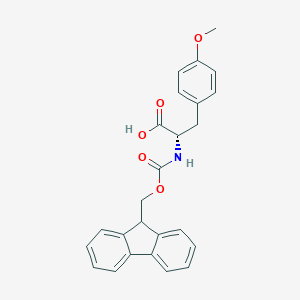
Fmoc-tyr(ME)-OH
Overview
Description
Fmoc-Tyr(ME)-OH (9-fluorenylmethoxycarbonyl-O-methylphosphotyrosine) is a protected tyrosine derivative widely used in solid-phase peptide synthesis (SPPS) to incorporate phosphorylated tyrosine residues into peptides. The methyl ester (ME) group protects the phosphate moiety, preventing undesired side reactions during peptide assembly. This compound addresses challenges posed by unprotected phosphates (e.g., Fmoc-Tyr(PO3H2)-OH), which are prone to hydrolysis and β-elimination under basic SPPS conditions .
Key structural features:
- Fmoc group: Protects the α-amino group during SPPS.
- Methyl ester (ME): Stabilizes the phosphate group, enabling efficient coupling.
- Phosphotyrosine core: Mimics post-translational modifications critical for studying signaling pathways.
Preparation Methods
Synthetic Routes for Fmoc-Tyr(Me)-OH
Fmoc Protection of Tyrosine
The amino group of tyrosine is protected using Fmoc-Cl (fluorenylmethyloxycarbonyl chloride) under basic conditions. Sodium bicarbonate (NaHCO₃) or N,N-diisopropylethylamine (DIPEA) is typically employed to deprotonate the amino group, facilitating nucleophilic attack on Fmoc-Cl . The reaction proceeds in tetrahydrofuran (THF) or dimethylformamide (DMF) at 0–25°C, yielding Fmoc-Tyr-OH as an intermediate .
Methylation of the Phenolic Hydroxyl Group
The hydroxyl group on tyrosine’s aromatic ring is methylated using methyl iodide (MeI) in the presence of a strong base such as potassium carbonate (K₂CO₃) or sodium hydride (NaH) . This step is conducted in anhydrous DMF or acetone under inert atmosphere (e.g., nitrogen) to prevent demethylation . The reaction typically achieves >95% conversion, as confirmed by thin-layer chromatography (TLC) .
Purification and Isolation
Crude this compound is purified via:
-
Flash chromatography using silica gel and gradients of ethyl acetate in hexane .
-
High-performance liquid chromatography (HPLC) for analytical validation, with purity ≥99% (area%) .
Analytical Validation of Synthetic Products
Purity Assessment
Parameter | Method | Specification | Source |
---|---|---|---|
Purity (HPLC) | Reverse-phase C18 column | ≥99.0% (area%) | |
Enantiomeric purity | Chiral HPLC | ≥99.5% (a/a) | |
Water content | Karl Fischer titration | ≤1.00% |
Solubility Profile
This compound exhibits solubility in polar aprotic solvents:
Industrial-Scale Production
Process Optimization
Industrial synthesis employs automated peptide synthesizers to ensure batch consistency . Key parameters include:
-
Temperature control : 2–30°C during storage to prevent decomposition .
-
Reagent stoichiometry : 1.2 equivalents of Fmoc-Cl for complete amino protection .
Quality Control Metrics
Comparative Analysis of Synthetic Methods
Traditional vs. Industrial Methods
Aspect | Laboratory-Scale | Industrial-Scale |
---|---|---|
Reaction time | 12–24 hours | 6–8 hours (optimized) |
Yield | 70–80% | 85–90% |
Purity (HPLC) | ≥98% | ≥99% |
Challenges in Methylation
-
Incomplete methylation : Residual hydroxyl groups may persist, requiring iterative MeI treatment .
-
Side reactions : Over-methylation of carboxyl groups is mitigated by controlling base stoichiometry .
Applications in Peptide Synthesis
This compound is integral to synthesizing tyrosine-containing peptides resistant to oxidation. Its methyl ether group enhances stability during SPPS, particularly in sequences requiring prolonged coupling times . Post-synthesis, the Fmoc group is removed using 20% piperidine in DMF, while the methyl ether remains intact under standard cleavage conditions .
Chemical Reactions Analysis
Coupling Reactions in SPPS
Fmoc-Tyr(Me)-OH exhibits efficient coupling kinetics using uronium/guanidinium-based activators. Comparative coupling efficiencies are summarized below:
Coupling Reagent | Base | Coupling Efficiency | Side Reactions | Reference |
---|---|---|---|---|
HATU | DIPEA | >98% | Minimal acylation byproducts | |
DIC/Oxyma | – | 95–97% | Occasional racemization (<1%) | |
PyBOP® | DIPEA | 90–92% | Reduced solubility in DMF |
For challenging sequences (e.g., sterically hindered residues), double coupling with 4 equivalents of this compound and extended reaction times (2–4 hours) are recommended .
