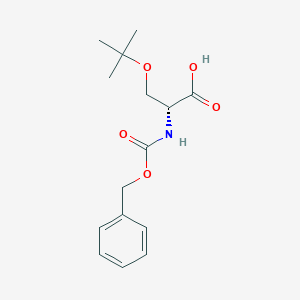
z-d-Ser(tbu)-oh
Overview
Description
Z-D-Ser(tBu)-OH (CAS 65806-90-8) is a protected amino acid derivative used in peptide synthesis and biochemical research. Its molecular formula is C₁₅H₂₁NO₅, with a molecular weight of 295.3 g/mol. The compound features:
- A benzyloxycarbonyl (Z) group protecting the α-amino group.
- A tert-butyl (tBu) group protecting the hydroxyl (-OH) side chain of serine.
- D-configuration at the α-carbon, distinguishing it from the naturally occurring L-serine.
This derivative is critical in solid-phase peptide synthesis (SPPS), where its protecting groups enhance stability during coupling reactions. The tBu group is resistant to acidic and basic conditions, making it compatible with Fmoc (9-fluorenylmethyloxycarbonyl) chemistry.
Preparation Methods
Fundamental Synthetic Routes
Stepwise Protection of Functional Groups
The synthesis of Z-D-Ser(tBu)-OH involves sequential protection of serine’s hydroxyl and amino groups:
Hydroxyl Protection (tert-Butylation):
Serine’s hydroxyl group is protected using tert-butyl chloride in the presence of a base (e.g., triethylamine or sodium bicarbonate). This reaction typically proceeds at 0–25°C for 4–6 hours, yielding O-tert-butyl-serine .
Amino Protection (Benzyloxycarbonylation):
The amino group is then protected with benzyloxycarbonyl chloride (Z-Cl) under basic conditions (pH 8–9). Sodium bicarbonate or N-methylmorpholine in a THF/water mixture facilitates this step, forming this compound .
Key Reaction Conditions:
Step | Reagents | Solvent | Temperature | Yield |
---|---|---|---|---|
Hydroxyl Protection | tert-Butyl chloride, TEA | DCM/THF | 0–25°C | 85–92% |
Amino Protection | Z-Cl, NaHCO₃ | THF/H₂O | 0–5°C | 78–88% |
Industrial-Scale Production
Optimized Large-Scale Protocols
Industrial synthesis employs automated peptide synthesizers and high-throughput purification. For example, a patent describing goserelin synthesis outlines:
-
Scale-Up tert-Butylation: Reactors with precise temperature control (‑20°C to 25°C) and tert-butyl chloride in 10:1 molar excess.
-
Continuous Flow Purification: Reverse-phase chromatography (C18 columns) with acetonitrile/water gradients achieves >98% purity .
Challenges and Solutions
-
Byproduct Formation: Residual tert-butyl groups are minimized using TFA/DCM (7.5:5:87.5 v/v) for selective cleavage .
-
Yield Optimization: Recrystallization from ethyl acetate/hexane mixtures improves crystallinity and purity .
Deprotection and Functionalization
Selective Deprotection Strategies
This compound serves as a building block in peptide chains, requiring controlled deprotection:
-
Z-Group Removal: Catalytic hydrogenation (H₂/Pd-C) in methanol or DMF .
-
tert-Butyl Cleavage: Trifluoroacetic acid (TFA) in dichloromethane (DCM) .
Comparative Deprotection Efficiency:
Method | Reagents | Time | Purity |
---|---|---|---|
Hydrogenation | H₂ (1 atm), Pd-C | 2 h | 95% |
Acidic Cleavage | TFA/DCM (1:9 v/v) | 1 h | 98% |
Advanced Methodological Innovations
Solid-Phase Peptide Synthesis (SPPS)
This compound is integrated into SPPS protocols using:
-
Coupling Agents: DIC/HOBt or EDCI/Oxyma in DMF, achieving 85–90% coupling efficiency .
-
Side Reaction Mitigation: Low-temperature (‑20°C) coupling minimizes diketopiperazine (DKP) formation .
