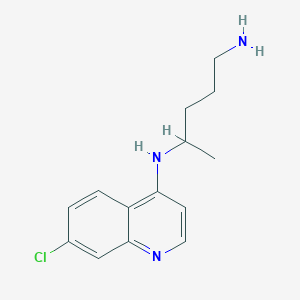
Didesethyl Chloroquine
Overview
Description
Didesethyl Chloroquine (DDCQ), also known as bisdesethylchloroquine, is a major metabolite of the antimalarial drug chloroquine (CQ). Its molecular formula is C₁₄H₁₈ClN₃, with a molecular weight of 263.77 g/mol and a CAS registry number of 4298-14-0 . Structurally, DDCQ is formed by the removal of two ethyl groups from chloroquine, distinguishing it from intermediate metabolites like desethyl chloroquine.
DDCQ has garnered attention in pharmacological research, particularly in studies related to COVID-19 antiviral activity and cardiotoxicity. Evidence indicates that DDCQ acts as a potent myocardial depressant, affecting cardiac sodium currents and mitochondrial function in preclinical models . Its physicochemical properties, such as lower rotatable bond count compared to chloroquine, are associated with improved oral bioavailability, making it a candidate for further therapeutic exploration .
Preparation Methods
Synthetic Routes and Reaction Conditions: Bisdesethylchloroquine is typically synthesized through the metabolic pathway of chloroquine and hydroxychloroquine. The primary synthetic route involves the dealkylation of these parent compounds via cytochrome P450 enzymes. This process results in the removal of ethyl groups, leading to the formation of bisdesethylchloroquine .
Industrial Production Methods: Industrial production of bisdesethylchloroquine is not commonly practiced as it is primarily a metabolite rather than a directly synthesized compound. its production can be achieved through controlled enzymatic reactions using cytochrome P450 enzymes in a laboratory setting .
Chemical Reactions Analysis
Types of Reactions: Bisdesethylchloroquine undergoes various chemical reactions, including:
Reduction: This reaction involves the removal of oxygen or the addition of hydrogen, typically using reducing agents.
Substitution: This reaction involves the replacement of one functional group with another, often using nucleophiles or electrophiles
Common Reagents and Conditions:
Oxidation: Common oxidizing agents include hydrogen peroxide and potassium permanganate.
Reduction: Common reducing agents include sodium borohydride and lithium aluminum hydride.
Substitution: Common reagents include halogens and alkylating agents
Major Products Formed: The major products formed from these reactions depend on the specific conditions and reagents used. For example, oxidation may yield hydroxylated derivatives, while reduction may produce deoxygenated compounds .
Scientific Research Applications
Scientific Research Applications
Didesethyl Chloroquine has several notable research applications across different domains:
Chemistry
- Reference Standard : Utilized as a reference standard in analytical chemistry for the identification and quantification of chloroquine derivatives.
- Synthesis Studies : Important in synthetic chemistry for understanding the modification of chloroquine derivatives.
Biology
- Molecular Docking Studies : Employed in studies to analyze interactions with biological targets, particularly in relation to viral infections such as chikungunya virus. Research indicates that this compound can bind effectively to viral proteins, suggesting potential therapeutic strategies against viral diseases .
Medicine
- Antiviral Potential : Investigated for its efficacy against various viruses, including chikungunya and SARS-CoV-2. Preliminary studies indicate that it may inhibit viral replication through mechanisms similar to those of chloroquine .
- Autoimmune Disorders : Like chloroquine, it may have applications in treating autoimmune conditions due to its immunomodulatory effects.
Industry
- Pharmaceutical Development : Used in the development of new chemical entities aimed at treating malaria and other diseases, enhancing drug discovery efforts .
Case Studies and Clinical Trials
Several studies have highlighted the potential applications of this compound:
Safety Profile and Considerations
While this compound shows promise in various applications, safety remains a concern. Reports indicate potential serious side effects, including cardiac issues when used at higher doses than recommended . Ongoing research aims to establish comprehensive safety profiles through well-designed clinical trials.
Mechanism of Action
Bisdesethylchloroquine exerts its effects through several mechanisms:
Lysosomal Activity: It interferes with lysosomal activity and autophagy, leading to the accumulation of undigested cellular debris.
