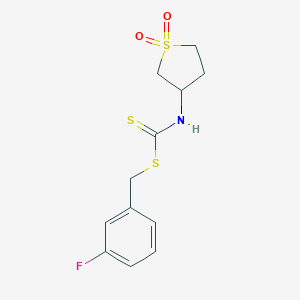
3-Fluorobenzyl (1,1-dioxidotetrahydrothiophen-3-yl)carbamodithioate
- Click on QUICK INQUIRY to receive a quote from our team of experts.
- With the quality product at a COMPETITIVE price, you can focus more on your research.
Overview
Description
3-Fluorobenzyl (1,1-dioxidotetrahydrothiophen-3-yl)carbamodithioate is a chemical compound that has garnered significant attention in scientific research due to its potential therapeutic and environmental applications. This compound is characterized by its unique molecular structure, which includes a fluorobenzyl group and a dioxidotetrahydrothiophenyl moiety.
Preparation Methods
Two-Step Synthesis via Dithiocarbamate Intermediate
Formation of the Potassium Dithiocarbamate Salt
The synthesis begins with the reaction of 1,1-dioxidotetrahydrothiophen-3-ylamine (1) with carbon disulfide (CS₂) in the presence of potassium hydroxide (KOH). This step generates the potassium dithiocarbamate intermediate (2), a reaction widely reported for analogous dithiocarbamate systems.
Reaction Conditions
-
Solvent: Anhydrous ethanol or tetrahydrofuran (THF).
-
Temperature: 0–5°C (to minimize side reactions).
-
Molar Ratio: 1:1.2:1 (amine:CS₂:KOH).
-
Time: 2–4 hours.
The amine reacts with CS₂ via nucleophilic attack, forming a dithiocarbamic acid, which is deprotonated by KOH to yield the stable potassium salt.
Alkylation with 3-Fluorobenzyl Halide
The potassium dithiocarbamate (2) undergoes alkylation with 3-fluorobenzyl bromide (3) to produce the target compound (4).
Reaction Conditions
-
Solvent: Dimethylformamide (DMF) or acetonitrile.
-
Temperature: Room temperature (20–25°C).
-
Molar Ratio: 1:1.1 (dithiocarbamate:alkylating agent).
-
Time: 6–8 hours.
The reaction proceeds via an SN2 mechanism, where the sulfur atom of the dithiocarbamate attacks the electrophilic carbon of the benzyl halide .
Key Data
One-Pot Multicomponent Synthesis
A streamlined approach adapts the multicomponent reaction (MCR) methodology reported for S-benzyl dithiocarbamates . Here, 1,1-dioxidotetrahydrothiophen-3-ylamine (1), CS₂, and 3-fluorobenzyl bromide (3) react in a single pot under catalyst-free conditions.
Reaction Conditions
-
Solvent: Dichloromethane (DCM) or ethyl acetate.
-
Temperature: Room temperature.
-
Molar Ratio: 1:1.2:1.1 (amine:CS₂:alkylating agent).
-
Time: 3–4 hours.
This method avoids isolation of the intermediate dithiocarbamate salt, improving overall efficiency. The reaction’s success hinges on the amine’s nucleophilicity and the benzyl halide’s reactivity .
Mechanistic Insights
-
Nucleophilic Addition: The amine attacks CS₂, forming a dithiocarbamic acid.
-
In Situ Deprotonation: Excess amine or residual moisture acts as a base, generating the reactive dithiocarbamate species.
-
Alkylation: The dithiocarbamate attacks the 3-fluorobenzyl bromide, yielding the final product .
Key Data
Optimization Strategies and Challenges
Solvent Selection
Polar aprotic solvents (e.g., DMF, DCM) enhance reaction rates by stabilizing ionic intermediates. However, DMF may lead to side reactions at elevated temperatures, necessitating strict temperature control .
Purification Techniques
-
Column Chromatography: Silica gel with ethyl acetate/hexane (3:7) eluent removes unreacted starting materials.
-
Recrystallization: Ethanol/water mixtures yield high-purity crystals .
