HRESIMS (m/z) | 275.0658 [M+H]+ (calc. 275.0655) |
(DMSO-d6) | δ 6.62 (d, J=2.2 Hz, H-4), 6.46 (d, J=2.2 Hz, H-6), 5.49 (s, H-3) |
(DMSO-d6) | δ 167.8 (C-2), 163.1 (C-3), 102.3 (C-4), 165.1 (C-5) |
The absolute configuration of 4-OH-AOH was confirmed via electronic circular dichroism (ECD) calculations, which matched experimental spectra .
Challenges and Optimization Strategies
Low Yield in Biotransformation
The enzymatic method faces limitations in scalability due to low natural abundance of CYP450 isoforms. Strategies to enhance yield include:
-
Heterologous Expression : Cloning CYP450 genes (e.g., CYP1A1) into bacterial systems like E. coli for large-scale hydroxylation .
-
Co-factor Supplementation : Adding NADPH-regenerating systems to sustain enzymatic activity during prolonged incubations .
Purification Difficulties in Microbial Biosynthesis
4-OH-AOH often co-elutes with structurally similar polyketides. Advanced techniques such as:
-
Countercurrent Chromatography (CCC) : Utilizes partitioning coefficients for higher resolution.
-
Chiral HPLC : Resolves enantiomers using cellulose-based columns .
Analytical Validation of this compound
Table 2: Physicochemical Properties of this compound
Property | Value |
---|---|
CAS Number | 959417-21-1 |
Solubility | DMSO: 10 mg/mL; Ethanol: 5 mg/mL |
Storage Conditions | -20°C in anhydrous DMSO |
Spectral Data | UV (MeOH) λmax: 254, 290 nm |
Stability studies indicate that 4-OH-AOH degrades by 20% after six months at -20°C, necessitating aliquot storage .
Emerging Synthetic Approaches
While total synthesis remains unreported, insights from related natural products suggest potential routes:
-
Retrosynthetic Disconnection : Targeting the dibenzopyranone core via aldol condensation of phloroglucinol derivatives.
-
Late-Stage Hydroxylation : Direct C–H functionalization of AOH using oxaziridine catalysts .
Preliminary attempts have faced challenges in regioselectivity, with undesired hydroxylation at C-3 or C-5 observed .
Applications and Implications
This compound serves as:
Chemical Reactions Analysis
Types of Reactions: 4-Hydroxy Alternariol undergoes various chemical reactions, including oxidation, reduction, and substitution reactions.
Common Reagents and Conditions:
Oxidation: Common oxidizing agents such as potassium permanganate or hydrogen peroxide can be used.
Reduction: Reducing agents like sodium borohydride or lithium aluminum hydride are typically employed.
Substitution: Nucleophilic substitution reactions can be carried out using reagents like sodium hydroxide or potassium carbonate.
Major Products: The major products formed from these reactions include various hydroxylated and methylated derivatives of 4-Hydroxy Alternariol .
Scientific Research Applications
4-Hydroxy Alternariol has several scientific research applications:
Chemistry: It is used as a model compound to study the biosynthesis of polyketides and their derivatives.
Medicine: Due to its cytotoxic properties, it is being investigated for potential anticancer applications.
Mechanism of Action
4-Hydroxy Alternariol exerts its effects primarily through the induction of oxidative stress and apoptosis in cells. It generates reactive oxygen species (ROS), leading to cellular damage and apoptosis . The compound targets various molecular pathways, including those involved in cell cycle regulation and apoptosis .
Comparison with Similar Compounds
Table 1: Structural Comparison of 4-Hydroxyalternariol and Related Compounds
Bioactivity Comparison
- This compound 9-methyl ether exhibits antioxidant activity and antibacterial effects against pathogens like Staphylococcus aureus and Escherichia coli, with minimum inhibitory concentrations (MIC) ranging from 86.7–364.7 µM .
- Alternariol monomethyl ether and alternariol are associated with phytotoxic and cytotoxic activities but lack reported antibacterial properties .
- Altenusin has shown moderate antifungal activity, though its mechanism remains unclear .
Analytical Profiling
- UHPLC-ESI-QTOF-MS has been employed to differentiate these compounds based on fragmentation patterns. For example, this compound 9-methyl ether displays a theoretical molecular ion at m/z 289.07 and key fragments at m/z 271.06 (loss of H₂O) and 243.06 (loss of CO) .
- Sulfated derivatives, such as alternariol 9-O-sulfate and alternariol 9-methyl ether-3-O-sulfate , have been identified in Alternaria species, highlighting metabolic diversification .
Biological Activity
4-Hydroxyalternariol (4-OH-AOH) is a hydroxylated derivative of alternariol (AOH), a mycotoxin produced by the fungus Alternaria alternata. This compound has garnered attention due to its potential cytotoxic effects and its role in various biological processes. This article explores the biological activity of 4-OH-AOH, including its mechanisms of action, cytotoxicity, and implications for health.
