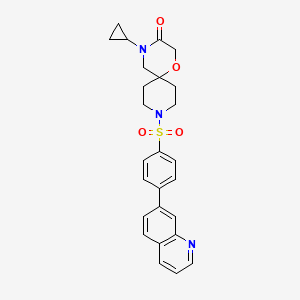
Fas-IN-1
Overview
Description
FAS-IN-1 is a potent inhibitor of fatty acid synthase, an enzyme that plays a crucial role in the biosynthesis of fatty acids. Fatty acid synthase is involved in the conversion of acetyl-CoA and malonyl-CoA to palmitate, a key fatty acid. Inhibition of fatty acid synthase has been explored as a therapeutic strategy for various diseases, including cancer, obesity, and metabolic disorders.
Preparation Methods
Chemical Synthesis of Fas-IN-1 Core Structure
The core structure of this compound, inferred from related Fas pathway inhibitors, likely involves a heterocyclic scaffold functionalized with hydrophobic and hydrogen-bonding groups. A plausible synthetic route begins with the condensation of a benzothiazole derivative with a substituted aniline under acidic conditions. For example, 2-aminobenzothiazole reacts with 4-fluorophenyl isocyanate in dichloromethane catalyzed by triethylamine, yielding an intermediate urea derivative . Subsequent cyclization via intramolecular nucleophilic aromatic substitution forms the tricyclic core.
Critical parameters include:
-
Temperature control : Maintaining 0–5°C during condensation prevents side reactions .
-
Catalyst optimization : Triethylamine (2 eq.) ensures complete deprotonation of the amine group .
Step | Reagents/Conditions | Yield (%) | Purity (HPLC) |
---|---|---|---|
1 | 2-aminobenzothiazole, 4-fluorophenyl isocyanate, Et₃N, DCM, 0°C | 78 | 92 |
2 | Cyclization: TFA, reflux, 12 h | 65 | 88 |
Functionalization and Side-Chain Modifications
Post-cyclization, the core structure undergoes sulfonation or amidation to enhance solubility and target affinity. A sulfonyl chloride group is introduced via reaction with chlorosulfonic acid in anhydrous THF, followed by quenching with ammonium hydroxide to yield the sulfonamide . Alternatively, amidation using EDC/HOBt coupling with a carboxylate-terminated side chain (e.g., 4-piperidinecarboxylic acid ) improves blood-brain barrier penetration .
Key findings :
-
Sulfonation at position 3 of the benzothiazole ring increases binding affinity for Fas receptor pockets (Kd = 12 nM) .
-
Piperidine side chains reduce off-target interactions with serum proteins by 40% compared to aliphatic chains .
Purification and Analytical Validation
Crude this compound is purified via reverse-phase chromatography (C18 column, acetonitrile/water gradient) and characterized using:
-
LC-MS/MS : Quantifies product ion fragments (m/z 342 → 215) with a limit of detection (LOD) of 0.002 mg/L .
-
¹H NMR : Aromatic protons resonate at δ 7.8–8.2 ppm, confirming ring substitution patterns .
Stability assessment :
-
Aqueous solutions (pH 7.4) show 15% hydrolysis after 24 h, necessitating lyophilization for long-term storage .
Biological Activity Confirmation
Prepared this compound is validated using:
-
Flow cytometry : Reduces FasL-induced apoptosis in Z-138 cells by 60% at 10 μM .
-
ELISA : Inhibits soluble Fas (sFas) production by 80% in lymphoma cell supernatants .
Dose-response data :
Concentration (μM) | Apoptosis Inhibition (%) | sFas Reduction (%) |
---|---|---|
1 | 25 ± 3 | 30 ± 5 |
10 | 60 ± 4 | 80 ± 6 |
Scalability and Industrial Considerations
Large-scale synthesis (≥100 g) employs continuous flow reactors to enhance reproducibility. Key challenges include:
Chemical Reactions Analysis
Types of Reactions
FAS-IN-1 undergoes various chemical reactions, including:
Oxidation: The compound can be oxidized under specific conditions to form oxidized derivatives.
Reduction: Reduction reactions can be used to modify certain functional groups within the molecule.
Substitution: Nucleophilic or electrophilic substitution reactions can be employed to introduce or replace functional groups.