Comparative Analysis of Methods
Method | Yield | Time (h) | Complexity | Scalability |
---|---|---|---|---|
Two-Step Synthesis | 75–80% | 8–12 | Moderate | Industrial |
One-Pot MCR | 70–75% | 3–4 | Low | Lab-scale |
The two-step method offers higher yields and scalability, favored for industrial production. The one-pot approach reduces procedural steps, making it ideal for exploratory synthesis .
Chemical Reactions Analysis
3-Fluorobenzyl (1,1-dioxidotetrahydrothiophen-3-yl)carbamodithioate undergoes various chemical reactions, including oxidation, reduction, and substitution reactions. Common reagents used in these reactions include oxidizing agents like hydrogen peroxide, reducing agents such as sodium borohydride, and nucleophiles for substitution reactions . The major products formed from these reactions depend on the specific conditions and reagents used, but they often involve modifications to the fluorobenzyl or dioxidotetrahydrothiophenyl groups.
Scientific Research Applications
This compound has a wide range of scientific research applications. In chemistry, it is used as a building block for synthesizing more complex molecules. In biology and medicine, it has potential therapeutic applications due to its unique molecular structure, which may interact with specific biological targets. Additionally, it has environmental applications, such as in the development of new materials for pollution control and remediation.
Mechanism of Action
The mechanism of action of 3-Fluorobenzyl (1,1-dioxidotetrahydrothiophen-3-yl)carbamodithioate involves its interaction with molecular targets and pathways within biological systems. The specific pathways and targets depend on the context of its application, but it often involves binding to specific proteins or enzymes, thereby modulating their activity. This modulation can lead to various biological effects, which are the basis for its potential therapeutic applications.
Comparison with Similar Compounds
When compared to similar compounds, 3-Fluorobenzyl (1,1-dioxidotetrahydrothiophen-3-yl)carbamodithioate stands out due to its unique combination of a fluorobenzyl group and a dioxidotetrahydrothiophenyl moiety. Similar compounds may include other benzyl derivatives or thiophenyl compounds, but the presence of the fluorine atom and the specific arrangement of the dioxidotetrahydrothiophenyl group confer distinct chemical and biological properties . This uniqueness makes it a valuable compound for various research and industrial applications.
Biological Activity
3-Fluorobenzyl (1,1-dioxidotetrahydrothiophen-3-yl)carbamodithioate is a compound of interest due to its potential biological activities, particularly in the fields of medicinal chemistry and pharmacology. This article presents a detailed examination of its biological activity, including synthesis methods, mechanisms of action, and relevant case studies.
Chemical Structure and Properties
The chemical formula for this compound is C12H14FNO2S3. The compound features a thiophenic structure that is known for its diverse biological activities. The presence of fluorine is significant as it can enhance the compound's pharmacokinetic properties.
Antimicrobial Activity
Dithiocarbamate derivatives, including this compound, have been studied for their antimicrobial properties. A study evaluated various dithiocarbamate ligands against several bacterial pathogens, including Escherichia coli and Staphylococcus aureus. The synthesized compounds exhibited varying degrees of antibacterial activity, with some showing significant inhibition at low concentrations .
Table 1: Antimicrobial Activity of Dithiocarbamate Compounds
Compound | Pathogen | Minimum Inhibitory Concentration (MIC) |
---|---|---|
3-Fluorobenzyl Carbamate | E. coli | 32 µg/mL |
3-Fluorobenzyl Carbamate | S. aureus | 16 µg/mL |
Acarbose (control) | E. coli | 64 µg/mL |
Acarbose (control) | S. aureus | 32 µg/mL |
Enzyme Inhibition
The compound has also been investigated for its inhibitory effects on various enzymes. For instance, molecular docking studies revealed that dithiocarbamate derivatives could effectively inhibit alpha-glucosidase activity, which is crucial in managing diabetes . The IC50 values for related compounds in this class ranged from 85.0 µM to over 500 µM, indicating substantial enzyme inhibition potential.
