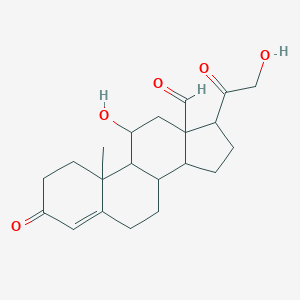
Aldosterone
Overview
Description
Aldosterone is a steroid hormone secreted by the adrenal glands. It serves as the principal regulator of the salt and water balance of the body and is categorized as a mineralocorticoid . It also has a small effect on the metabolism of fats, carbohydrates, and proteins . Aldosterone helps regulate blood pressure by managing the levels of sodium and potassium in your blood .
Synthesis Analysis
Aldosterone is synthesized from corticosterone, a steroid derived from cholesterol. The renin-angiotensin system regulates aldosterone production in the adrenal cortex’s glomerulosa zone. Renin is secreted from the kidneys in response to variations in blood pressure and volume and plasma levels of sodium and potassium . The synthesis of aldosterone is primarily stimulated by the activation of intracellular calcium signaling in the ZG that can be mediated either by angiotensin II from the renin-angiotensin system or by extracellular potassium levels .
Molecular Structure Analysis
The molecular structure of Aldosterone is available as a 2D Mol file .
Chemical Reactions Analysis
Two reactions, derivatization by Girard’s reagents and 1,1-carbonyldiimidazole (CDI), have been explored for improving IM separation of steroid hormone isomers. These reactions are fast (≤30 min), simple (requiring only basic lab equipment/expertise), and low-cost .
Physical And Chemical Properties Analysis
Aldosterone has a density of 1.3±0.1 g/cm3, a boiling point of 568.1±50.0 °C at 760 mmHg, and a vapour pressure of 0.0±3.5 mmHg at 25°C. Its enthalpy of vaporization is 97.9±6.0 kJ/mol and its flash point is 311.4±26.6 °C. It has an index of refraction of 1.586, a molar refractivity of 94.3±0.4 cm3, and a molar volume of 281.3±5.0 cm3 .
Scientific Research Applications
Cardiovascular Disease and Aldosterone
Aldosterone plays a crucial role in the pathophysiology of cardiovascular and renal target organ damage. Elevated aldosterone levels, despite body sodium expansion, have been linked to cardiac and vascular inflammation and fibrosis, leading to remodeling and disease. Novel findings indicate that disruption of circadian clock genes may increase aldosterone secretion and hypertension. Additionally, the interaction of mineralocorticoid and angiotensin AT1 receptors contributes to target organ damage (Carey, 2010).
Aldosterone in Pediatric Studies
In pediatric clinical research, the reliability of pharmacodynamic measures is tied to bioanalytical data quality. A comprehensive bioanalytical quality monitoring system was developed for the bioanalysis of aldosterone by immunoassay, demonstrating the critical role of aldosterone analysis in pediatric studies (Makowski et al., 2020).
Aldosterone's Role in Cardiovascular Physiology and Pathophysiology
Aldosterone's interaction with the mineralocorticoid receptor (MR) activates genomic and nongenomic pathways, crucially regulating cardiovascular system homeostasis. High levels of aldosterone can significantly alter the physiology of cells like fibroblasts, cardiomyocytes, and vascular cells, laying the groundwork for cardiovascular disorders like heart failure (Cannavo et al., 2018).
Aldosterone and Myocardial Pathology
Aldosterone exerts significant extra-renal effects, particularly on the heart, influencing cell function and growth. High aldosterone levels have been associated with various cardiovascular disorders, emphasizing the need to understand its role in myocardial pathology (Cannavo et al., 2019).
Effects on the Kidney and Cardiovascular System
Aldosterone impacts renal inflammatory and fibrotic processes, podocyte injury, and mesangial cell proliferation. In the cardiovascular system, it has specific hypertrophic and fibrotic effects and can alter endothelial function. These effects necessitate concomitant pathophysiological conditions such as high salt intake or increased oxidative stress (Briet & Schiffrin, 2010).
Aldosterone in Chronic Heart Failure
Elevated aldosterone levels in congestive heart failure patients suggest its significant role in cardiovascular pathology. Aldosterone receptor antagonists are increasingly used in heart failure management, with evidence supporting their benefits in reducing mortality and hospitalizations (Nappi & Sieg, 2011).