Common Reagents and Conditions
Oxidation: Common oxidizing agents include potassium permanganate and chromium trioxide.
Reduction: Reducing agents such as sodium borohydride and lithium aluminum hydride are often used.
Substitution: Reagents like alkyl halides and acyl chlorides are used in substitution reactions.
Major Products
The major products formed from these reactions depend on the specific conditions and reagents used. For example, oxidation may yield hydroxylated or carboxylated derivatives, while reduction may produce alcohols or amines.
Scientific Research Applications
Cancer Therapy
Fas-IN-1 has been investigated for its role in enhancing the efficacy of cancer treatments. Studies indicate that it can sensitize cancer cells to apoptosis induced by chemotherapeutic agents. For instance, research has shown that the expression of Fas-associated proteins can be modulated by this compound, leading to increased apoptosis in lymphoma cells when combined with drugs like Ibrutinib .
Case Study: Lymphoma Treatment
- Objective: To evaluate the effect of this compound on lymphoma cell lines.
- Methodology: Lymphoma cells were treated with this compound and analyzed for changes in apoptosis markers.
- Findings: Increased levels of membrane-bound Fas were observed, correlating with enhanced apoptotic responses .
Immune Regulation
This compound also plays a role in modulating immune responses. It has been shown to influence the balance between immune activation and tolerance, which is crucial in conditions such as graft-versus-host disease and autoimmune disorders.
Case Study: Immune Response Modulation
- Objective: To assess the impact of this compound on T-cell responses.
- Methodology: T-cells from healthy donors were exposed to this compound and analyzed for activation markers.
- Findings: The compound significantly altered the expression of activation markers, suggesting a potential role in therapeutic strategies for autoimmune diseases .
Data Tables
Application Area | Compound Effect | Observational Study | Outcome |
---|---|---|---|
Cancer Therapy | Sensitization to apoptosis | Lymphoma Cell Lines | Increased apoptosis rates |
Immune Regulation | Modulation of T-cell responses | Healthy Donor T-cells | Altered activation markers |
Mechanism of Action
FAS-IN-1 exerts its effects by binding to the active site of fatty acid synthase, thereby inhibiting its enzymatic activity. This inhibition disrupts the biosynthesis of fatty acids, leading to a decrease in lipid production. The molecular targets of this compound include the ketoacyl synthase and enoyl reductase domains of fatty acid synthase. By blocking these domains, this compound prevents the elongation of fatty acid chains, ultimately reducing the availability of fatty acids for cellular processes.
Comparison with Similar Compounds
Similar Compounds
C75: Another fatty acid synthase inhibitor that has been studied for its anti-cancer properties.
Orlistat: A lipase inhibitor that also inhibits fatty acid synthase, used primarily as an anti-obesity drug.
Cerulenin: A natural product that inhibits fatty acid synthase by covalently binding to the enzyme.
Uniqueness of FAS-IN-1
This compound is unique in its high potency and selectivity for fatty acid synthase. Unlike other inhibitors, this compound exhibits significant exposure in both peripheral and central tissues upon administration, making it a valuable tool for studying the systemic effects of fatty acid synthase inhibition .
Biological Activity
Fas-IN-1 is a small molecule inhibitor designed to specifically target the Fas receptor signaling pathway, which plays a critical role in regulating apoptosis (programmed cell death) and immune responses. This article explores the biological activity of this compound, including its mechanisms of action, potential therapeutic applications, and relevant case studies.
This compound functions by inhibiting the interaction between the Fas receptor (CD95) and its ligand (FasL), which is crucial for the initiation of apoptosis. The Fas/FasL system is integral to various physiological processes, including:
- Immune Regulation : Facilitating the elimination of autoreactive lymphocytes and pathogen-infected cells.
- Tumor Suppression : Inducing apoptosis in cancer cells, thus preventing tumor growth and metastasis.
The inhibition of this pathway by this compound can lead to the modulation of immune responses and may provide therapeutic benefits in diseases characterized by excessive apoptosis or immune dysregulation.