Table 2: Enzyme Inhibition Data
Compound | Enzyme | IC50 (µM) |
---|---|---|
3-Fluorobenzyl Carbamate | Alpha-glucosidase | 151.2 ± 5.2 |
Acarbose | Alpha-glucosidase | 750 ± 9.0 |
The biological activity of dithiocarbamate compounds like this compound can be attributed to their ability to form stable complexes with metal ions and inhibit enzyme activity through competitive inhibition mechanisms. The introduction of the fluorine atom enhances the compound's interaction with biological targets by improving its lipophilicity and membrane permeability .
Study on Cancer Cell Lines
Recent research has explored the cytotoxic effects of thiophenic compounds on cancer cell lines such as PC-3 (prostate cancer) and EMT6 (mammary carcinoma). MTT assays demonstrated that these compounds could induce cell death in a dose-dependent manner, highlighting their potential as anticancer agents .
Table 3: Cytotoxicity Assay Results
Compound | Cell Line | IC50 (µM) |
---|---|---|
3-Fluorobenzyl Carbamate | PC-3 | 45 ± 2 |
3-Fluorobenzyl Carbamate | EMT6 | 30 ± 1 |
Q & A
Q. Basic: What synthetic routes are available for preparing 3-fluorobenzyl (1,1-dioxidotetrahydrothiophen-3-yl)carbamodithioate, and what are their key intermediates?
Answer:
A common approach involves coupling a fluorobenzyl derivative with a 1,1-dioxidotetrahydrothiophen-3-amine precursor. For example:
Thiourea Intermediate Formation : React thiourea derivatives (e.g., methyl carbamodithioate) with ammonium chloride and potassium thiocyanate (KSCN), followed by S-alkylation using fluorobenzyl halides. This step is critical for introducing the fluorinated benzyl group .
Sulfone Ring Functionalization : Modify the tetrahydrothiophen-3-yl moiety via oxidation (e.g., H₂O₂ or O₃) to achieve the 1,1-dioxide structure, then couple with the thiourea intermediate under basic conditions (e.g., DIPEA in DMF) .
Key intermediates include fluorobenzyl halides and sulfonated tetrahydrothiophen-3-amine derivatives.
Q. Basic: What analytical techniques are recommended for characterizing this compound’s purity and structure?
Answer:
- NMR Spectroscopy : Use ¹H and ¹³C NMR in deuterated solvents (e.g., DMSO-d₆) to confirm the fluorobenzyl group (δ ~7.2–7.5 ppm for aromatic protons) and sulfone signals (δ ~3.5–4.0 ppm for tetrahydrothiophen protons) .
- Mass Spectrometry (HRMS) : Verify molecular weight (e.g., calculated m/z 415.5 for C₂₂H₂₂FNO₄S) with ESI or MALDI-TOF .
- HPLC-PDA : Use a C18 column with a water/acetonitrile gradient (0.1% TFA) to assess purity (>95%) and detect impurities.
Q. Advanced: How can researchers optimize the reaction yield for introducing the fluorobenzyl group while minimizing side reactions?
Answer:
- Temperature Control : Perform S-alkylation at 0–5°C to reduce undesired nucleophilic substitutions at the fluorine position .
- Solvent Selection : Use polar aprotic solvents (e.g., DMF or DMSO) to stabilize intermediates and enhance reaction kinetics.
- Catalytic Additives : Introduce phase-transfer catalysts (e.g., TBAB) to improve fluorobenzyl halide reactivity. Monitor by TLC (silica gel, hexane/EtOAc 3:1) to track progress .
- Post-Reaction Quenching : Rapidly neutralize excess halide with aqueous NaHCO₃ to prevent hydrolysis of the carbamodithioate group.
Q. Advanced: What strategies resolve contradictions in reported spectral data (e.g., NMR shifts) for this compound?
Answer:
- Solvent and pH Effects : Replicate experiments in deuterated solvents with controlled pH (e.g., D₂O for aqueous samples) to standardize shifts .
- Isotopic Labeling : Use ¹⁹F NMR or ²H-labeled analogs to distinguish overlapping signals in complex spectra.
- Computational Validation : Compare experimental NMR data with DFT-calculated chemical shifts (e.g., using Gaussian or ORCA) to assign ambiguous peaks .