Orthogonal Stability Profiles
The methyl ether and Fmoc groups display orthogonal stability, enabling selective modifications:
Functional Group | Stability | Deprotection Method |
---|---|---|
Fmoc | Acid-stable, base-labile | 20% piperidine/DMF |
O-Methyl ether | Stable under basic/acidic SPPS conditions | Requires strong acids (e.g., TFA/HF) |
Notably, the methyl ether remains intact during standard TFA-mediated resin cleavage (95% TFA, 2.5% H₂O, 2.5% TIPS) .
Comparative Analysis with Other Tyrosine Derivatives
Key differences between this compound and phosphorylated/sulfated tyrosine analogs:
Troubleshooting Common Issues
Biological Activity
Fmoc-tyrosine methyl ester (Fmoc-tyr(ME)-OH) is a derivative of the amino acid tyrosine, widely utilized in peptide synthesis due to its protective Fmoc (9-fluorenylmethoxycarbonyl) group. This compound exhibits significant biological activity, particularly in the context of peptide synthesis and modification, influencing various biochemical pathways and interactions. This article explores the biological activity of this compound, emphasizing its applications, mechanisms of action, and relevant case studies.
Overview of this compound
This compound is characterized by its protective Fmoc group that allows for selective coupling during solid-phase peptide synthesis (SPPS). The methyl ester group enhances the lipophilicity of the tyrosine residue, affecting its interaction with biological membranes and proteins.
Biological Activity
1. Peptide Synthesis:
- This compound is primarily used in SPPS to introduce tyrosine residues into peptides. The Fmoc group facilitates easy removal under basic conditions, allowing for subsequent coupling reactions without interfering with the peptide backbone .
- Table 1: Comparison of Different Tyrosine Derivatives in Peptide Synthesis
Derivative | Fmoc Group | Biological Activity | Stability |
---|---|---|---|
This compound | Yes | High | Moderate |
Fmoc-Tyr(SO3nP)-OH | Yes | Modulates interactions | High |
Fmoc-Tyr(Ph)-OH | Yes | Antioxidant properties | Low |
2. Modulation of Protein Interactions:
- Tyrosine residues play a crucial role in protein-protein interactions and signaling pathways. The methyl ester form can influence these interactions by altering the hydrophobicity and steric properties of peptides.
- In studies involving sulfated tyrosine derivatives, it was found that sulfation enhances binding affinity to certain receptors, suggesting that modifications like those introduced by this compound may similarly affect biological activity through structural changes .
3. Case Studies:
- A study demonstrated the utility of this compound in synthesizing biologically active peptides that mimic natural hormone structures. These peptides exhibited significant activity in modulating cellular responses related to metabolism and growth factors .
- Another research highlighted the role of modified tyrosine residues in enhancing the stability and efficacy of peptide-based drugs, showcasing how this compound can be strategically employed to improve pharmacological profiles .
The biological activity of this compound can be attributed to several mechanisms:
1. Structural Influence:
- The introduction of a methyl ester alters the conformation of peptides, potentially enhancing their ability to interact with target proteins or receptors.
2. Enhanced Lipophilicity:
- The methyl group increases the lipophilicity of the tyrosine residue, which can facilitate membrane permeability and improve bioavailability in therapeutic applications .
3. Post-translational Modifications:
Scientific Research Applications
Peptide Synthesis
Solid-Phase Peptide Synthesis (SPPS)
Fmoc-tyrosine(ME)-OH is primarily utilized as a building block in SPPS, enabling the synthesis of complex peptides with high purity and yield. It allows for the sequential addition of amino acids to a growing peptide chain while maintaining the integrity of the Fmoc protecting group during coupling reactions.
Case Study: Synthesis of Bioactive Peptides
In a study focused on synthesizing neuropeptides, researchers employed Fmoc-tyrosine(ME)-OH to construct peptides that interact with specific receptors in the brain. The resulting peptides showed enhanced binding affinity and selectivity due to the strategic incorporation of tyrosine residues .
Drug Development
Peptide-Based Therapeutics
Fmoc-tyrosine(ME)-OH is instrumental in developing peptide-based drugs aimed at treating various diseases, including cancer and metabolic disorders. The compound's ability to enhance the stability and bioavailability of therapeutic peptides makes it a valuable tool in medicinal chemistry.
