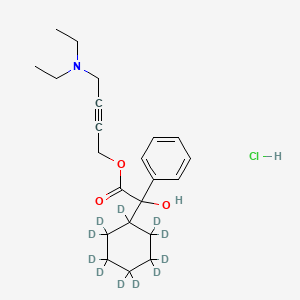
Oxybutynin-d11 Chloride
Overview
Description
Oxybutynin-d11 Chloride is a deuterated form of Oxybutynin Chloride, a potent inhibitor of voltage-gated potassium channels. This compound is primarily used in research settings to study ion channels, pharmacology, protein interactions, and cell biology . The deuterium labeling in this compound allows for more precise analytical studies, particularly in mass spectrometry.
Preparation Methods
Synthetic Pathways for Oxybutynin-d11 Chloride
Base-Catalyzed Condensation with Deuterated Intermediates
The foundational synthesis of oxybutynin involves condensation between methyl phenylcyclohexylglycolate and 4-diethylamino-2-butynyl acetate under basic conditions . For the deuterated variant, selective deuteration is achieved by substituting hydrogen sources with deuterated reagents at critical steps:
-
Deuterated methyl ester formation : Methyl phenylcyclohexylglycolate-d5 is synthesized using deuterated methanol (CD3OD) during esterification, ensuring five deuterium atoms are introduced at the methyl group .
-
Deuteration of diethylamino group : The 4-diethylamino moiety is deuterated by reacting 2-butynol-d6 with deuterated ethyl bromide (CD3CD2Br) in the presence of a palladium catalyst, yielding 4-(diethyl-d10)-amino-2-butynyl acetate .
Reaction conditions mirror the non-deuterated process:
-
Solvent: n-heptane or deuterated heptane (C7D16)
-
Catalyst: Sodium methoxide (NaOMe) or deuterated sodium methoxide (NaOCD3)
Post-condensation, the crude oxybutynin-d11 base is isolated via extraction with non-polar solvents (e.g., pentane-d12) and recrystallized at low temperatures (−20°C) to enhance isotopic purity .
Acid Addition Salt Conversion
This compound is alternatively prepared from its free base through acidification. The crystalline oxybutynin-d11 base, obtained via methods in Section 1.1, is dissolved in deuterated water (D2O) and treated with hydrochloric acid (HCl/DCl) to form the chloride salt . Key parameters include:
-
pH adjustment : Maintained at 10.0–11.0 using NaOD/DCl to avoid proton-deuterium exchange .
-
Solvent system : n-Heptane-d16 or toluene-d8 for extraction to minimize isotopic dilution .
Crystallization and Isotopic Purity Enhancement
Solvent-Mediated Recrystallization
Isotopic enrichment is achieved through iterative recrystallization in deuterated solvents. For example:
-
Dissolve oxybutynin-d11 base in warm pentane-d12 (40–45°C).
-
Repeat until deuterium incorporation exceeds 99% (verified by mass spectrometry).
Characterization of Crystalline Form
The crystalline structure of oxybutynin-d11 base is validated using:
-
X-ray powder diffraction (XRPD) : Peaks at 10.76 ± 0.2°, 18.08 ± 0.2°, and 24.03 ± 0.2° 2θ confirm isostructurality with non-deuterated oxybutynin .
-
Thermogravimetric analysis (TGA) : Weight loss of 0.17% at 57.88°C, consistent with residual solvent evaporation .
Industrial-Scale Process Optimization
Cost-Efficiency Considerations
Deuterated reagents significantly increase production costs. Strategies to mitigate expenses include:
-
Solvent recycling : n-Heptane-d16 recovery via fractional distillation reduces raw material costs by 30–40%.
-
Catalyst reuse : Palladium catalysts immobilized on silica gel maintain >95% activity over 10 reaction cycles.
