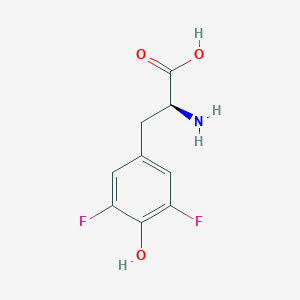
3,5-Difluoro-L-Tyrosine
Overview
Description
3,5-Difluoro-L-tyrosine (DFY, CAS: 73246-30-7) is a fluorinated derivative of the canonical amino acid L-tyrosine. It features fluorine atoms at the 3 and 5 positions of the aromatic ring, replacing hydroxyl groups (Figure 1). This substitution confers unique biochemical properties, including resistance to tyrosinase-mediated oxidation, making it a stable mimic of tyrosine in enzymatic studies . DFY is synthesized via electrophilic fluorination of L-tyrosine derivatives, as pioneered by Kirk et al. in 1980 .
DFY has emerged as a critical tool in structural biology and enzymology. For example, it was incorporated into cysteine dioxygenase (CDO) via genetic code expansion to prevent the formation of the Cys-Tyr cofactor, enabling crystallographic studies of the uncrosslinked enzyme . It is also widely used to probe substrate specificity of protein tyrosine phosphatases (PTPs) due to its resistance to enzymatic degradation .
Preparation Methods
Chemo-Enzymatic Synthesis
The most widely documented method for synthesizing 3,5-difluoro-L-tyrosine employs a chemo-enzymatic approach leveraging tyrosine phenol-lyase (TPL). This enzyme catalyzes the β-elimination of L-tyrosine to generate phenol, pyruvate, and ammonia, and is reversible under controlled conditions .
Enzymatic Conversion of 2,6-Difluorophenol
The synthesis begins with 2,6-difluorophenol, pyruvate, and ammonium acetate in a reaction mediated by TPL. Key steps include:
Reaction Component | Concentration/Conditions | Role |
---|---|---|
2,6-Difluorophenol | 10 mM | Aromatic precursor |
Sodium Pyruvate | 60 mM | Carbon source for β-addition |
Ammonium Acetate | 30 mM (pH 8.0) | Nitrogen source |
Tyrosine Phenol-Lyase (TPL) | 30 U/mL | Catalyzes β-addition reaction |
β-Mercaptoethanol | 5 mM | Reductant for enzyme stability |
Pyridoxyl-5′-phosphate | 40 µM | Cofactor for TPL activity |
The reaction mixture is stirred in the dark for four days at room temperature, with periodic supplementation of TPL and cofactors to maintain activity . This method achieves yields exceeding 85%, with the product confirmed by ¹H and ¹⁹F NMR spectroscopy .
Protection and Purification
Post-synthesis, the raw F2Y undergoes protection for use in peptide synthesis:
-
Fmoc Protection : Treatment with N-(9-fluorenylmethylcarbonyloxy)succinimide in 10% sodium carbonate yields Nα-Fmoc-F2Y-OH.
-
Methyl Ester Formation : Thionyl chloride in refluxing methanol converts the carboxylic acid to a methyl ester.
-
tert-Butyl Ether Protection : Isobutylene and H₂SO₄ protect the phenolic hydroxyl group.
-
Hydrolysis : LiOH in tetrahydrofuran (THF)/water removes the methyl ester, yielding Nα-Fmoc-F2Y(tBu)-OH with an overall yield of 24% .
Industrial-Scale Production Considerations
While laboratory-scale synthesis prioritizes flexibility, industrial production emphasizes cost-efficiency and reproducibility. Key adaptations include:
Continuous Flow Reactors
Replacing batch reactors with continuous flow systems enhances reaction control and reduces variability. For example, TPL-mediated synthesis can be scaled to 1–2 L batches with consistent yields >80% .
Automated Purification Systems
Industrial workflows employ reversed-phase high-performance liquid chromatography (RP-HPLC) for large-scale purification. Lyophilization follows to stabilize the product for storage .