Hybrid Liquid-Phase Techniques
A patent details fragment condensation using this compound in LH-RH derivative synthesis:
-
Azide Coupling: Isoamyl nitrite converts hydrazides to acyl azides, enabling segment condensation at ‑20°C .
-
Purification: Centrifugation and ether washing yield 87.5% pure intermediates .
Quality Control and Analytical Validation
Purity Assessment
-
HPLC: C18 columns with 0.1% TFA/acetonitrile gradients (retention time: 8–10 min) .
-
NMR: Characteristic peaks include tert-butyl (δ 1.2–1.4 ppm) and Z-group aromatics (δ 7.2–7.4 ppm).
Stability Protocols
-
Storage: ‑20°C under nitrogen prevents hydrolysis.
-
Handling: Anhydrous DMF ensures stability during peptide synthesis.
Comparative Analysis of Synthetic Approaches
Method | Advantages | Limitations |
---|---|---|
Stepwise Protection | High selectivity, scalable | Multi-step, moderate yields |
SPPS Integration | Automation, minimal side reactions | High reagent costs |
Industrial Production | Batch consistency, >98% purity | Specialized equipment |
Chemical Reactions Analysis
Types of Reactions
Z-D-Ser(tbu)-oh undergoes various chemical reactions, including:
Deprotection Reactions: The protective groups can be removed under specific conditions to yield free serine.
Coupling Reactions: It can be coupled with other amino acids or peptides to form longer peptide chains.
Common Reagents and Conditions
Deprotection: The tert-butyl group can be removed using trifluoroacetic acid, while the benzyloxycarbonyl group can be removed using hydrogenation in the presence of a palladium catalyst.
Coupling: Coupling reactions typically use reagents such as dicyclohexylcarbodiimide (DCC) or N,N’-diisopropylcarbodiimide (DIC) in the presence of a coupling agent like hydroxybenzotriazole (HOBt).
Major Products Formed
The major products formed from these reactions include free serine and various peptide chains, depending on the specific reactions and conditions used .
Scientific Research Applications
The compound Fmoc-D-Ser(tBu)-OH , also known as O-tert-butyl-N-(9H-fluoren-9-ylmethoxycarbonyl)-D-serine, is a derivative of D-serine that plays a significant role in various scientific research applications, particularly in the fields of biochemistry and medicinal chemistry. This article provides a detailed overview of its applications, supported by comprehensive data tables and case studies.
Chemical Properties and Structure
Fmoc-D-Ser(tBu)-OH has the molecular formula and a molecular weight of 385.44 g/mol. Its structure includes a fluorenylmethoxycarbonyl (Fmoc) protecting group, which is commonly used in peptide synthesis to protect the amino group, and a tert-butyl group that enhances the compound's stability and solubility.
Peptide Synthesis
Fmoc-D-Ser(tBu)-OH is primarily utilized in solid-phase peptide synthesis (SPPS). The Fmoc group allows for selective deprotection under mild conditions, facilitating the stepwise assembly of peptides. This method is essential for producing peptides with specific sequences for biological studies.
Case Study: Synthesis of Neuroactive Peptides
Research has demonstrated the successful synthesis of neuroactive peptides using Fmoc-D-Ser(tBu)-OH as a building block. These peptides have shown potential in modulating neurotransmitter systems, indicating their utility in neuropharmacology.
Drug Development
The compound has been investigated for its role in drug development, particularly as a scaffold for creating bioactive compounds. Its structural features allow for modifications that can enhance pharmacological properties.
Case Study: Anticancer Agents
Studies have explored derivatives of Fmoc-D-Ser(tBu)-OH that exhibit cytotoxic activity against cancer cell lines. The incorporation of this compound into drug design has led to promising candidates for further development in oncology.
Bioconjugation
Fmoc-D-Ser(tBu)-OH is also employed in bioconjugation strategies to attach biomolecules such as antibodies or enzymes to therapeutic agents. This enhances the specificity and efficacy of treatments.
Case Study: Targeted Drug Delivery
Research has shown that conjugating Fmoc-D-Ser(tBu)-OH derivatives to antibody fragments improves targeted delivery to tumor cells, significantly enhancing therapeutic outcomes in preclinical models.