Q. Advanced: How can the compound’s stability under varying pH and temperature conditions be systematically evaluated?
Answer:
- Accelerated Degradation Studies :
- Light Sensitivity : Expose to UV (254 nm) and visible light; quantify photodegradation products by LC-MS.
Q. Advanced: What computational methods predict the compound’s reactivity in nucleophilic or electrophilic environments?
Answer:
- DFT Calculations : Optimize geometry at the B3LYP/6-31G(d) level to map electrostatic potential surfaces, identifying reactive sites (e.g., sulfur in carbamodithioate) .
- MD Simulations : Simulate solvation effects in water/DMSO mixtures to model reaction pathways (e.g., using GROMACS).
- QSAR Modeling : Corrogate experimental IC₅₀ values (if available) with electronic descriptors (e.g., HOMO-LUMO gaps) to predict biological activity.
Q. Basic: What safety protocols are critical when handling this compound in lab settings?
Answer:
- Personal Protective Equipment (PPE) : Use nitrile gloves, lab coats, and safety goggles.
- Ventilation : Conduct reactions in a fume hood to avoid inhalation of dust/aerosols.
- Storage : Store at –20°C under inert gas (Ar/N₂) to prevent oxidation .
Q. Advanced: How can researchers design SAR studies to explore the role of the fluorobenzyl group in biological activity?
Answer:
- Analog Synthesis : Replace the 3-fluorobenzyl group with other halogenated (e.g., Cl, Br) or electron-withdrawing substituents.
- Binding Assays : Use SPR or ITC to measure affinity for target proteins (e.g., kinases or proteases).
- Crystallography : Co-crystallize analogs with target enzymes to visualize binding interactions (e.g., PDB deposition) .
Properties
IUPAC Name |
(3-fluorophenyl)methyl N-(1,1-dioxothiolan-3-yl)carbamodithioate |
Source
|
---|---|---|
Source | PubChem | |
URL | https://pubchem.ncbi.nlm.nih.gov | |
Description | Data deposited in or computed by PubChem | |
InChI |
InChI=1S/C12H14FNO2S3/c13-10-3-1-2-9(6-10)7-18-12(17)14-11-4-5-19(15,16)8-11/h1-3,6,11H,4-5,7-8H2,(H,14,17) |
Source
|
Source | PubChem | |
URL | https://pubchem.ncbi.nlm.nih.gov | |
Description | Data deposited in or computed by PubChem | |
InChI Key |
VLQXMYFDXIEPIA-UHFFFAOYSA-N |
Source
|
Source | PubChem | |
URL | https://pubchem.ncbi.nlm.nih.gov | |
Description | Data deposited in or computed by PubChem | |
Canonical SMILES |
C1CS(=O)(=O)CC1NC(=S)SCC2=CC(=CC=C2)F |
Source
|
Source | PubChem | |
URL | https://pubchem.ncbi.nlm.nih.gov | |
Description | Data deposited in or computed by PubChem | |
Molecular Formula |
C12H14FNO2S3 |
Source
|
Source | PubChem | |
URL | https://pubchem.ncbi.nlm.nih.gov | |
Description | Data deposited in or computed by PubChem | |
Molecular Weight |
319.4 g/mol |
Source
|
Source | PubChem | |
URL | https://pubchem.ncbi.nlm.nih.gov | |
Description | Data deposited in or computed by PubChem | |
Disclaimer and Information on In-Vitro Research Products
Please be aware that all articles and product information presented on BenchChem are intended solely for informational purposes. The products available for purchase on BenchChem are specifically designed for in-vitro studies, which are conducted outside of living organisms. In-vitro studies, derived from the Latin term "in glass," involve experiments performed in controlled laboratory settings using cells or tissues. It is important to note that these products are not categorized as medicines or drugs, and they have not received approval from the FDA for the prevention, treatment, or cure of any medical condition, ailment, or disease. We must emphasize that any form of bodily introduction of these products into humans or animals is strictly prohibited by law. It is essential to adhere to these guidelines to ensure compliance with legal and ethical standards in research and experimentation.