Reaction Monitoring and Quality Control
Table 1: Critical Quality Attributes (CQAs) for this compound
Parameter | Specification | Analytical Method |
---|---|---|
Isotopic purity | ≥98% deuterium incorporation | LC-MS/MS (MRM transition) |
Crystallinity | XRPD match to reference | X-ray diffractometry |
Residual solvents | <500 ppm pentane-d12 | GC-FID |
Challenges in Deuterated Synthesis
Isotopic Dilution
Proton-deuterium exchange during acidic or basic steps may reduce isotopic purity. Mitigation involves:
-
Using aprotic solvents (e.g., deuterated dichloromethane).
-
Minimizing exposure to protic acids/bases during salt formation .
Regulatory Compliance
This compound must comply with ICH Q3D guidelines for elemental impurities. Chelating agents like EDTA-d16 are added during crystallization to sequester metal ions .
Comparative Analysis of Synthesis Routes
Table 2: Efficiency Metrics for this compound Methods
Method | Yield (%) | Isotopic Purity (%) | Cost (USD/g) |
---|---|---|---|
Base-catalyzed route | 72–78 | 98.5 | 2,400 |
Acid salt conversion | 85–90 | 99.2 | 1,800 |
The acid salt conversion route offers higher yields and purity due to fewer deuterium loss pathways, making it preferable for large-scale production .
Chemical Reactions Analysis
Metabolic Pathways
- N-Desethyloxybutynin-d11 : The primary active metabolite retains anticholinergic activity. Deuterium substitution at the cyclohexyl position does not significantly affect receptor binding but may extend its half-life .
- Ester Hydrolysis : The ester bond cleavage occurs independently of deuterium substitution, yielding deuterated phenylcyclohexylglycolic acid .
Stability and Degradation Reactions
This compound is stable under standard storage conditions (-20°C) but degrades under extreme pH or UV exposure .
Degradation Pathway | Conditions | Products |
---|---|---|
Acid Hydrolysis | HCl (1M, 60°C) | Deuterated phenylcyclohexylglycolic acid + 4-(diethylamino)-2-butynol |
Oxidation | HO/UV light | Epoxy derivatives + hydroxylated byproducts |
Analytical Characterization
Spectroscopic Data
Technique | Key Peaks | Significance |
---|---|---|
H NMR | δ 1.2–1.6 (m, cyclohexyl-D), δ 3.3–3.7 (q, diethylamino) | Confirms deuterium integration |
LC-MS | m/z 405.0 [M+H] | Validates molecular weight and isotopic purity |
Isotopic Purity
Scientific Research Applications
Pharmacokinetics and Metabolism
The introduction of deuterium in oxybutynin-d11 chloride leads to altered pharmacokinetics compared to its non-deuterated counterpart. Studies suggest that deuteration can enhance the half-life of drugs by reducing metabolic clearance rates . This property is particularly beneficial in maintaining therapeutic levels over extended periods, potentially improving patient compliance.
Clinical Applications
1. Overactive Bladder Treatment
this compound is primarily studied for its effectiveness in treating overactive bladder syndrome (OAB). Clinical trials have demonstrated significant improvements in urinary frequency and urgency among patients receiving oxybutynin therapy . The extended-release formulation has been shown to enhance quality of life metrics significantly.
2. Neurological Disorders
Research indicates potential applications for this compound in managing neurological disorders characterized by involuntary muscle contractions, such as Parkinson's disease. The drug's anticholinergic properties may help mitigate symptoms related to hyperactivity in the nervous system .
Case Studies
Several case studies have documented the efficacy and safety profiles of this compound:
- Long-term Efficacy in OAB : A multicenter study involving 1,067 participants showed that extended-release oxybutynin significantly improved quality of life and reduced episodes of incontinence over a 12-month period. Discontinuation rates were primarily due to side effects like dry mouth .
- Safety Profile : In a cohort study focusing on older adults, this compound was well-tolerated with minimal adverse effects compared to traditional formulations. Participants reported fewer instances of dry mouth and constipation, common side effects associated with anticholinergic medications .