Tissue Engineering
In tissue engineering, Fmoc-D-Ser(tBu)-OH is used to create hydrogels that mimic extracellular matrices. These hydrogels support cell growth and differentiation, making them valuable for regenerative medicine applications.
Case Study: Scaffold Development
Investigations into scaffolds made from Fmoc-D-Ser(tBu)-OH-based hydrogels have demonstrated their effectiveness in promoting the growth of neural stem cells, indicating potential applications in nerve repair.
Mechanism of Action
The mechanism of action of Z-D-Ser(tbu)-oh primarily involves its role as a protected amino acid in peptide synthesis. The protective groups prevent unwanted side reactions, allowing for the selective formation of peptide bonds. The benzyloxycarbonyl group protects the amino group, while the tert-butyl group protects the hydroxyl group. These protective groups can be selectively removed under specific conditions to yield the desired peptide product .
Comparison with Similar Compounds
Structural and Functional Differences
The table below compares Z-D-Ser(tBu)-OH with structurally related serine derivatives:
Compound Name | CAS Number | Molecular Formula | Molecular Weight (g/mol) | Protecting Groups | Configuration | Key Applications |
---|---|---|---|---|---|---|
This compound | 65806-90-8 | C₁₅H₂₁NO₅ | 295.3 | Z (amino), tBu (hydroxyl) | D | Peptide synthesis, SPPS |
Z-Ser(tBu)-OH (L-isomer) | 1676-75-1 | C₁₅H₂₁NO₅ | 295.3 | Z (amino), tBu (hydroxyl) | L | Synthesis of L-serine peptides |
Z-D-Ser-OH | 6081-61-4 | C₁₁H₁₃NO₅ | 239.2 | Z (amino), unprotected -OH | D | Short peptide sequences |
Z-D-Ser(tBu)-OMe | 93204-37-6 | C₁₆H₂₃NO₅ | 309.4 | Z (amino), tBu (hydroxyl), OMe (carboxyl) | D | Esterified intermediates |
Boc-D-Ser(tBu)-OH | 248921-66-6 | C₁₂H₂₃NO₅ | 261.2 | Boc (amino), tBu (hydroxyl) | D | Boc-based SPPS strategies |
Key Analysis
(a) Protecting Group Chemistry
- Z vs. Boc: The Z group (benzyloxycarbonyl) is removed via hydrogenolysis or strong acids (e.g., HBr/acetic acid), while the Boc (tert-butoxycarbonyl) group is cleaved under milder acidic conditions (e.g., trifluoroacetic acid). This makes Boc-D-Ser(tBu)-OH preferable for acid-sensitive sequences.
- tBu vs. Tos : Z-Ser(Tos)-OMe (CAS 1492-52-0) uses a tosyl (Tos) group for hydroxyl protection, which is more stable under acidic conditions but requires harsh reagents (e.g., Na/NH₃) for removal compared to tBu.
(b) Stereochemical Impact
- D vs. L Configuration : this compound is essential for synthesizing D-serine-containing peptides, which are increasingly studied for their resistance to enzymatic degradation and unique biological activities.
(c) Solubility and Reactivity
- The tBu group in this compound reduces polarity, enhancing solubility in organic solvents (e.g., DMF, dichloromethane) but limiting aqueous solubility. In contrast, Z-D-Ser-OH (unprotected hydroxyl) is more hydrophilic.
- Methyl ester (OMe) derivatives like Z-D-Ser(tBu)-OMe are less reactive at the carboxyl terminus, enabling selective coupling at the amino group.
Research Findings and Trends
- Stability Studies : this compound exhibits >95% stability under Fmoc deprotection conditions (20% piperidine/DMF), whereas Tos-protected analogs degrade under similar conditions.
- Cost and Availability : this compound is priced at $100/25g , while Z-Ser(Tos)-OMe costs $55/25g , reflecting differences in synthetic complexity.
- Emerging Uses : D-serine derivatives are being explored in neurological drug development due to their role in modulating NMDA receptors.
Biological Activity
Z-D-Ser(tBu)-OH, also known as Z-O-tert-butyl-D-serine, is a derivative of the amino acid D-serine. This compound plays a significant role in biochemical research and has implications in neurobiology due to its structural and functional properties. This article explores its biological activity, synthesis, and applications, supported by relevant data and case studies.