Case Study: Targeting Cancer Cells
A research project demonstrated that peptides synthesized using Fmoc-tyrosine(ME)-OH exhibited improved pharmacokinetic properties. These peptides were designed to target cancer cells specifically, leading to reduced side effects compared to traditional chemotherapy agents .
Bioconjugation
Targeted Delivery Systems
The compound is also utilized in bioconjugation processes, where it aids in attaching peptides to other biomolecules such as antibodies or drugs. This application is crucial for developing targeted delivery systems in cancer therapy and other medical applications.
Case Study: Antibody-Peptide Conjugates
In an experiment involving antibody-drug conjugates, researchers used Fmoc-tyrosine(ME)-OH to create stable linkages between therapeutic peptides and monoclonal antibodies. This approach enhanced the specificity of drug delivery to tumor cells while minimizing systemic toxicity .
Protein Engineering
Structure-Function Studies
Fmoc-tyrosine(ME)-OH plays a significant role in protein engineering by enabling modifications that help elucidate structure-function relationships. This application is vital for designing proteins with enhanced properties for industrial purposes.
Case Study: Enzyme Modification
A study focused on modifying enzymes for industrial applications utilized Fmoc-tyrosine(ME)-OH to introduce specific functionalities into enzyme structures. The modified enzymes demonstrated improved catalytic efficiency and stability under harsh conditions .
Neuroscience Research
Neuropeptides and Neurological Studies
In neuroscience, Fmoc-tyrosine(ME)-OH is employed in studies related to neuropeptides, contributing to understanding neurological functions and potential treatments for neurodegenerative diseases.
Case Study: Investigating Neuropeptide Functions
Research investigating the role of neuropeptides in pain signaling pathways utilized Fmoc-tyrosine(ME)-OH to synthesize peptides that mimic natural neuropeptides. These synthesized peptides provided insights into receptor interactions and potential therapeutic targets for pain management .
Q & A
Basic Research Questions
Q. What are the recommended methods for synthesizing peptides using Fmoc-Tyr(ME)-OH in solid-phase peptide synthesis (SPPS)?
this compound is incorporated into peptide chains via standard SPPS protocols. Key steps include:
- Activation : Use carbodiimide-based coupling reagents (e.g., HBTU/HOBt) in DMF to activate the carboxyl group.
- Coupling : Employ a 2-4-fold molar excess of this compound relative to resin-bound amines, with reaction times of 30–60 minutes.
- Deprotection : Remove the Fmoc group using 20% piperidine in DMF.
- Methyl Ester Stability : The methyl ester (ME) group remains intact during Fmoc deprotection but requires acidic conditions (e.g., TFA) for final cleavage from the resin .
Q. How should this compound be stored to maintain stability?
Store the compound at 2–8°C under an inert atmosphere (e.g., argon) and protect from light and moisture. Prolonged exposure to humidity or elevated temperatures can lead to ester hydrolysis or Fmoc-group degradation, compromising purity .
Q. What role does the methyl ester (ME) group play in this compound during peptide synthesis?
The ME group protects the tyrosine hydroxyl side chain, preventing undesired side reactions (e.g., oxidation or nucleophilic attacks) during SPPS. It is stable under basic Fmoc-deprotection conditions but cleaved during final TFA-mediated resin cleavage, yielding free tyrosine .
Advanced Research Questions
Q. How can researchers optimize coupling efficiency for this compound in sterically hindered peptide sequences?
- Double Coupling : Perform sequential couplings with fresh reagents to ensure complete reaction.
- Microwave Assistance : Use microwave irradiation (50°C, 10–15 W) to enhance reaction kinetics.
- Additives : Include 1-hydroxy-7-azabenzotriazole (HOAt) to improve activation efficiency in challenging environments .
Q. What analytical techniques confirm the integrity of this compound during synthesis?
- HPLC : Monitor purity using reverse-phase C18 columns (≥98% purity threshold) with UV detection at 265 nm (Fmoc absorption) .
- NMR : Characterize the methyl ester group via H NMR (δ ~3.7–3.8 ppm for -OCH) and verify aromatic protons (δ ~6.9–7.2 ppm for tyrosine) .
- Mass Spectrometry : Confirm molecular weight (MW: 417.45 g/mol) with ESI-MS or MALDI-TOF .
Q. How can the methyl ester group be selectively removed without affecting other acid-labile protections (e.g., tBu)?
Use mild acidic conditions (e.g., 0.1 M HCl in dioxane/water) to hydrolyze the ME group while preserving tBu protections. Monitor reaction progress via TLC (Rf shift) or HPLC retention time changes .