Regulation of Aldosterone Synthesis and Secretion
Understanding the regulation of aldosterone biosynthesis and secretion is vital for addressing hypertension, primary aldosteronism, congestive heart failure, and other cardiovascular disorders. The review discusses signaling pathways involved in aldosterone production, emphasizing the importance of the renin-angiotensin II system, serum potassium levels, and adrenocorticotrophic hormone (Bollag, 2014).
Aldosterone and End-Organ Damage
Aldosterone induces oxidative stress, endothelial dysfunction, inflammation, and fibrosis in the heart and kidneys. The progression from inflammation to fibrosis and remodeling due to aldosterone is a critical area of study for developing new strategies in cardiovascular and renal disease management (Brown, 2005).
Future Directions
Significant progress has been made in the measurement of aldosterone. In Japan, aldosterone can now be measured by the sandwich chemi-luminescent enzyme immunoassay (CLEIA) method . There is a major renaissance in interest concerning the role of aldosterone excess in human hypertension that has arisen in part from the gradual realization that primary aldosteronism is much more common than previously thought .
properties
IUPAC Name |
(8S,9S,10R,11S,13R,14S,17S)-11-hydroxy-17-(2-hydroxyacetyl)-10-methyl-3-oxo-1,2,6,7,8,9,11,12,14,15,16,17-dodecahydrocyclopenta[a]phenanthrene-13-carbaldehyde | |
---|---|---|
Source | PubChem | |
URL | https://pubchem.ncbi.nlm.nih.gov | |
Description | Data deposited in or computed by PubChem | |
InChI |
InChI=1S/C21H28O5/c1-20-7-6-13(24)8-12(20)2-3-14-15-4-5-16(18(26)10-22)21(15,11-23)9-17(25)19(14)20/h8,11,14-17,19,22,25H,2-7,9-10H2,1H3/t14-,15-,16+,17-,19+,20-,21+/m0/s1 | |
Source | PubChem | |
URL | https://pubchem.ncbi.nlm.nih.gov | |
Description | Data deposited in or computed by PubChem | |
InChI Key |
PQSUYGKTWSAVDQ-ZVIOFETBSA-N | |
Source | PubChem | |
URL | https://pubchem.ncbi.nlm.nih.gov | |
Description | Data deposited in or computed by PubChem | |
Canonical SMILES |
CC12CCC(=O)C=C1CCC3C2C(CC4(C3CCC4C(=O)CO)C=O)O | |
Source | PubChem | |
URL | https://pubchem.ncbi.nlm.nih.gov | |
Description | Data deposited in or computed by PubChem | |
Isomeric SMILES |
C[C@]12CCC(=O)C=C1CC[C@@H]3[C@@H]2[C@H](C[C@]4([C@H]3CC[C@@H]4C(=O)CO)C=O)O | |
Source | PubChem | |
URL | https://pubchem.ncbi.nlm.nih.gov | |
Description | Data deposited in or computed by PubChem | |
Molecular Formula |
C21H28O5 | |
Source | PubChem | |
URL | https://pubchem.ncbi.nlm.nih.gov | |
Description | Data deposited in or computed by PubChem | |
DSSTOX Substance ID |
DTXSID7022419 | |
Record name | Aldosterone | |
Source | EPA DSSTox | |
URL | https://comptox.epa.gov/dashboard/DTXSID7022419 | |
Description | DSSTox provides a high quality public chemistry resource for supporting improved predictive toxicology. | |
Molecular Weight |
360.4 g/mol | |
Source | PubChem | |
URL | https://pubchem.ncbi.nlm.nih.gov | |
Description | Data deposited in or computed by PubChem | |
Physical Description |
Solid | |
Record name | Aldosterone | |
Source | Human Metabolome Database (HMDB) | |
URL | http://www.hmdb.ca/metabolites/HMDB0000037 | |
Description | The Human Metabolome Database (HMDB) is a freely available electronic database containing detailed information about small molecule metabolites found in the human body. | |
Explanation | HMDB is offered to the public as a freely available resource. Use and re-distribution of the data, in whole or in part, for commercial purposes requires explicit permission of the authors and explicit acknowledgment of the source material (HMDB) and the original publication (see the HMDB citing page). We ask that users who download significant portions of the database cite the HMDB paper in any resulting publications. | |
Solubility |
0.0512 mg/mL at 37 °C | |
Record name | Aldosterone | |
Source | DrugBank | |
URL | https://www.drugbank.ca/drugs/DB04630 | |
Description | The DrugBank database is a unique bioinformatics and cheminformatics resource that combines detailed drug (i.e. chemical, pharmacological and pharmaceutical) data with comprehensive drug target (i.e. sequence, structure, and pathway) information. | |
Explanation | Creative Common's Attribution-NonCommercial 4.0 International License (http://creativecommons.org/licenses/by-nc/4.0/legalcode) | |
Record name | Aldosterone | |
Source | Human Metabolome Database (HMDB) | |
URL | http://www.hmdb.ca/metabolites/HMDB0000037 | |
Description | The Human Metabolome Database (HMDB) is a freely available electronic database containing detailed information about small molecule metabolites found in the human body. | |