Chemical Structure and Properties
This compound is characterized by a tert-butyl group protecting the hydroxyl side chain and a benzyloxycarbonyl (Z) group protecting the amine group. The molecular formula is with a molecular weight of approximately 161.199 g/mol. These protective groups enhance the compound's stability and solubility compared to its parent amino acid, D-serine .
This compound functions primarily as a building block for peptides, allowing researchers to incorporate D-serine into various peptide sequences. D-serine itself acts as a co-agonist at the N-methyl-D-aspartate (NMDA) receptor, which is essential for synaptic plasticity and memory formation. This receptor's modulation is crucial in understanding neurological disorders such as schizophrenia and Alzheimer's disease .
Neurobiological Implications
Research indicates that D-serine levels are critical in several neurological processes. For instance, studies have shown that D-serine can influence neurotransmitter systems, particularly those involving glutamate receptors. This modulation can lead to new therapeutic strategies for managing conditions like schizophrenia and depression .
Synthesis of this compound
The synthesis of this compound typically involves:
- Protection of Functional Groups : The amine group is protected with a benzyloxycarbonyl (Z) group while the hydroxyl group is protected with a tert-butyl (tBu) group.
- Formation of Peptide Bonds : The methyl ester form can be used to activate the carboxyl group for peptide bond formation.
- Deprotection : Upon completion of peptide synthesis, the protective groups can be removed to yield the desired peptide containing D-serine .
Applications in Research
This compound has several applications in biochemical research:
- Peptide Synthesis : It serves as an essential building block for synthesizing peptides that target specific neurological pathways.
- Drug Development : Compounds incorporating D-serine may offer therapeutic benefits in treating neurodegenerative diseases.
- Receptor Interaction Studies : Research often focuses on how derivatives like this compound can influence receptor binding affinities and activities .
Comparative Analysis with Other Amino Acid Derivatives
To understand the uniqueness of this compound, it is helpful to compare it with other serine derivatives:
Compound Name | Structure Features | Unique Characteristics |
---|---|---|
Z-L-Ser(tbu)-OH | L-serine derivative with tert-butyl protection | Used in similar applications but differs in chirality |
Fmoc-L-Ser(tbu)-OH | L-serine derivative with fluorenylmethoxycarbonyl protection | More stable under basic conditions; used extensively in solid-phase peptide synthesis |
Z-O-tert-butyl-L-serine | L-serine derivative similar to this compound | Directly comparable but with L-stereochemistry |
The distinct D-stereochemistry of this compound significantly influences its biological activity compared to L-isomers.
Case Studies and Research Findings
Several studies have explored the biological activity of D-serine derivatives:
- D-serine in Schizophrenia Treatment : Clinical trials have indicated that increasing D-serine levels can ameliorate symptoms in patients with schizophrenia by enhancing NMDA receptor function .
- Role in Alzheimer's Disease : Research has suggested that D-serine may play a protective role against neurodegeneration observed in Alzheimer's disease models, indicating potential therapeutic avenues for compounds like this compound .
- Peptide Design for Neuroprotection : Investigations into peptides synthesized using this compound have shown promising results in modulating neuroprotective pathways, highlighting its utility in drug development .
Q & A
Basic Research Questions
Q. How is Z-D-Ser(tBu)-OH synthesized, and what are the critical parameters affecting yield and purity?
- Methodological Answer : Synthesis typically involves tert-butyl protection of the serine hydroxyl group followed by Z-carbobenzoxy protection of the amino group. Key parameters include reaction temperature (optimized between 0–25°C), solvent choice (e.g., dichloromethane or DMF for solubility), and stoichiometry of protecting reagents (e.g., di-tert-butyl dicarbonate for tBu). Purification via flash chromatography or recrystallization is critical, with yield and purity monitored by HPLC (≥95% purity threshold recommended) .
Q. What spectroscopic methods are recommended for characterizing this compound?
- Methodological Answer : Nuclear Magnetic Resonance (NMR) spectroscopy (¹H and ¹³C) is essential for confirming the tert-butyl (δ ~1.2 ppm for 9H singlet) and Z-protecting groups (aromatic protons δ ~7.3 ppm). Mass spectrometry (ESI-MS or MALDI-TOF) validates molecular weight ([M+H]+ expected ~310.3 g/mol). Discrepancies in NMR splitting patterns may indicate racemization, requiring chiral HPLC or polarimetry for resolution .