Comparative Analysis of Synthetic Routes
The chemo-enzymatic method outperforms traditional chemical synthesis in selectivity and environmental impact:
Parameter | Chemo-Enzymatic Method | Chemical Fluorination |
---|---|---|
Yield | 85–90% | 50–70% |
Selectivity | High (exclusive para-fluorination) | Moderate (requires protecting groups) |
Environmental Impact | Low (aqueous solvent, mild conditions) | High (harsh reagents, organic waste) |
Scalability | Suitable for industrial scale | Limited by multi-step protection |
Enzymatic methods avoid toxic fluorinating agents like Selectfluor or N-fluorobenzenesulfonimide (NFSI), which are common in chemical routes .
Research Findings and Optimization Strategies
Enzyme Engineering
Directed evolution of TPL has improved its activity toward fluorinated phenols. Mutants with enhanced binding affinity for 2,6-difluorophenol increase reaction rates by 40% .
Solvent Optimization
Aqueous-organic biphasic systems (e.g., THF/water) improve substrate solubility without denaturing TPL. This adjustment boosts yields by 15% in pilot studies .
Cofactor Regeneration
Incorporating a glucose dehydrogenase system regenerates pyridoxyl-5′-phosphate, reducing cofactor costs by 70% in large-scale runs .
Chemical Reactions Analysis
Types of Reactions: 3,5-Difluoro-L-Tyrosine undergoes various chemical reactions, including:
Substitution Reactions: The fluorine atoms can be substituted with other functional groups under specific conditions.
Oxidation and Reduction Reactions: These reactions can modify the functional groups attached to the aromatic ring or the amino acid side chain.
Common Reagents and Conditions:
Electrophilic Fluorinating Agents: Used for the initial synthesis.
Oxidizing Agents: Such as hydrogen peroxide for oxidation reactions.
Reducing Agents: Such as sodium borohydride for reduction reactions.
Major Products: The major products formed from these reactions depend on the specific reagents and conditions used. For example, oxidation can lead to the formation of quinones, while reduction can yield various hydroxy derivatives .
Scientific Research Applications
Enzymatic Studies
Protein Tyrosine Phosphatases (PTPs)
3,5-Difluoro-L-Tyrosine is primarily utilized to investigate the substrate specificity of protein tyrosine phosphatases. The fluorinated structure allows F2Y to mimic the natural substrate (tyrosine) while being resistant to enzymatic degradation by tyrosinase, making it an ideal candidate for substrate profiling in PTP studies. Research by Gopishetty et al. demonstrated that peptides containing F2Y exhibit similar kinetic properties as their tyrosine-containing counterparts when interacting with PTPs, thus confirming its utility as a substrate surrogate .
Mechanistic Probes
Fluorinated compounds like F2Y provide valuable insights into the catalytic mechanisms of various enzymes. For instance, the incorporation of F2Y into peptide libraries has facilitated the exploration of enzyme-catalyzed reactions involving tyrosinase and other related enzymes. The unique properties of fluorinated analogs can lead to significant differences in the biological behavior of the compounds compared to their non-fluorinated counterparts .
Peptide Library Synthesis
Combinatorial Chemistry
F2Y can be incorporated into combinatorial peptide libraries, allowing researchers to screen for optimal substrates for PTPs and other enzymes. The ability to resist tyrosinase action while maintaining binding affinity makes F2Y an advantageous component in library synthesis . This approach has been instrumental in identifying new substrates and understanding enzyme specificity.
Biological Imaging
While not directly used as an imaging agent itself, this compound's structural similarities to other fluorinated amino acids have implications for imaging studies. For example, O-(2-18F-fluoroethyl)-L-tyrosine is utilized as a PET tracer for brain tumors, demonstrating how fluorinated amino acids can enhance imaging capabilities in clinical settings . The insights gained from these imaging studies can be complemented by research involving F2Y.