Explanation | HMDB is offered to the public as a freely available resource. Use and re-distribution of the data, in whole or in part, for commercial purposes requires explicit permission of the authors and explicit acknowledgment of the source material (HMDB) and the original publication (see the HMDB citing page). We ask that users who download significant portions of the database cite the HMDB paper in any resulting publications. | |
Product Name |
Aldosterone | |
CAS RN |
52-39-1 | |
Record name | (+)-Aldosterone | |
Source | CAS Common Chemistry | |
URL | https://commonchemistry.cas.org/detail?cas_rn=52-39-1 | |
Description | CAS Common Chemistry is an open community resource for accessing chemical information. Nearly 500,000 chemical substances from CAS REGISTRY cover areas of community interest, including common and frequently regulated chemicals, and those relevant to high school and undergraduate chemistry classes. This chemical information, curated by our expert scientists, is provided in alignment with our mission as a division of the American Chemical Society. | |
Explanation | The data from CAS Common Chemistry is provided under a CC-BY-NC 4.0 license, unless otherwise stated. | |
Record name | Aldosterone hemiacetal | |
Source | ChemIDplus | |
URL | https://pubchem.ncbi.nlm.nih.gov/substance/?source=chemidplus&sourceid=0000052391 | |
Description | ChemIDplus is a free, web search system that provides access to the structure and nomenclature authority files used for the identification of chemical substances cited in National Library of Medicine (NLM) databases, including the TOXNET system. | |
Record name | Aldosterone | |
Source | DrugBank | |
URL | https://www.drugbank.ca/drugs/DB04630 | |
Description | The DrugBank database is a unique bioinformatics and cheminformatics resource that combines detailed drug (i.e. chemical, pharmacological and pharmaceutical) data with comprehensive drug target (i.e. sequence, structure, and pathway) information. | |
Explanation | Creative Common's Attribution-NonCommercial 4.0 International License (http://creativecommons.org/licenses/by-nc/4.0/legalcode) | |
Record name | ALDOSTERONE | |
Source | DTP/NCI | |
URL | https://dtp.cancer.gov/dtpstandard/servlet/dwindex?searchtype=NSC&outputformat=html&searchlist=73856 | |
Description | The NCI Development Therapeutics Program (DTP) provides services and resources to the academic and private-sector research communities worldwide to facilitate the discovery and development of new cancer therapeutic agents. | |
Explanation | Unless otherwise indicated, all text within NCI products is free of copyright and may be reused without our permission. Credit the National Cancer Institute as the source. | |
Record name | Pregn-4-en-18-al, 11,21-dihydroxy-3,20-dioxo-, (11.beta.)- | |
Source | EPA Chemicals under the TSCA | |
URL | https://www.epa.gov/chemicals-under-tsca | |
Description | EPA Chemicals under the Toxic Substances Control Act (TSCA) collection contains information on chemicals and their regulations under TSCA, including non-confidential content from the TSCA Chemical Substance Inventory and Chemical Data Reporting. | |
Record name | Aldosterone | |
Source | EPA DSSTox | |
URL | https://comptox.epa.gov/dashboard/DTXSID7022419 | |
Description | DSSTox provides a high quality public chemistry resource for supporting improved predictive toxicology. | |
Record name | Aldosterone | |
Source | European Chemicals Agency (ECHA) | |
URL | https://echa.europa.eu/substance-information/-/substanceinfo/100.000.128 | |
Description | The European Chemicals Agency (ECHA) is an agency of the European Union which is the driving force among regulatory authorities in implementing the EU's groundbreaking chemicals legislation for the benefit of human health and the environment as well as for innovation and competitiveness. | |
Explanation | Use of the information, documents and data from the ECHA website is subject to the terms and conditions of this Legal Notice, and subject to other binding limitations provided for under applicable law, the information, documents and data made available on the ECHA website may be reproduced, distributed and/or used, totally or in part, for non-commercial purposes provided that ECHA is acknowledged as the source: "Source: European Chemicals Agency, http://echa.europa.eu/". Such acknowledgement must be included in each copy of the material. ECHA permits and encourages organisations and individuals to create links to the ECHA website under the following cumulative conditions: Links can only be made to webpages that provide a link to the Legal Notice page. | |
Record name | ALDOSTERONE | |
Source | FDA Global Substance Registration System (GSRS) | |
URL | https://gsrs.ncats.nih.gov/ginas/app/beta/substances/4964P6T9RB | |