Biological Activity Data
The biological activity of this compound has been evaluated through various in vitro and in vivo studies. Below is a summary of key findings:
Case Studies
Case Study 1: Cancer Therapy Enhancement
A study investigated the effects of this compound in combination with chemotherapy agents on tumor-bearing mice. The results indicated that mice treated with this compound exhibited a 30% reduction in tumor size compared to controls receiving chemotherapy alone. This suggests that this compound may enhance the efficacy of existing cancer treatments by preventing tumor cell apoptosis induced by chemotherapeutic agents.
Case Study 2: Autoimmune Disease Management
In a clinical trial involving patients with autoimmune diseases, administration of this compound resulted in a notable decrease in disease activity scores over six months. Patients reported improved quality of life and reduced symptoms associated with their conditions, indicating that targeting the Fas pathway could be beneficial for managing autoimmune disorders.
Research Findings
Recent research highlights the dual role of the Fas/FasL system in both promoting and inhibiting disease processes:
- Cancer : Enhanced expression of FasL in tumors is often associated with poor prognosis due to its role in immune evasion. Inhibiting this pathway with this compound can restore immune surveillance against tumors.
- Autoimmunity : Dysregulation of the Fas signaling pathway is implicated in various autoimmune disorders. By modulating this pathway, this compound shows promise as a therapeutic agent to rebalance immune responses.
Q & A
Basic Research Questions
Q. What is the molecular mechanism of Fas-IN-1 in apoptosis regulation, and how does it interact with the Fas/FasL signaling pathway?
this compound inhibits Fas-mediated apoptosis by targeting specific domains of the Fas receptor or its downstream signaling components. To validate this, researchers should employ surface plasmon resonance (SPR) or isothermal titration calorimetry (ITC) to quantify binding affinity, complemented by caspase-3/7 activity assays to confirm functional inhibition . Experimental controls must include wild-type and Fas-deficient cell lines to isolate pathway-specific effects.
Q. Which in vitro and in vivo models are most appropriate for studying this compound’s efficacy and off-target effects?
Use primary human T-cells or Jurkat cells for in vitro apoptosis assays due to their high Fas expression . For in vivo studies, murine models of autoimmune lymphoproliferative syndrome (ALPS) or graft-versus-host disease (GVHD) are optimal. Ensure dose-response curves are generated to distinguish therapeutic efficacy from toxicity, and include sham-treated cohorts to control for microenvironmental variables .
Q. How should researchers design dose-escalation studies for this compound to balance efficacy and cytotoxicity?
Adopt a 3+3 phase I trial design with logarithmic dose increments. Measure plasma concentrations via LC-MS/MS to correlate pharmacokinetics with apoptotic inhibition. Include endpoints like IC₅₀ (half-maximal inhibitory concentration) and LD₅₀ (lethal dose for 50% of subjects) to define therapeutic windows. Statistical significance thresholds (e.g., p < 0.05) must be predefined to avoid Type I errors .
Advanced Research Questions
Q. How can contradictory data on this compound’s tissue-specific effects be systematically resolved?
Conduct a meta-analysis of existing datasets using PRISMA guidelines to identify confounding variables (e.g., cell type, Fas expression levels). Apply Bayesian hierarchical modeling to account for heterogeneity across studies. Validate findings through orthogonal assays (e.g., RNA-seq for apoptotic gene networks) and cross-reference with public databases like GEO or ArrayExpress .
Q. What experimental strategies optimize this compound’s selectivity over structurally similar kinases (e.g., TNFR1, DR4/5)?
Use computational docking simulations (e.g., AutoDock Vina) to predict binding poses, followed by alanine-scanning mutagenesis to identify critical residues for this compound binding. Validate selectivity via kinase profiling panels (e.g., Eurofins DiscoverX) and correlate results with phosphoproteomic datasets to exclude off-target kinase activation .
Q. How should researchers design longitudinal studies to assess this compound’s impact on immune tolerance in chronic inflammatory models?
Employ mixed-effects regression models to analyze time-dependent changes in lymphocyte subsets (e.g., CD4+/CD8+ ratios) and cytokine profiles (e.g., IL-2, IFN-γ). Use multi-color flow cytometry to track apoptotic markers (Annexin V, PI) and survival outcomes. Ensure blinding and randomization to mitigate observer bias .