Q. What are common side reactions when using this compound, and how are they mitigated?
- Racemization : Minimize by coupling at low temperatures (0–4°C) and using HOBt/DIC activation.
- Incomplete Coupling : Address with extended reaction times or ultra-concentrated reagents (0.4 M).
- Ester Hydrolysis : Avoid prolonged exposure to basic conditions (e.g., piperidine >30 min) .
Q. How does this compound influence peptide conformational studies compared to unmodified tyrosine?
The ME group alters side-chain polarity, impacting hydrogen bonding and hydrophobic interactions. Use circular dichroism (CD) or molecular dynamics simulations to compare secondary structures (e.g., α-helices vs. β-sheets) in peptides with and without the modification .
Q. Methodological Guidelines
- Orthogonal Protection Strategies : Combine this compound with other protected residues (e.g., Fmoc-Ser(tBu)-OH) to enable selective deprotection in complex sequences .
- Data Contradiction Analysis : If HPLC/MS data conflicts with expected yields, verify reagent purity (≥98% by TLC) and resin loading efficiency via quantitative ninhydrin tests .
Comparison with Similar Compounds
Phosphorylated Tyrosine Derivatives
Fmoc-Tyr(PO(OBzl)OH)-OH
- Protecting group : Benzyl (Bzl) on one phosphate oxygen; the other remains unprotected.
- Synthesis compatibility : Compatible with most coupling reagents but requires optimized conditions (e.g., 2% DBU in DMF) to prevent partial deprotection by piperidine .
- Deprotection : Requires TMSBr/TFA for full phosphate regeneration .
- Key issues : Slow coupling efficiency and risk of benzyl group removal during SPPS .
Fmoc-Tyr(PO(NMe2)2)-OH
- Protecting group : Dimethylamide (NMe2) on both phosphate oxygens.
- Deprotection : Requires 10% aqueous TFA overnight to regenerate phosphotyrosine .
- Advantage : Eliminates coupling inefficiencies but necessitates harsh deprotection .
Fmoc-Tyr(PO3Bzl2)-OH
- Protecting group : Two benzyl groups on the phosphate.
- Synthesis compatibility : Couples smoothly but converts to Fmoc-Tyr(PO(OBzl)OH)-OH during piperidine treatment, leading to similar issues .
Sulfated Tyrosine Derivatives
Fmoc-Tyr(SO3nP)-OH
- Protecting group : Neopentyl (Np) ester for sulfate stabilization.
- Synthesis compatibility : Stable under TFA; cleaved with sodium azide/DMSO or aqueous ammonium acetate .
- Application : Used for tyrosine sulfation studies, a distinct post-translational modification .
Hydroxyl-Protected Tyrosine Derivatives
Fmoc-Tyr(tBu)-OH
- Protecting group: tert-Butyl (tBu) on the phenolic hydroxyl.
- Application : Protects the hydroxyl group during SPPS, unrelated to phosphorylation.
- Deprotection : Removed with standard TFA cleavage .
Data Table: Comparative Analysis
Compound | Protecting Group(s) | Coupling Compatibility | Deprotection Method | Key Advantages/Issues |
---|---|---|---|---|
Fmoc-Tyr(ME)-OH | Methyl ester (PO) | Moderate; requires tuning | TFA/water | Balances stability and coupling efficiency |
Fmoc-Tyr(PO(OBzl)OH)-OH | Benzyl (PO) | HBTU/HOBt with DBU | TMSBr/TFA | Risk of benzyl deprotection |
Fmoc-Tyr(PO(NMe2)2)-OH | Dimethylamide (PO) | All coupling reagents | 10% aqueous TFA, overnight | Stable coupling; harsh cleavage |
Fmoc-Tyr(SO3nP)-OH | Neopentyl (SO3) | Specific to sulfation | Sodium azide/DMSO | Stable in TFA; niche use |
Fmoc-Tyr(tBu)-OH | tert-Butyl (OH) | Standard SPPS | TFA | Simple hydroxyl protection |
Properties
IUPAC Name |
(2S)-2-(9H-fluoren-9-ylmethoxycarbonylamino)-3-(4-methoxyphenyl)propanoic acid | |
---|---|---|
Source | PubChem | |
URL | https://pubchem.ncbi.nlm.nih.gov | |
Description | Data deposited in or computed by PubChem | |
InChI |
InChI=1S/C25H23NO5/c1-30-17-12-10-16(11-13-17)14-23(24(27)28)26-25(29)31-15-22-20-8-4-2-6-18(20)19-7-3-5-9-21(19)22/h2-13,22-23H,14-15H2,1H3,(H,26,29)(H,27,28)/t23-/m0/s1 | |
Source | PubChem | |
URL | https://pubchem.ncbi.nlm.nih.gov | |
Description | Data deposited in or computed by PubChem | |
InChI Key |
JYQODLWFOPCSCS-QHCPKHFHSA-N | |
Source | PubChem | |
URL | https://pubchem.ncbi.nlm.nih.gov | |