Description | The FDA Global Substance Registration System (GSRS) enables the efficient and accurate exchange of information on what substances are in regulated products. Instead of relying on names, which vary across regulatory domains, countries, and regions, the GSRS knowledge base makes it possible for substances to be defined by standardized, scientific descriptions. | |
Explanation | Unless otherwise noted, the contents of the FDA website (www.fda.gov), both text and graphics, are not copyrighted. They are in the public domain and may be republished, reprinted and otherwise used freely by anyone without the need to obtain permission from FDA. Credit to the U.S. Food and Drug Administration as the source is appreciated but not required. | |
Record name | Aldosterone | |
Source | Human Metabolome Database (HMDB) | |
URL | http://www.hmdb.ca/metabolites/HMDB0000037 | |
Description | The Human Metabolome Database (HMDB) is a freely available electronic database containing detailed information about small molecule metabolites found in the human body. | |
Explanation | HMDB is offered to the public as a freely available resource. Use and re-distribution of the data, in whole or in part, for commercial purposes requires explicit permission of the authors and explicit acknowledgment of the source material (HMDB) and the original publication (see the HMDB citing page). We ask that users who download significant portions of the database cite the HMDB paper in any resulting publications. | |
Melting Point |
166.5 °C | |
Record name | Aldosterone | |
Source | DrugBank | |
URL | https://www.drugbank.ca/drugs/DB04630 | |
Description | The DrugBank database is a unique bioinformatics and cheminformatics resource that combines detailed drug (i.e. chemical, pharmacological and pharmaceutical) data with comprehensive drug target (i.e. sequence, structure, and pathway) information. | |
Explanation | Creative Common's Attribution-NonCommercial 4.0 International License (http://creativecommons.org/licenses/by-nc/4.0/legalcode) | |
Record name | Aldosterone | |
Source | Human Metabolome Database (HMDB) | |
URL | http://www.hmdb.ca/metabolites/HMDB0000037 | |
Description | The Human Metabolome Database (HMDB) is a freely available electronic database containing detailed information about small molecule metabolites found in the human body. | |
Explanation | HMDB is offered to the public as a freely available resource. Use and re-distribution of the data, in whole or in part, for commercial purposes requires explicit permission of the authors and explicit acknowledgment of the source material (HMDB) and the original publication (see the HMDB citing page). We ask that users who download significant portions of the database cite the HMDB paper in any resulting publications. | |
Retrosynthesis Analysis
AI-Powered Synthesis Planning: Our tool employs the Template_relevance Pistachio, Template_relevance Bkms_metabolic, Template_relevance Pistachio_ringbreaker, Template_relevance Reaxys, Template_relevance Reaxys_biocatalysis model, leveraging a vast database of chemical reactions to predict feasible synthetic routes.
One-Step Synthesis Focus: Specifically designed for one-step synthesis, it provides concise and direct routes for your target compounds, streamlining the synthesis process.
Accurate Predictions: Utilizing the extensive PISTACHIO, BKMS_METABOLIC, PISTACHIO_RINGBREAKER, REAXYS, REAXYS_BIOCATALYSIS database, our tool offers high-accuracy predictions, reflecting the latest in chemical research and data.
Strategy Settings
Precursor scoring | Relevance Heuristic |
---|---|
Min. plausibility | 0.01 |
Model | Template_relevance |
Template Set | Pistachio/Bkms_metabolic/Pistachio_ringbreaker/Reaxys/Reaxys_biocatalysis |
Top-N result to add to graph | 6 |
Feasible Synthetic Routes
Citations
Disclaimer and Information on In-Vitro Research Products
Please be aware that all articles and product information presented on BenchChem are intended solely for informational purposes. The products available for purchase on BenchChem are specifically designed for in-vitro studies, which are conducted outside of living organisms. In-vitro studies, derived from the Latin term "in glass," involve experiments performed in controlled laboratory settings using cells or tissues. It is important to note that these products are not categorized as medicines or drugs, and they have not received approval from the FDA for the prevention, treatment, or cure of any medical condition, ailment, or disease. We must emphasize that any form of bodily introduction of these products into humans or animals is strictly prohibited by law. It is essential to adhere to these guidelines to ensure compliance with legal and ethical standards in research and experimentation.