Membrane Stability: It interacts with cellular membranes, altering their stability and permeability.
Signaling Pathways: It modulates signaling pathways and transcriptional activity, affecting cytokine production and co-stimulatory molecules
Comparison with Similar Compounds
Comparison with Parent Compounds: Chloroquine and Hydroxychloroquine
Structural and Physicochemical Differences
Property | Chloroquine (CQ) | Hydroxychloroquine (HCQ) | Didesethyl Chloroquine (DDCQ) |
---|---|---|---|
Molecular Formula | C₁₈H₂₆ClN₃ | C₁₈H₂₆ClN₃O | C₁₄H₁₈ClN₃ |
Molecular Weight | 319.87 g/mol | 335.87 g/mol | 263.77 g/mol |
Rotatable Bonds | 6 | 6 | 4 (estimated) |
Key Functional Group | Ethyl side chains | Hydroxyl group (+ ethyl) | Two ethyl groups removed |
- Bioavailability : DDCQ’s lower rotatable bonds correlate with higher predicted oral bioavailability compared to CQ and HCQ .
Pharmacological Activity
- Metabolic Role : DDCQ is a terminal metabolite in the CQ/HCQ pathway, contributing to plasma concentrations ≥500 ng/mL in therapeutic settings .
Toxicity Profile
- Cardiotoxicity : DDCQ exhibits potent myocardial depressive effects , inhibiting sodium currents in cardiac tissues, unlike CQ or HCQ .
- Retinal Toxicity: While CQ is associated with retinopathy (19% incidence in long-term use), HCQ shows lower risk (0% in studies), and DDCQ’s role remains underexplored .
Comparison with Other Chloroquine Metabolites
Desethyl Chloroquine (DECQ)
- Structure : Intermediate metabolite with one ethyl group removed (C₁₆H₂₂ClN₃).
- Pharmacokinetics : Shorter half-life than DDCQ, with transient plasma accumulation .
- Activity : Less studied, but DECQ contributes to cumulative antimalarial effects in combination with DDCQ .
Hydroxychloroquine Metabolites
- Desethyl Hydroxychloroquine : Shares structural similarities with DDCQ but retains a hydroxyl group, altering solubility and target interactions .
Research Findings and Therapeutic Potential
COVID-19 Research
- Computational Studies : DDCQ’s cheminformatics profile aligns with CQ/HCQ, supporting its investigation as a SARS-CoV-2 antagonist. Its lower molecular weight and rotatable bonds may improve cellular uptake .
Data Tables
Table 1: Key Physicochemical Properties
Compound | Molecular Weight | Rotatable Bonds | LogP | Oral Bioavailability (Predicted) |
---|---|---|---|---|
Chloroquine | 319.87 | 6 | 4.67 | Moderate |
Hydroxychloroquine | 335.87 | 6 | 3.49 | Moderate |
This compound | 263.77 | 4 | 3.12 | High |
Desethyl Chloroquine | 275.78 | 5 | 3.89 | Moderate |
Table 2: Binding Affinities in Antiviral Studies
Compound | Target (P23pro-zbd) | Binding Affinity (kcal/mol) | Key Residues |
---|---|---|---|
Chloroquine | Chikungunya virus | -7.5 | MET 165, GLN 189 |
This compound | Chikungunya virus | -8.2 | Thr1312, Asp1364, Cys1367 |
Hydroxyacetamide (DDCQ derivative) | Chikungunya virus | -8.5 | Asn1357, Gly1403, Tyr1444 |
Source:
Biological Activity
Didesethyl chloroquine (DDCQ), also known as bisdesethylchloroquine, is a significant metabolite of chloroquine (CQ), an antimalarial drug. Understanding the biological activity of DDCQ is crucial for its potential therapeutic applications, particularly in treating malaria and other viral infections. This article synthesizes current research findings, case studies, and data on the biological activity of DDCQ.
Overview of this compound
- Chemical Structure : DDCQ is characterized by the chemical formula and has a molecular weight of approximately 273.76 g/mol.
- Metabolism : DDCQ is primarily formed through the N-dealkylation of chloroquine by cytochrome P450 enzymes, particularly CYP2C8 and CYP3A4, with further metabolism yielding 7-chloro-4-aminoquinoline as a minor product .