Description | Data deposited in or computed by PubChem | |
Canonical SMILES |
COC1=CC=C(C=C1)CC(C(=O)O)NC(=O)OCC2C3=CC=CC=C3C4=CC=CC=C24 | |
Source | PubChem | |
URL | https://pubchem.ncbi.nlm.nih.gov | |
Description | Data deposited in or computed by PubChem | |
Isomeric SMILES |
COC1=CC=C(C=C1)C[C@@H](C(=O)O)NC(=O)OCC2C3=CC=CC=C3C4=CC=CC=C24 | |
Source | PubChem | |
URL | https://pubchem.ncbi.nlm.nih.gov | |
Description | Data deposited in or computed by PubChem | |
Molecular Formula |
C25H23NO5 | |
Source | PubChem | |
URL | https://pubchem.ncbi.nlm.nih.gov | |
Description | Data deposited in or computed by PubChem | |
Molecular Weight |
417.5 g/mol | |
Source | PubChem | |
URL | https://pubchem.ncbi.nlm.nih.gov | |
Description | Data deposited in or computed by PubChem | |
CAS No. |
77128-72-4 | |
Record name | N-alpha-(9-Fluorenylmethyloxycarbonyl)-O-methyl-L-tyrosine | |
Source | European Chemicals Agency (ECHA) | |
URL | https://echa.europa.eu/information-on-chemicals | |
Description | The European Chemicals Agency (ECHA) is an agency of the European Union which is the driving force among regulatory authorities in implementing the EU's groundbreaking chemicals legislation for the benefit of human health and the environment as well as for innovation and competitiveness. | |
Explanation | Use of the information, documents and data from the ECHA website is subject to the terms and conditions of this Legal Notice, and subject to other binding limitations provided for under applicable law, the information, documents and data made available on the ECHA website may be reproduced, distributed and/or used, totally or in part, for non-commercial purposes provided that ECHA is acknowledged as the source: "Source: European Chemicals Agency, http://echa.europa.eu/". Such acknowledgement must be included in each copy of the material. ECHA permits and encourages organisations and individuals to create links to the ECHA website under the following cumulative conditions: Links can only be made to webpages that provide a link to the Legal Notice page. | |
Retrosynthesis Analysis
AI-Powered Synthesis Planning: Our tool employs the Template_relevance Pistachio, Template_relevance Bkms_metabolic, Template_relevance Pistachio_ringbreaker, Template_relevance Reaxys, Template_relevance Reaxys_biocatalysis model, leveraging a vast database of chemical reactions to predict feasible synthetic routes.
One-Step Synthesis Focus: Specifically designed for one-step synthesis, it provides concise and direct routes for your target compounds, streamlining the synthesis process.
Accurate Predictions: Utilizing the extensive PISTACHIO, BKMS_METABOLIC, PISTACHIO_RINGBREAKER, REAXYS, REAXYS_BIOCATALYSIS database, our tool offers high-accuracy predictions, reflecting the latest in chemical research and data.
Strategy Settings
Precursor scoring | Relevance Heuristic |
---|---|
Min. plausibility | 0.01 |
Model | Template_relevance |
Template Set | Pistachio/Bkms_metabolic/Pistachio_ringbreaker/Reaxys/Reaxys_biocatalysis |
Top-N result to add to graph | 6 |
Feasible Synthetic Routes
Disclaimer and Information on In-Vitro Research Products
Please be aware that all articles and product information presented on BenchChem are intended solely for informational purposes. The products available for purchase on BenchChem are specifically designed for in-vitro studies, which are conducted outside of living organisms. In-vitro studies, derived from the Latin term "in glass," involve experiments performed in controlled laboratory settings using cells or tissues. It is important to note that these products are not categorized as medicines or drugs, and they have not received approval from the FDA for the prevention, treatment, or cure of any medical condition, ailment, or disease. We must emphasize that any form of bodily introduction of these products into humans or animals is strictly prohibited by law. It is essential to adhere to these guidelines to ensure compliance with legal and ethical standards in research and experimentation.