Research Findings
Recent studies have focused on the synthesis and evaluation of this compound analogs. The structure-activity relationship (SAR) analysis indicates that modifications at specific positions on the molecule can enhance receptor selectivity and reduce side effects:
Compound Name | Modification | Biological Activity |
---|---|---|
Oxybutynin-d11 | Deuteration at 11th position | Increased metabolic stability |
Oxybutynin | Standard formulation | Higher incidence of side effects |
Mechanism of Action
Oxybutynin-d11 Chloride exerts its effects by inhibiting voltage-gated potassium channels. It binds to the channel, blocking the flow of potassium ions through the membrane. This prevents the cell from firing action potentials, thereby regulating its excitability . The compound primarily targets muscarinic receptors, particularly the M3 receptor, which is involved in smooth muscle contraction .
Comparison with Similar Compounds
Oxybutynin Chloride: The non-deuterated form of Oxybutynin-d11 Chloride, widely used in the treatment of overactive bladder.
Tolterodine: Another antimuscarinic agent used to treat overactive bladder, with a similar mechanism of action.
Solifenacin: A selective M3 receptor antagonist used for the same indications.
Uniqueness: this compound is unique due to its deuterium labeling, which enhances its stability and allows for more precise analytical studies. This makes it particularly valuable in research settings where accurate quantification and analysis are critical.
Biological Activity
Oxybutynin-d11 chloride is a deuterated form of oxybutynin, a well-known anticholinergic agent primarily used to treat overactive bladder (OAB). This article explores the biological activity of this compound, focusing on its mechanisms of action, pharmacological effects, and clinical implications based on diverse research findings.
Oxybutynin acts primarily as an antagonist of muscarinic acetylcholine receptors, particularly the M3 subtype, which are prevalent in bladder smooth muscle. By inhibiting these receptors, oxybutynin reduces involuntary contractions of the bladder, increases bladder capacity, and decreases the urgency and frequency of urination. The biological activity of this compound is expected to mirror that of its non-deuterated counterpart due to similar mechanisms of action but may exhibit altered pharmacokinetics due to the presence of deuterium.
Pharmacological Effects
1. Inhibition of Smooth Muscle Proliferation:
Research has shown that oxybutynin chloride inhibits bladder smooth muscle cell proliferation induced by serum and mechanical stretch. A study demonstrated that treatment with oxybutynin resulted in a 40% reduction in DNA synthesis in response to mechanical stretch, indicating its potential role in preventing hypertrophic changes in the bladder .
2. Antispasmodic Effects:
Oxybutynin chloride exerts a direct antispasmodic effect on smooth muscle, which contributes to its therapeutic efficacy in managing OAB. It has been reported to diminish the frequency of uninhibited contractions and delay the initial desire to void .
3. Quality of Life Improvement:
Long-term studies have indicated that extended-release formulations of oxybutynin significantly improve quality of life for individuals suffering from OAB. In a multicenter study involving 1,067 participants, significant improvements were noted in quality-of-life measures over a 12-month period .
Case Studies and Clinical Findings
Case Study: Crystalline Change from Salt to Free Base
A recent case study highlighted the transition from oxybutynin hydrochloride to its free base form. This change was associated with reduced side effects and improved drug delivery efficiency. The study emphasized that this formulation change not only enhanced patient compliance but also minimized adverse reactions such as dry mouth, a common side effect associated with traditional formulations .
Clinical Efficacy in Pediatric Populations:
Oxybutynin has also been evaluated for safety and efficacy in pediatric populations with neurogenic bladder conditions. Findings suggest that oxybutynin is both safe and effective for treating such conditions, with tolerability profiles comparable to those seen in adult populations .