Pharmacological Properties
DDCQ exhibits several pharmacological properties that contribute to its biological activity:
- Antimalarial Activity : DDCQ retains significant antiplasmodial activity against various strains of Plasmodium falciparum, including resistant strains. Studies have reported IC50 values ranging from 0.310 µM to 12.75 µM against different strains .
- Mechanism of Action : Similar to chloroquine, DDCQ inhibits heme polymerase in malarial parasites, leading to the accumulation of toxic heme, which ultimately kills the parasite . Additionally, it has been shown to modulate immune responses by inhibiting toll-like receptors (TLRs) .
Biological Activity Data
The following table summarizes key biological activities and pharmacokinetic properties of DDCQ:
Property | Value/Description |
---|---|
Molecular Formula | |
Molecular Weight | 273.76 g/mol |
Antimalarial IC50 (D10 strain) | 6202.00–9659.70 ng/mL (12.75–19.85 µM) |
Antimalarial IC50 (W2 strain) | 2424.15–5648.07 ng/mL (4.98–11.95 µM) |
Selectivity Index | Higher selectivity against resistant strains compared to CQ |
Toxicity | Minimal hemolysis observed at effective concentrations |
Case Studies
-
Case Study on Antiplasmodial Efficacy :
A study evaluated the efficacy of DDCQ against chloroquine-resistant P. falciparum strains. The results indicated that DDCQ exhibited comparable or superior activity compared to chloroquine, with significantly lower resistance indexes (RI values) indicating its potential as an alternative treatment for resistant malaria cases . -
Clinical Implications in COVID-19 :
During the COVID-19 pandemic, chloroquine and its metabolites, including DDCQ, were investigated for their antiviral properties. Although initial studies suggested potential benefits, subsequent clinical trials highlighted a need for caution due to safety concerns and inconsistent efficacy in preventing COVID-19 symptoms .
Research Findings
Recent research has focused on the molecular docking studies of DDCQ and its derivatives against various viral targets, including chikungunya virus proteases. These studies suggest that modifications in the chemical structure can enhance binding affinity and biological activity against viral targets .
Q & A
Basic Research Questions
Q. How is Didesethyl Chloroquine quantified in biological samples during pharmacokinetic studies?
this compound is quantified using high-performance liquid chromatography coupled with mass spectrometry (HPLC-MS) due to its high sensitivity and specificity. Analytical validation involves spiking biological matrices (e.g., plasma, tissue homogenates) with reference standards to establish linearity, precision, and recovery rates. Stability studies under varying storage conditions (e.g., freeze-thaw cycles) are critical for reliable measurement .
Q. What is the significance of this compound as a metabolite in Chloroquine's pharmacokinetics?
As the primary metabolite of Chloroquine, this compound provides insights into metabolic pathways and hepatic clearance mechanisms. Researchers track its formation using radiolabeled Chloroquine in animal models or in vitro microsomal assays to calculate metabolic half-lives and assess enzyme kinetics (e.g., cytochrome P450 involvement) .
Q. What analytical techniques are recommended for validating this compound concentrations in environmental samples?
Gas chromatography-mass spectrometry (GC-MS) and liquid chromatography-tandem mass spectrometry (LC-MS/MS) are preferred for environmental monitoring. Sample preparation includes solid-phase extraction (SPE) to isolate the compound from complex matrices like water or soil. Method validation requires adherence to EPA or ISO guidelines for detection limits and matrix effects .
Advanced Research Questions
Q. How can molecular docking simulations elucidate this compound's binding affinity to viral proteins like CHIKV P23pro-zbd?
Computational workflows involve homology modeling of the target protein (e.g., CHIKV P23pro-zbd) and docking studies using software like AutoDock Vina. Binding energy calculations and molecular dynamics (MD) simulations (e.g., 100 ns runs) identify key residues (e.g., Thr1312, Asp1364) stabilizing ligand interactions. In vitro validation includes surface plasmon resonance (SPR) to measure dissociation constants (Kd) .