Q. How should researchers design stability studies for this compound under varying storage conditions?
- Methodological Answer : Conduct accelerated stability testing by storing the compound at –20°C, 4°C, and room temperature (25°C) with controlled humidity (e.g., 60% RH). Analyze aliquots at 0, 1, 3, and 6 months using HPLC to track degradation (e.g., hydrolysis of the tBu group). Include lyophilized vs. solution-state comparisons, as moisture accelerates decomposition .
Advanced Research Questions
Q. How does the tert-butyl protection in this compound influence its reactivity in solid-phase peptide synthesis (SPPS)?
- Methodological Answer : The bulky tert-butyl group reduces steric hindrance during amino acid coupling compared to bulkier alternatives (e.g., trityl), improving reaction efficiency. However, it may slow deprotection steps requiring strong acids (e.g., TFA). Optimize coupling reagents (e.g., HBTU or DIC/Oxyma) and monitor via Kaiser test to ensure complete reactions. Comparative studies with other serine derivatives (e.g., Z-Ser-OtBu vs. Fmoc-Ser(tBu)-OH) can clarify steric vs. electronic effects .
Q. What are the common side reactions during this compound coupling, and how can they be mitigated?
- Methodological Answer : Racemization (up to 5% in basic conditions) and incomplete coupling are key issues. Mitigation strategies include:
- Using low-basicity coupling agents (e.g., COMU instead of HATU).
- Adding anti-racemization additives (e.g., HOBt or Oxyma).
- Monitoring reaction progress via LC-MS.
Data contradictions in peptide yield vs. purity should be resolved by repeating reactions under inert atmospheres (N₂/Ar) to exclude oxidative side reactions .
Q. How can researchers resolve conflicting data on this compound’s solubility in non-polar solvents?
- Methodological Answer : Solubility discrepancies often arise from batch-to-batch variability in crystallinity. Pre-treat the compound via lyophilization or sonication in anhydrous solvents. Quantify solubility using UV-Vis spectroscopy (λ = 220 nm for Z-group absorption) and compare with computational predictions (e.g., Hansen solubility parameters). Contradictions may necessitate alternative solvent systems (e.g., THF/DMF mixtures) .
Q. What strategies optimize the integration of this compound into complex peptide sequences while preserving stereochemistry?
- Methodological Answer : Use iterative SPPS with orthogonal protecting groups (e.g., Fmoc for other residues). Monitor chiral integrity via circular dichroism (CD) after each coupling step. For high-risk sequences (e.g., β-sheet-prone regions), incorporate pseudo-proline dipeptides to reduce aggregation. Post-synthesis analysis via 2D NMR (e.g., NOESY) verifies correct folding and stereochemistry .
Q. Data Analysis and Contradiction Management
Q. How should researchers address inconsistencies in this compound’s reported melting points?
- Methodological Answer : Variations in melting points (e.g., 90–100°C) may stem from polymorphic forms or residual solvents. Characterize thermal behavior via differential scanning calorimetry (DSC) and thermogravimetric analysis (TGA). Cross-validate with X-ray crystallography to identify crystalline vs. amorphous phases. Re-crystallize from multiple solvents (e.g., ethyl acetate/hexane) to isolate pure polymorphs .
Q. What analytical workflows validate the absence of racemization in this compound-derived peptides?
- Methodological Answer : Combine Marfey’s analysis (post-hydrolysis derivatization with FDAA) with chiral HPLC. For large peptides, use enzymatic digestion (e.g., trypsin) followed by LC-MS/MS to isolate and analyze serine-containing fragments. Discrepancies between methods require re-synthesis under optimized conditions (e.g., lower temperature, shorter reaction times) .
Q. Experimental Design Frameworks
Q. How can the P-E/I-C-O framework structure studies on this compound’s role in peptide bioactivity?
- Methodological Answer :
- Population (P) : Target peptide sequences (e.g., antimicrobial peptides).