Comparative Analysis of Formulations
The following table summarizes key pharmacokinetic parameters and tolerability profiles across different formulations of oxybutynin:
Formulation | Cmax (ng/mL) | Tmax (hr) | Half-life (hr) | Common Side Effects |
---|---|---|---|---|
Immediate Release | 6.1 ± 3.2 | 1.0 | 2 | Dry mouth, constipation |
Extended Release | 10.1 ± 7.5 | 1.0 | 2 | Reduced dry mouth |
Transdermal System | Varies | 48 | 62-84 | Least dry mouth |
This comparative analysis indicates that while all formulations are effective, the transdermal system offers improved tolerability due to lower peak concentrations and sustained release profiles.
Q & A
Basic Research Questions
Q. What is the pharmacological mechanism of Oxybutynin-d11 chloride in inhibiting vascular Kv channels?
this compound acts as an anticholinergic agent, inhibiting vascular Kv channels in a concentration-dependent manner with an IC50 of 11.51 μM . Methodologically, researchers should validate this mechanism using patch-clamp electrophysiology in vascular smooth muscle cells, ensuring proper controls (e.g., vehicle and non-deuterated Oxybutynin chloride) to isolate deuterium-specific effects. Dose-response curves should be constructed across a logarithmic concentration range (e.g., 1 nM–100 μM) to confirm the IC50 .
Q. How can researchers verify the purity and deuteration level of synthesized this compound?
Analytical techniques such as high-performance liquid chromatography (HPLC) with UV detection (purity >98% ) and mass spectrometry (MS) should be employed. Nuclear magnetic resonance (<sup>2</sup>H-NMR) or isotope ratio MS is critical for quantifying deuterium incorporation at specific positions. Cross-referencing with synthetic protocols (e.g., deuterated precursor ratios) ensures batch consistency .
Q. What experimental controls are essential when assessing muscarinic receptor subtype selectivity?
Use subtype-specific antagonists (e.g., PD 102807 for M4 receptors, IC50 = 90.7 nM ) in competitive binding assays. Include positive controls (e.g., atropine for pan-muscarinic inhibition) and negative controls (e.g., untransfected cells). Data should be normalized to receptor expression levels via radioligand saturation binding .
Advanced Research Questions
Q. How does deuterium labeling alter the metabolic stability of this compound compared to its non-deuterated form?
Deuterium’s kinetic isotope effect slows CYP450-mediated metabolism. To evaluate this, conduct in vitro hepatic microsomal assays with LC-MS/MS quantification of parent compound and metabolites. Compare half-life (t1/2) and intrinsic clearance (CLint) between deuterated and non-deuterated forms. Statistical analysis (e.g., paired t-tests) should confirm significance (p < 0.05) .
Q. What strategies resolve discrepancies in reported IC50 values for Kv channel inhibition across studies?
Variability may arise from differences in cell type (e.g., vascular vs. neuronal Kv channels) or assay conditions (e.g., temperature, ion concentrations). Replicate experiments using standardized protocols (e.g., HEK293 cells expressing Kv1.5). Meta-analyses of published data should account for covariates via multivariate regression .
Q. How can researchers optimize experimental designs to isolate deuterium-specific effects in in vivo models?
Use paired dosing in animal studies: administer this compound and its non-deuterated counterpart to the same subject cohort, separated by a washout period. Pharmacokinetic parameters (AUC, Cmax) should be compared using non-compartmental analysis. Tissue distribution studies via quantitative whole-body autoradiography can further localize isotopic effects .
Q. Methodological Considerations
Q. What statistical approaches are recommended for analyzing concentration-response data in anticholinergic assays?
Fit data to a four-parameter logistic model (e.g., Hill equation) using nonlinear regression. Report 95% confidence intervals for IC50 values. For receptor selectivity, calculate selectivity ratios (e.g., M4/M1 = IC50,M1/IC50,M4) and validate via bootstrap resampling .
Q. How should researchers address potential isotopic exchange in stability studies?
Monitor deuterium retention in biological matrices (e.g., plasma, liver homogenates) using stable isotope tracing . Accelerated stability studies (e.g., 40°C/75% RH) can predict long-term exchange rates. Data should be reported as % deuterium remaining over time, with degradation kinetics modeled via first-order decay .