Q. What experimental approaches are used to assess the myocardial inhibitory effects of this compound?
Cardiomyocyte viability assays (e.g., MTT or lactate dehydrogenase release) and patch-clamp electrophysiology evaluate hERG channel inhibition. In vivo models (e.g., Langendorff-perfused hearts) measure changes in cardiac output and arrhythmia incidence. Comparative studies with Chloroquine control for metabolite-specific effects .
Q. How do researchers address discrepancies in toxicity profiles between Chloroquine and its metabolites like this compound?
Dose-response studies in animal models (e.g., rodents) compare organ-specific toxicity using histopathology and serum biomarkers (e.g., troponin for cardiotoxicity). Pharmacokinetic-pharmacodynamic (PK-PD) modeling distinguishes parent drug versus metabolite contributions. Meta-analyses of adverse event databases (e.g., FAERS) identify signal disparities .
Q. What in vitro models are employed to study this compound's role in antimalarial resistance?
Cultured Plasmodium falciparum strains (e.g., chloroquine-resistant K1) are treated with this compound to measure IC50 shifts. Synergism assays with verapamil (a resistance reversal agent) assess PfCRT transporter involvement. Metabolic profiling via LC-MS identifies accumulation patterns in resistant vs. sensitive strains .
Q. Which key amino acid residues are critical for this compound's interaction with viral targets, and how are they identified?
MD simulations and per-residue energy decomposition (e.g., MM-PBSA) highlight residues like Asn1357, Cys1367, and Tyr1444 in CHIKV P23pro-zbd. Alanine scanning mutagenesis followed by binding affinity assays (e.g., isothermal titration calorimetry) confirm their functional roles .
Q. How does the metabolic conversion of Chloroquine to this compound influence experimental outcomes in antimalarial studies?
Co-administration of CYP450 inhibitors (e.g., ketoconazole) in rodent models reduces metabolite formation, enabling direct comparison of antimalarial efficacy. Pharmacokinetic compartmental models simulate metabolite exposure to correlate with parasite clearance rates .
Q. What strategies are used to differentiate the cytotoxic effects of this compound from its parent compound in cardiac tissue models?
Dual-treatment designs with Chloroquine and pre-formed this compound isolate metabolite-specific effects. Transcriptomic profiling (e.g., RNA-seq) identifies unique pathways (e.g., oxidative stress vs. calcium signaling). CRISPR-edited cardiomyocytes (e.g., hERG-knockout) clarify target specificity .
Properties
IUPAC Name |
4-N-(7-chloroquinolin-4-yl)pentane-1,4-diamine | |
---|---|---|
Source | PubChem | |
URL | https://pubchem.ncbi.nlm.nih.gov | |
Description | Data deposited in or computed by PubChem | |
InChI |
InChI=1S/C14H18ClN3/c1-10(3-2-7-16)18-13-6-8-17-14-9-11(15)4-5-12(13)14/h4-6,8-10H,2-3,7,16H2,1H3,(H,17,18) | |
Source | PubChem | |
URL | https://pubchem.ncbi.nlm.nih.gov | |
Description | Data deposited in or computed by PubChem | |
InChI Key |
GYEDIFVVTRKXHP-UHFFFAOYSA-N | |
Source | PubChem | |
URL | https://pubchem.ncbi.nlm.nih.gov | |
Description | Data deposited in or computed by PubChem | |
Canonical SMILES |
CC(CCCN)NC1=C2C=CC(=CC2=NC=C1)Cl | |
Source | PubChem | |
URL | https://pubchem.ncbi.nlm.nih.gov | |
Description | Data deposited in or computed by PubChem | |
Molecular Formula |
C14H18ClN3 | |
Source | PubChem | |
URL | https://pubchem.ncbi.nlm.nih.gov | |
Description | Data deposited in or computed by PubChem | |
DSSTOX Substance ID |
DTXSID70962823 | |
Record name | N~4~-(7-Chloroquinolin-4-yl)pentane-1,4-diamine | |
Source | EPA DSSTox | |
URL | https://comptox.epa.gov/dashboard/DTXSID70962823 | |
Description | DSSTox provides a high quality public chemistry resource for supporting improved predictive toxicology. | |
Molecular Weight |
263.76 g/mol | |
Source | PubChem | |
URL | https://pubchem.ncbi.nlm.nih.gov | |
Description | Data deposited in or computed by PubChem | |
CAS No. |
4298-14-0 | |
Record name | Bisdesethylchloroquine | |
Source | CAS Common Chemistry | |
URL | https://commonchemistry.cas.org/detail?cas_rn=4298-14-0 | |