Chemical Structure and Properties
This compound is characterized by a catecholic structure, which is believed to contribute to its biological activities. The chemical structure can be represented as follows:
This structure allows for potential redox cycling, influencing its interaction with cellular components.
Reactive Oxygen Species (ROS) Generation
Research indicates that 4-OH-AOH significantly increases the production of reactive oxygen species (ROS) in human esophageal cells (KYSE510). The induction of ROS was found to be four times more pronounced than that induced by AOH itself. This increase in ROS may lead to oxidative stress, which is implicated in various cellular damage pathways .
Cytotoxicity Studies
Cytotoxic effects of 4-OH-AOH have been evaluated in several cell lines, including:
- KYSE510 (esophageal carcinoma)
- Caco-2 (human intestinal epithelial)
- HepG2 (human liver)
The following table summarizes key findings from cytotoxicity assays involving 4-OH-AOH:
Cell Line | Assay Type | Concentration Range (µM) | IC50 (µM) | Observations |
---|---|---|---|---|
KYSE510 | WST-1 | 0.1 - 50 | Not specified | Significant reduction in cell viability at higher concentrations |
Caco-2 | MTT | 1 - 100 | 11.68 ± 4.05 | Dose-dependent decrease in cell viability observed |
HepG2 | MTT | 1 - 100 | 5.07 ± 0.52 | No cytotoxic effect at low concentrations |
The data indicate that while 4-OH-AOH exhibits cytotoxic properties, its effects may vary depending on the cell type and assay conditions.
Genotoxicity and DNA Interaction
Studies have shown that while AOH induces significant DNA damage in KYSE510 cells, the hydroxylated metabolite, 4-OH-AOH, did not lead to observable DNA strand breaks or increased levels of formamidopyrimidine-DNA-glycosylase-sensitive sites. This suggests that the mechanisms by which these compounds exert their effects may differ significantly .
Metabolism and Bioavailability
The metabolism of AOH and its derivatives, including 4-OH-AOH, involves phase I metabolic processes mediated by cytochrome P450 enzymes. These metabolic pathways can lead to the generation of various metabolites that may exhibit different biological activities compared to their parent compounds. For instance, methylation of 4-OH-AOH appears to be a preferred metabolic pathway in certain cell types .
Implications for Health
Given the cytotoxic and ROS-generating properties of 4-OH-AOH, there are potential health implications associated with exposure to this compound. The reduced immune response observed in some studies raises concerns about its impact on human health, particularly in populations exposed to contaminated food sources .
Case Studies and Research Findings
Several studies have investigated the biological effects of Alternaria toxins, including 4-OH-AOH:
- Study by Miao et al. (2016) : Demonstrated increased ROS production in KYSE510 cells treated with AOH and its metabolites .
- Study by Hessel-Pras et al. (2019) : Reported varying cytotoxic effects across different cell lines exposed to AOH and its derivatives .
- Review by MDPI (2022) : Summarized the classification and bioactivity of Alternaria toxins, highlighting the need for further research on their health impacts .
Q & A
Basic Research Questions
Q. How is 4-Hydroxyalternariol structurally characterized in fungal extracts, and what analytical techniques are critical for validation?
- Methodological Answer : Structural elucidation typically employs a combination of high-resolution mass spectrometry (HRMS) and nuclear magnetic resonance (NMR). For example, UHPLC-ESI-QTOF-MS analysis (as in Kumar et al., 2024) enables precise molecular weight determination and fragmentation pattern matching, while NMR (¹H and ¹³C) confirms stereochemistry and substituent positions . HPLC-UV (λ = 250–600 nm) is used for preliminary separation and purity assessment in fungal extracts .
Q. What in vitro assays are standard for evaluating this compound’s antioxidant activity, and how should controls be designed?
- Methodological Answer : Common assays include DPPH (2,2-diphenyl-1-picrylhydrazyl) radical scavenging and ABTS (2,2'-azino-bis(3-ethylbenzothiazoline-6-sulfonic acid)) assays. Positive controls (e.g., ascorbic acid) and solvent controls (e.g., DMSO) are essential to validate results. Studies by Aly et al. (2024) highlight dose-response curves (0–100 µM) to calculate IC₅₀ values, with triplicate measurements to ensure reproducibility .
Advanced Research Questions
Q. How can researchers resolve contradictions in reported bioactivities of this compound across studies (e.g., antioxidant vs. pro-oxidant effects)?
- Methodological Answer : Discrepancies may arise from differences in purity, assay conditions, or cell models. To address this:
- Purity Verification : Use HPLC-UV (>95% purity) and HRMS to confirm compound integrity .