- Exposure/Intervention (E/I) : Incorporation of this compound vs. native serine.
- Comparison (C) : Bioactivity of peptides with/without tert-butyl protection.
- Outcome (O) : Measured via minimum inhibitory concentration (MIC) assays or cytotoxicity profiles.
Predefine "shell" tables to capture variables (e.g., MIC values, HPLC retention times) for systematic analysis .
Properties
IUPAC Name |
(2R)-3-[(2-methylpropan-2-yl)oxy]-2-(phenylmethoxycarbonylamino)propanoic acid | |
---|---|---|
Source | PubChem | |
URL | https://pubchem.ncbi.nlm.nih.gov | |
Description | Data deposited in or computed by PubChem | |
InChI |
InChI=1S/C15H21NO5/c1-15(2,3)21-10-12(13(17)18)16-14(19)20-9-11-7-5-4-6-8-11/h4-8,12H,9-10H2,1-3H3,(H,16,19)(H,17,18)/t12-/m1/s1 | |
Source | PubChem | |
URL | https://pubchem.ncbi.nlm.nih.gov | |
Description | Data deposited in or computed by PubChem | |
InChI Key |
TXDGEONUWGOCJG-GFCCVEGCSA-N | |
Source | PubChem | |
URL | https://pubchem.ncbi.nlm.nih.gov | |
Description | Data deposited in or computed by PubChem | |
Canonical SMILES |
CC(C)(C)OCC(C(=O)O)NC(=O)OCC1=CC=CC=C1 | |
Source | PubChem | |
URL | https://pubchem.ncbi.nlm.nih.gov | |
Description | Data deposited in or computed by PubChem | |
Isomeric SMILES |
CC(C)(C)OC[C@H](C(=O)O)NC(=O)OCC1=CC=CC=C1 | |
Source | PubChem | |
URL | https://pubchem.ncbi.nlm.nih.gov | |
Description | Data deposited in or computed by PubChem | |
Molecular Formula |
C15H21NO5 | |
Source | PubChem | |
URL | https://pubchem.ncbi.nlm.nih.gov | |
Description | Data deposited in or computed by PubChem | |
Molecular Weight |
295.33 g/mol | |
Source | PubChem | |
URL | https://pubchem.ncbi.nlm.nih.gov | |
Description | Data deposited in or computed by PubChem | |
Retrosynthesis Analysis
AI-Powered Synthesis Planning: Our tool employs the Template_relevance Pistachio, Template_relevance Bkms_metabolic, Template_relevance Pistachio_ringbreaker, Template_relevance Reaxys, Template_relevance Reaxys_biocatalysis model, leveraging a vast database of chemical reactions to predict feasible synthetic routes.
One-Step Synthesis Focus: Specifically designed for one-step synthesis, it provides concise and direct routes for your target compounds, streamlining the synthesis process.
Accurate Predictions: Utilizing the extensive PISTACHIO, BKMS_METABOLIC, PISTACHIO_RINGBREAKER, REAXYS, REAXYS_BIOCATALYSIS database, our tool offers high-accuracy predictions, reflecting the latest in chemical research and data.
Strategy Settings
Precursor scoring | Relevance Heuristic |
---|---|
Min. plausibility | 0.01 |
Model | Template_relevance |
Template Set | Pistachio/Bkms_metabolic/Pistachio_ringbreaker/Reaxys/Reaxys_biocatalysis |
Top-N result to add to graph | 6 |
Feasible Synthetic Routes
Disclaimer and Information on In-Vitro Research Products
Please be aware that all articles and product information presented on BenchChem are intended solely for informational purposes. The products available for purchase on BenchChem are specifically designed for in-vitro studies, which are conducted outside of living organisms. In-vitro studies, derived from the Latin term "in glass," involve experiments performed in controlled laboratory settings using cells or tissues. It is important to note that these products are not categorized as medicines or drugs, and they have not received approval from the FDA for the prevention, treatment, or cure of any medical condition, ailment, or disease. We must emphasize that any form of bodily introduction of these products into humans or animals is strictly prohibited by law. It is essential to adhere to these guidelines to ensure compliance with legal and ethical standards in research and experimentation.