Description | CAS Common Chemistry is an open community resource for accessing chemical information. Nearly 500,000 chemical substances from CAS REGISTRY cover areas of community interest, including common and frequently regulated chemicals, and those relevant to high school and undergraduate chemistry classes. This chemical information, curated by our expert scientists, is provided in alignment with our mission as a division of the American Chemical Society. | |
Explanation | The data from CAS Common Chemistry is provided under a CC-BY-NC 4.0 license, unless otherwise stated. | |
Record name | N,N-Dideethylchloroquine | |
Source | ChemIDplus | |
URL | https://pubchem.ncbi.nlm.nih.gov/substance/?source=chemidplus&sourceid=0004298140 | |
Description | ChemIDplus is a free, web search system that provides access to the structure and nomenclature authority files used for the identification of chemical substances cited in National Library of Medicine (NLM) databases, including the TOXNET system. | |
Record name | N~4~-(7-Chloroquinolin-4-yl)pentane-1,4-diamine | |
Source | EPA DSSTox | |
URL | https://comptox.epa.gov/dashboard/DTXSID70962823 | |
Description | DSSTox provides a high quality public chemistry resource for supporting improved predictive toxicology. | |
Record name | BISDESETHYLCHLOROQUINE | |
Source | FDA Global Substance Registration System (GSRS) | |
URL | https://gsrs.ncats.nih.gov/ginas/app/beta/substances/M48JF33RF8 | |
Description | The FDA Global Substance Registration System (GSRS) enables the efficient and accurate exchange of information on what substances are in regulated products. Instead of relying on names, which vary across regulatory domains, countries, and regions, the GSRS knowledge base makes it possible for substances to be defined by standardized, scientific descriptions. | |
Explanation | Unless otherwise noted, the contents of the FDA website (www.fda.gov), both text and graphics, are not copyrighted. They are in the public domain and may be republished, reprinted and otherwise used freely by anyone without the need to obtain permission from FDA. Credit to the U.S. Food and Drug Administration as the source is appreciated but not required. | |
Retrosynthesis Analysis
AI-Powered Synthesis Planning: Our tool employs the Template_relevance Pistachio, Template_relevance Bkms_metabolic, Template_relevance Pistachio_ringbreaker, Template_relevance Reaxys, Template_relevance Reaxys_biocatalysis model, leveraging a vast database of chemical reactions to predict feasible synthetic routes.
One-Step Synthesis Focus: Specifically designed for one-step synthesis, it provides concise and direct routes for your target compounds, streamlining the synthesis process.
Accurate Predictions: Utilizing the extensive PISTACHIO, BKMS_METABOLIC, PISTACHIO_RINGBREAKER, REAXYS, REAXYS_BIOCATALYSIS database, our tool offers high-accuracy predictions, reflecting the latest in chemical research and data.
Strategy Settings
Precursor scoring | Relevance Heuristic |
---|---|
Min. plausibility | 0.01 |
Model | Template_relevance |
Template Set | Pistachio/Bkms_metabolic/Pistachio_ringbreaker/Reaxys/Reaxys_biocatalysis |
Top-N result to add to graph | 6 |
Feasible Synthetic Routes
Disclaimer and Information on In-Vitro Research Products
Please be aware that all articles and product information presented on BenchChem are intended solely for informational purposes. The products available for purchase on BenchChem are specifically designed for in-vitro studies, which are conducted outside of living organisms. In-vitro studies, derived from the Latin term "in glass," involve experiments performed in controlled laboratory settings using cells or tissues. It is important to note that these products are not categorized as medicines or drugs, and they have not received approval from the FDA for the prevention, treatment, or cure of any medical condition, ailment, or disease. We must emphasize that any form of bodily introduction of these products into humans or animals is strictly prohibited by law. It is essential to adhere to these guidelines to ensure compliance with legal and ethical standards in research and experimentation.