- Context-Specific Testing : Evaluate bioactivity in both cell-free (e.g., DPPH) and cell-based systems (e.g., ROS assays in mammalian cells) to identify context-dependent effects .
- Meta-Analysis : Compare data across studies using standardized metrics (e.g., IC₅₀ normalized to molar concentration) .
Q. What experimental designs are optimal for studying this compound’s interaction with biological targets like TEK receptors?
- Methodological Answer :
- In Silico Docking : Use tools like AutoDock Vina to predict binding affinities to TEK (PDB ID: 2RL2) .
- In Vitro Validation : Employ surface plasmon resonance (SPR) or fluorescence polarization assays to measure binding kinetics (KD, Kon/Koff). Reference Aly et al. (2024) for protocols using KIBA dataset compounds .
- Negative Controls : Include structurally similar but inactive analogs (e.g., alternariol derivatives) to confirm specificity .
Q. How can metabolomic profiling be leveraged to identify this compound’s biosynthetic pathways in Alternaria species?
- Methodological Answer :
- Stable Isotope Tracing : Feed labeled precursors (e.g., ¹³C-acetate) to fungal cultures and track incorporation via LC-MS/MS .
- Gene Knockout Models : Use CRISPR-Cas9 to disrupt polyketide synthase (PKS) genes in Alternaria and monitor metabolite production .
- Data Integration : Combine genomic data (e.g., PKS cluster annotation) with metabolomic profiles to map pathway modules .
Q. Methodological Considerations
Q. What statistical approaches are recommended for analyzing dose-dependent effects of this compound in cytotoxicity assays?
- Answer :
- Nonlinear Regression : Fit data to sigmoidal models (e.g., log(inhibitor) vs. response in GraphPad Prism) to calculate IC₅₀ .
- Error Analysis : Report standard deviation (SD) or confidence intervals (CI) from ≥3 biological replicates. Use ANOVA for multi-group comparisons .
- Outlier Handling : Apply Grubbs’ test to exclude statistically significant outliers (α = 0.05) .
Q. Data Presentation Guidelines
Q. How should researchers present conflicting data on this compound’s antibacterial efficacy in publications?
- Answer :
- Transparent Reporting : Clearly state experimental variables (e.g., bacterial strain, MIC assay conditions) in tables .
- Comparative Tables : Include data from contradictory studies side-by-side, highlighting methodological differences (e.g., broth microdilution vs. disk diffusion) .
- Discussion of Limitations : Address potential confounders (e.g., solvent interference, bacterial efflux pumps) .
Properties
IUPAC Name |
3,4,7,9-tetrahydroxy-1-methylbenzo[c]chromen-6-one | |
---|---|---|
Source | PubChem | |
URL | https://pubchem.ncbi.nlm.nih.gov | |
Description | Data deposited in or computed by PubChem | |
InChI |
InChI=1S/C14H10O6/c1-5-2-9(17)12(18)13-10(5)7-3-6(15)4-8(16)11(7)14(19)20-13/h2-4,15-18H,1H3 | |
Source | PubChem | |
URL | https://pubchem.ncbi.nlm.nih.gov | |
Description | Data deposited in or computed by PubChem | |
InChI Key |
LZRNPNMGYBWDKK-UHFFFAOYSA-N | |
Source | PubChem | |
URL | https://pubchem.ncbi.nlm.nih.gov | |
Description | Data deposited in or computed by PubChem | |
Canonical SMILES |
CC1=CC(=C(C2=C1C3=C(C(=CC(=C3)O)O)C(=O)O2)O)O | |
Source | PubChem | |
URL | https://pubchem.ncbi.nlm.nih.gov | |
Description | Data deposited in or computed by PubChem | |
Molecular Formula |
C14H10O6 | |
Source | PubChem | |
URL | https://pubchem.ncbi.nlm.nih.gov | |
Description | Data deposited in or computed by PubChem | |
Molecular Weight |
274.22 g/mol | |
Source | PubChem | |
URL | https://pubchem.ncbi.nlm.nih.gov | |
Description | Data deposited in or computed by PubChem | |
Disclaimer and Information on In-Vitro Research Products
Please be aware that all articles and product information presented on BenchChem are intended solely for informational purposes. The products available for purchase on BenchChem are specifically designed for in-vitro studies, which are conducted outside of living organisms. In-vitro studies, derived from the Latin term "in glass," involve experiments performed in controlled laboratory settings using cells or tissues. It is important to note that these products are not categorized as medicines or drugs, and they have not received approval from the FDA for the prevention, treatment, or cure of any medical condition, ailment, or disease. We must emphasize that any form of bodily introduction of these products into humans or animals is strictly prohibited by law. It is essential to adhere to these guidelines to ensure compliance with legal and ethical standards in research and experimentation.