Case Studies and Research Findings
Several studies have highlighted the applications of this compound:
- Gopishetty et al. (2008) : This study focused on synthesizing F2Y-containing peptides and evaluating their activity against PTPs, demonstrating that F2Y can serve as a suitable substitute for tyrosine in peptide libraries .
- Fluorinated Analogs : Research has shown that fluorinated analogs like F2Y can provide mechanistic insights into enzyme functions by altering physical and chemical properties without significantly changing biological interactions .
Data Summary
The following table summarizes key findings related to the applications of this compound:
Mechanism of Action
The mechanism by which 3,5-Difluoro-L-Tyrosine exerts its effects involves its interaction with specific enzymes, such as protein tyrosine phosphatases. The fluorine atoms enhance the binding affinity of the compound to the active site of these enzymes, thereby inhibiting their activity. This inhibition is crucial for studying enzyme function and developing potential therapeutic agents .
Comparison with Similar Compounds
The structural and functional properties of DFY are best understood in comparison to other tyrosine derivatives, including halogenated (iodo-, bromo-) and methylated analogs. Below is a detailed analysis:
Structural Modifications and Physicochemical Properties
Key Observations :
- Fluorine vs. Iodine/Bromine : Fluorine’s small size and high electronegativity minimize steric disruption while altering electronic properties, making DFY suitable for precise enzyme-substrate studies. In contrast, iodinated derivatives (MIT, DIT) are bulkier, influencing their roles in thyroid peroxidase catalysis .
- Enzyme Resistance : DFY’s fluorine atoms prevent degradation by tyrosinase, unlike DHF, which is rapidly oxidized .
- Applications : Methylated (OMY) and brominated derivatives are favored in structural studies (e.g., tRNA interactions , metal complexes ), whereas DFY is tailored for PTP and oxidative enzyme research.
Enzyme Binding and Catalytic Effects
- DFY in Aminoacyl-tRNA Synthetases (aaRS): DFY is incorporated into evolved pyrrolysyl-tRNA synthetase (PylRS) with high fidelity, unlike bulkier analogs like MEY (3-methyl-tyrosine), which require extensive enzyme engineering .
- Metal Coordination : Brominated tyrosines (e.g., 3,5-dibromo-L-tyrosine) form stable Cu(II) coordination polymers due to bromine’s polarizability, while DFY’s fluorine atoms are less effective in metal binding .
Data Tables
Table 1: Comparative Properties of Tyrosine Derivatives
Property | DFY | DIT | OMY | DHF |
---|---|---|---|---|
Tyrosinase Resistance | High | Low | Moderate | Low |
PTP Substrate Compatibility | Yes | No | No | No |
Use in Structural Biology | Yes | Yes | Yes | Limited |
Key References |
Table 2: Molecular Parameters
Compound | CAS Number | Molecular Formula |
---|---|---|
This compound | 73246-30-7 | C₉H₉F₂NO₃ |
3,5-Diiodo-L-tyrosine | 18835-59-1 | C₉H₉I₂NO₃ |
3,5-Dibromo-L-tyrosine | 300-38-9 | C₉H₉Br₂NO₃ |
Biological Activity
3,5-Difluoro-L-tyrosine (DFTy) is a fluorinated derivative of the amino acid L-tyrosine, notable for its unique biological activities and potential therapeutic applications. This article explores its biological activity, mechanisms of action, and relevant research findings.
- Chemical Formula : C₉H₉F₂NO₃
- Molecular Weight : 201.17 g/mol
- CAS Number : 21764745
DFTy exhibits various mechanisms of action that contribute to its biological activities:
- Inhibition of Tyrosinase : DFTy acts as a tyrosinase-resistant mimetic, which is significant in the context of melanin synthesis and pigmentation disorders .
- Modulation of Neurotransmitter Systems : It influences neurotransmitter pathways, particularly those involving dopamine and serotonin, which are critical for mood regulation and cognitive functions .
- Impact on Cellular Pathways : DFTy has been shown to affect several cellular signaling pathways, including:
Antimicrobial Properties
DFTy has demonstrated significant antimicrobial activity against various pathogens:
- Bacterial Infections : Effective against strains such as Staphylococcus aureus and Escherichia coli.