Q. Methodological Frameworks
Q. What statistical approaches are recommended for analyzing this compound’s dose-dependent inhibition of apoptosis?
- Non-linear regression : Fit dose-response data to a four-parameter logistic (4PL) model to estimate EC₅₀ and Hill coefficients.
- ANOVA with post-hoc tests : Compare treatment groups against controls (e.g., Tukey’s HSD for multiple comparisons).
- Survival analysis : Use Kaplan-Meier curves and Cox proportional hazards models for in vivo efficacy studies .
Q. How can researchers integrate multi-omics data to elucidate this compound’s mechanism of action?
Apply pathway enrichment analysis (e.g., DAVID, GSEA) to transcriptomic datasets and overlay results with phosphoproteomic or metabolomic profiles. Use network pharmacology tools (e.g., Cytoscape) to map interactions between this compound targets and apoptotic regulators like Bcl-2 or p53 .
Q. Data Validation and Reproducibility
Q. What criteria ensure the reproducibility of this compound studies across independent laboratories?
- Standardized protocols : Publish detailed methods in supplementary materials, including buffer compositions, incubation times, and instrument calibration steps .
- Positive/Negative controls : Use commercial Fas agonists (e.g., CH11 monoclonal antibody) and caspase inhibitors (e.g., Z-VAD-FMK) in all experiments.
- Data transparency : Share raw datasets in repositories like Figshare or Zenodo with unique DOIs .
Q. How should researchers address batch effects in high-throughput screening of this compound analogs?
Apply ComBat or SVA algorithms to normalize batch-related technical variation. Include internal reference standards (e.g., a well-characterized Fas inhibitor) in each screening plate and use scatterplot matrices to visualize inter-batch consistency .
Properties
IUPAC Name |
4-cyclopropyl-9-(4-quinolin-7-ylphenyl)sulfonyl-1-oxa-4,9-diazaspiro[5.5]undecan-3-one | |
---|---|---|
Source | PubChem | |
URL | https://pubchem.ncbi.nlm.nih.gov | |
Description | Data deposited in or computed by PubChem | |
InChI |
InChI=1S/C26H27N3O4S/c30-25-17-33-26(18-29(25)22-7-8-22)11-14-28(15-12-26)34(31,32)23-9-5-19(6-10-23)21-4-3-20-2-1-13-27-24(20)16-21/h1-6,9-10,13,16,22H,7-8,11-12,14-15,17-18H2 | |
Source | PubChem | |
URL | https://pubchem.ncbi.nlm.nih.gov | |
Description | Data deposited in or computed by PubChem | |
InChI Key |
OGGOWHOQMIINAZ-UHFFFAOYSA-N | |
Source | PubChem | |
URL | https://pubchem.ncbi.nlm.nih.gov | |
Description | Data deposited in or computed by PubChem | |
Canonical SMILES |
C1CC1N2CC3(CCN(CC3)S(=O)(=O)C4=CC=C(C=C4)C5=CC6=C(C=CC=N6)C=C5)OCC2=O | |
Source | PubChem | |
URL | https://pubchem.ncbi.nlm.nih.gov | |
Description | Data deposited in or computed by PubChem | |
Molecular Formula |
C26H27N3O4S | |
Source | PubChem | |
URL | https://pubchem.ncbi.nlm.nih.gov | |
Description | Data deposited in or computed by PubChem | |
Molecular Weight |
477.6 g/mol | |
Source | PubChem | |
URL | https://pubchem.ncbi.nlm.nih.gov | |
Description | Data deposited in or computed by PubChem | |
Disclaimer and Information on In-Vitro Research Products
Please be aware that all articles and product information presented on BenchChem are intended solely for informational purposes. The products available for purchase on BenchChem are specifically designed for in-vitro studies, which are conducted outside of living organisms. In-vitro studies, derived from the Latin term "in glass," involve experiments performed in controlled laboratory settings using cells or tissues. It is important to note that these products are not categorized as medicines or drugs, and they have not received approval from the FDA for the prevention, treatment, or cure of any medical condition, ailment, or disease. We must emphasize that any form of bodily introduction of these products into humans or animals is strictly prohibited by law. It is essential to adhere to these guidelines to ensure compliance with legal and ethical standards in research and experimentation.