Q. Data Presentation & Reproducibility
Q. What guidelines ensure reproducibility in reporting deuterated compound synthesis?
Follow CHEM21 guidelines for synthetic details: document deuterium sources (e.g., D2O, deuterated solvents), reaction conditions (time, temperature), and purification methods (e.g., column chromatography gradients). Provide <sup>1</sup>H/<sup>13</sup>C/<sup>2</sup>H NMR spectra in supplementary materials .
Q. How can researchers validate computational models predicting deuterium’s impact on receptor binding?
Combine molecular dynamics simulations (e.g., GROMACS) with experimental binding data. Use free-energy perturbation (FEP) to calculate ΔΔG for deuterated vs. non-deuterated forms. Validate predictions via isothermal titration calorimetry (ITC) to measure binding enthalpy .
Properties
IUPAC Name |
4-(diethylamino)but-2-ynyl 2-hydroxy-2-phenyl-2-(1,2,2,3,3,4,4,5,5,6,6-undecadeuteriocyclohexyl)acetate;hydrochloride | |
---|---|---|
Source | PubChem | |
URL | https://pubchem.ncbi.nlm.nih.gov | |
Description | Data deposited in or computed by PubChem | |
InChI |
InChI=1S/C22H31NO3.ClH/c1-3-23(4-2)17-11-12-18-26-21(24)22(25,19-13-7-5-8-14-19)20-15-9-6-10-16-20;/h5,7-8,13-14,20,25H,3-4,6,9-10,15-18H2,1-2H3;1H/i6D2,9D2,10D2,15D2,16D2,20D; | |
Source | PubChem | |
URL | https://pubchem.ncbi.nlm.nih.gov | |
Description | Data deposited in or computed by PubChem | |
InChI Key |
SWIJYDAEGSIQPZ-JPELPYLWSA-N | |
Source | PubChem | |
URL | https://pubchem.ncbi.nlm.nih.gov | |
Description | Data deposited in or computed by PubChem | |
Canonical SMILES |
CCN(CC)CC#CCOC(=O)C(C1CCCCC1)(C2=CC=CC=C2)O.Cl | |
Source | PubChem | |
URL | https://pubchem.ncbi.nlm.nih.gov | |
Description | Data deposited in or computed by PubChem | |
Isomeric SMILES |
[2H]C1(C(C(C(C(C1([2H])[2H])([2H])[2H])([2H])C(C2=CC=CC=C2)(C(=O)OCC#CCN(CC)CC)O)([2H])[2H])([2H])[2H])[2H].Cl | |
Source | PubChem | |
URL | https://pubchem.ncbi.nlm.nih.gov | |
Description | Data deposited in or computed by PubChem | |
Molecular Formula |
C22H32ClNO3 | |
Source | PubChem | |
URL | https://pubchem.ncbi.nlm.nih.gov | |
Description | Data deposited in or computed by PubChem | |
Molecular Weight |
405.0 g/mol | |
Source | PubChem | |
URL | https://pubchem.ncbi.nlm.nih.gov | |
Description | Data deposited in or computed by PubChem | |
Disclaimer and Information on In-Vitro Research Products
Please be aware that all articles and product information presented on BenchChem are intended solely for informational purposes. The products available for purchase on BenchChem are specifically designed for in-vitro studies, which are conducted outside of living organisms. In-vitro studies, derived from the Latin term "in glass," involve experiments performed in controlled laboratory settings using cells or tissues. It is important to note that these products are not categorized as medicines or drugs, and they have not received approval from the FDA for the prevention, treatment, or cure of any medical condition, ailment, or disease. We must emphasize that any form of bodily introduction of these products into humans or animals is strictly prohibited by law. It is essential to adhere to these guidelines to ensure compliance with legal and ethical standards in research and experimentation.