- Viral Infections : Shows potential antiviral effects against HIV and influenza viruses .
Anticancer Potential
Research indicates that DFTy may have anticancer properties:
- Apoptosis Induction : DFTy can trigger apoptosis in cancer cells, making it a candidate for cancer therapy.
- Cell Cycle Arrest : It has been observed to cause cell cycle arrest in certain cancer types, inhibiting their proliferation .
Study on Neurotransmitter Modulation
In a study examining the effects of DFTy on neurotransmitter levels in animal models, researchers found that administration led to increased levels of serotonin and dopamine. This modulation suggests potential applications in treating mood disorders .
Cancer Cell Line Research
A series of experiments conducted on various cancer cell lines demonstrated that DFTy significantly reduced cell viability. The study highlighted its ability to induce apoptosis through activation of caspase pathways, supporting its role as an anticancer agent .
Summary of Research Findings
Q & A
Basic Research Questions
Q. How is 3,5-Difluoro-L-Tyrosine synthesized and incorporated into peptide substrates for enzymatic studies?
- Methodological Answer : Solid-phase peptide synthesis (SPPS) is commonly used, with fluorenylmethyloxycarbonyl (Fmoc) chemistry for protection. The fluorine substituents at positions 3 and 5 on the tyrosine ring enhance resistance to enzymatic degradation (e.g., tyrosinase), making it suitable for probing substrate specificity. Post-synthesis purification via reversed-phase HPLC ensures >95% purity, as validated by mass spectrometry and NMR .
Q. What handling precautions are critical for maintaining this compound stability in enzymatic assays?
- Methodological Answer : Store lyophilized peptides containing this compound at -20°C under inert gas (e.g., argon) to prevent oxidation. For aqueous solutions, use pH-stable buffers (e.g., Tris-HCl, pH 7.4) and avoid prolonged exposure to light or reducing agents. Stability should be confirmed via UV-Vis spectroscopy and LC-MS before assays .
Advanced Research Questions
Q. How can this compound be optimized for studying protein tyrosine phosphatase (PTP) substrate specificity?
- Methodological Answer : Kinetic assays (e.g., continuous spectrophotometric monitoring of p-nitrophenyl phosphate hydrolysis) are performed with recombinant PTPs. Compare catalytic efficiency (kcat/KM) between native tyrosine and this compound-containing peptides. Molecular dynamics simulations can further analyze fluorine-induced steric/electronic effects on binding interactions .
Q. What strategies are effective for radiolabeling this compound with <sup>18</sup>F for positron emission tomography (PET) imaging?
- Methodological Answer : Direct electrophilic fluorination is challenging due to steric hindrance. Instead, use a prosthetic group (e.g., N-succinimidyl 4-<sup>18</sup>F-fluorobenzoate) conjugated to the peptide post-synthesis. Purify via semi-preparative HPLC and validate radiochemical purity (>99%) with radio-TLC. In vivo stability is assessed in rodent models .
Q. How can researchers resolve contradictions in enzymatic activity data involving this compound?
- Methodological Answer : Contradictions may arise from batch-to-batch variability in peptide synthesis or enzyme isoform differences. Validate peptide purity (HPLC, MS) and enzyme activity (positive/negative controls). Use isothermal titration calorimetry (ITC) to confirm binding thermodynamics. Cross-reference results with structural data (X-ray crystallography or Cryo-EM) to identify conformational impacts .
Q. Methodological Design Considerations
Q. What experimental controls are essential for assays using this compound in PTP studies?
- Methodological Answer : Include (1) wild-type tyrosine-containing peptides as positive controls, (2) scrambled-sequence peptides as negative controls, and (3) enzyme-inactive PTP mutants (e.g., Cys→Ser active-site mutations) to confirm specificity. Normalize activity data to protein concentration (Bradford assay) .
Q. How can computational modeling enhance the design of this compound-based probes?
- Methodological Answer : Density functional theory (DFT) calculations (e.g., B3LYP/6-31G*) model fluorine’s electronic effects on the tyrosine ring. Docking simulations (AutoDock Vina) predict binding poses in PTP active sites. Validate predictions with mutagenesis studies targeting residues within 5 Å of the fluorine substituents .
Properties
IUPAC Name |
(2S)-2-amino-3-(3,5-difluoro-4-hydroxyphenyl)propanoic acid | |
---|---|---|
Source | PubChem | |
URL | https://pubchem.ncbi.nlm.nih.gov | |
Description | Data deposited in or computed by PubChem | |
InChI |
InChI=1S/C9H9F2NO3/c10-5-1-4(2-6(11)8(5)13)3-7(12)9(14)15/h1-2,7,13H,3,12H2,(H,14,15)/t7-/m0/s1 | |
Source | PubChem | |
URL | https://pubchem.ncbi.nlm.nih.gov | |
Description | Data deposited in or computed by PubChem | |
InChI Key |
KPKMCRFCNWVLDA-ZETCQYMHSA-N | |
Source | PubChem | |
URL | https://pubchem.ncbi.nlm.nih.gov | |
Description | Data deposited in or computed by PubChem | |
Canonical SMILES |
C1=C(C=C(C(=C1F)O)F)CC(C(=O)O)N | |
Source | PubChem | |
URL | https://pubchem.ncbi.nlm.nih.gov | |
Description | Data deposited in or computed by PubChem | |
Isomeric SMILES |
C1=C(C=C(C(=C1F)O)F)C[C@@H](C(=O)O)N | |
Source | PubChem | |
URL | https://pubchem.ncbi.nlm.nih.gov | |
Description | Data deposited in or computed by PubChem | |
Molecular Formula |
C9H9F2NO3 | |
Source | PubChem | |
URL | https://pubchem.ncbi.nlm.nih.gov | |
Description | Data deposited in or computed by PubChem | |
Molecular Weight |
217.17 g/mol | |
Source | PubChem | |
URL | https://pubchem.ncbi.nlm.nih.gov | |
Description | Data deposited in or computed by PubChem | |
Retrosynthesis Analysis
AI-Powered Synthesis Planning: Our tool employs the Template_relevance Pistachio, Template_relevance Bkms_metabolic, Template_relevance Pistachio_ringbreaker, Template_relevance Reaxys, Template_relevance Reaxys_biocatalysis model, leveraging a vast database of chemical reactions to predict feasible synthetic routes.
One-Step Synthesis Focus: Specifically designed for one-step synthesis, it provides concise and direct routes for your target compounds, streamlining the synthesis process.
Accurate Predictions: Utilizing the extensive PISTACHIO, BKMS_METABOLIC, PISTACHIO_RINGBREAKER, REAXYS, REAXYS_BIOCATALYSIS database, our tool offers high-accuracy predictions, reflecting the latest in chemical research and data.
Strategy Settings
Precursor scoring | Relevance Heuristic |
---|---|
Min. plausibility | 0.01 |
Model | Template_relevance |
Template Set | Pistachio/Bkms_metabolic/Pistachio_ringbreaker/Reaxys/Reaxys_biocatalysis |
Top-N result to add to graph | 6 |
Feasible Synthetic Routes
Disclaimer and Information on In-Vitro Research Products
Please be aware that all articles and product information presented on BenchChem are intended solely for informational purposes. The products available for purchase on BenchChem are specifically designed for in-vitro studies, which are conducted outside of living organisms. In-vitro studies, derived from the Latin term "in glass," involve experiments performed in controlled laboratory settings using cells or tissues. It is important to note that these products are not categorized as medicines or drugs, and they have not received approval from the FDA for the prevention, treatment, or cure of any medical condition, ailment, or disease. We must emphasize that any form of bodily introduction of these products into humans or animals is strictly prohibited by law. It is essential to adhere to these guidelines to ensure compliance with legal and ethical standards in research and experimentation.