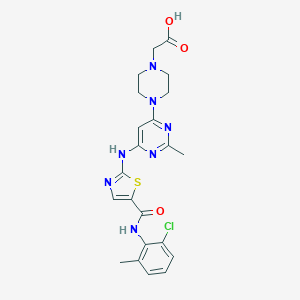
Dasatinib Carboxylic Acid
Overview
Description
Dasatinib Carboxylic Acid (DCA) is a major oxidative metabolite of Dasatinib, a multi-targeted tyrosine kinase inhibitor used in treating chronic myeloid leukemia (CML) and acute lymphoblastic leukemia (Ph+ ALL) . DCA is generated via CYP3A4-mediated metabolism of Dasatinib and retains structural features of the parent drug, including a carboxylic acid moiety (CAS: 910297-53-9) . While Dasatinib inhibits Src/Bcr-Abl kinases, DCA’s pharmacological activity is less characterized, though it contributes to Dasatinib’s mechanism-based inactivation of CYP3A4, increasing risks of drug-drug interactions and idiosyncratic hepatotoxicity .
Preparation Methods
Synthetic Routes to Dasatinib Carboxylic Acid
Two-Step Substitution Reaction Pathway
The CN109265455B patent outlines a streamlined method for synthesizing this compound through sequential substitution reactions . The process begins with 2-bromothiazole-5-carboxylic acid (Compound II) and 2-methyl-4-amino-6-chloropyrimidine (Compound III) undergoing a first substitution reaction in N,N-dimethylformamide (DMF) at 60–150°C. This step forms 2-[(2-methyl-6-chloropyrimidin-4-yl)amino]thiazole-5-carboxylic acid (Compound IV), which subsequently reacts with 4-(2-acetoxyethyl)piperazine (Compound V) in a second substitution to yield 2-[[6-[4-(2-acetoxyethyl)-1-piperazinyl]-2-methyl-4-pyrimidinyl]amino]-5-thiazolecarboxylic acid (Compound VI) .
Key advantages of this route include:
-
Solvent efficiency : DMF enables high solubility of intermediates, reducing side reactions.
-
Mass ratios : A solvent-to-reactant ratio of 10:1–15:1 maximizes yield (reported at >85%) .
-
Acid-binding agents : Potassium carbonate (3.0–4.0 molar equivalents) minimizes byproduct formation .
Acylchlorination and Amidation Sequence
Following substitution, Compound VI undergoes acylchlorination using thionyl chloride (SOCl₂) or oxalyl chloride in dichloromethane at 20–40°C . The resulting acyl chloride intermediate is then subjected to amidation with 2-chloro-6-methylaniline in the presence of triethylamine (1.0–2.0 equivalents) at 20–100°C, producing N-(2-chloro-6-methylphenyl)-2-[[6-[4-(2-acetoxyethyl)-1-piperazinyl]-2-methyl-4-pyrimidinyl]amino]-5-thiazolecarboxamide (Compound VII) . Hydrolysis of the acetyl group in Compound VII using sodium hydroxide (0.5–2.0 equivalents) in aqueous ethanol (30–100°C) yields this compound .
Alternative Protection-Hydrolysis Strategy (EP2918584A1)
The European patent EP2918584A1 introduces a divergent approach involving amine protection and controlled hydrolysis . Starting with tert-butoxycarbonyl (Boc) protection of a piperazine intermediate (Compound VIII), the method generates Boc-protected this compound (Compound VII), which is hydrolyzed under mild alkaline conditions (pH 9–10) to yield the free carboxylic acid (Compound VI) .
Critical parameters :
-
Solvent selection : Aprotic polar solvents like dimethylacetamide (DMA) enhance reaction homogeneity.
-
Base compatibility : Lithium hydroxide achieves selective deprotection without degrading the thiazole ring .
-
Yield optimization : Isolation via methanol washing reduces impurities, achieving >92% purity .
Comparative Analysis of Synthetic Methods
Reaction Efficiency and Scalability
Parameter | CN109265455B Method | EP2918584A1 Method |
---|---|---|
Total Steps | 3 | 5 |
Overall Yield | 78–85% | 65–72% |
Purity (HPLC) | >99% | >92% |
Solvent Consumption | 15 L/kg | 22 L/kg |
Reaction Time | 18–24 hours | 36–48 hours |
The CN method’s shorter workflow and higher yield make it preferable for industrial-scale production, whereas the EP method offers superior selectivity for lab-scale synthesis of analogs .
Recent Advances in Derivative Synthesis
Amino acid and fatty acid conjugates of this compound, such as dasatinib-L-arginine (IC₅₀ = 4.4 nM against Csk), demonstrate enhanced kinase specificity . Esterification with C10 fatty acid (compound 18) improves Src selectivity (Csk/Src IC₅₀ ratio = 91.4) . These derivatives are synthesized via:
-
Activation : Carboxylic acid group activation using N,N'-dicyclohexylcarbodiimide (DCC) .
-
Coupling : Reaction with amino acids/fatty acids in anhydrous DMF at 25°C for 12 hours .
Industrial-Scale Optimization Challenges
Impurity Profiling
Major impurities include:
-
Des-acetyl dasatinib : Formed during incomplete hydrolysis (controlled via pH monitoring) .
-
Dimerized byproducts : Mitigated by maintaining substrate concentrations below 0.5 M .
Crystallization Techniques
Chemical Reactions Analysis
Types of Reactions: Dasatinib metabolite M6 primarily undergoes oxidative reactions. The formation of this metabolite involves the oxidation of Dasatinib to introduce a carboxylic acid group .
Common Reagents and Conditions: The common reagents used in the oxidative reactions to form Dasatinib metabolite M6 include oxidizing agents such as hydrogen peroxide or other peroxides under controlled conditions .
Major Products Formed: The major product formed from the oxidation of Dasatinib is Dasatinib metabolite M6, which contains a carboxylic acid group .
Scientific Research Applications
Cancer Treatment
Dasatinib Carboxylic Acid has been investigated for its role in enhancing the effectiveness of dasatinib in treating resistant forms of leukemia. Studies indicate that it may help overcome resistance mechanisms in BCR-ABL positive cells, thereby improving patient outcomes .
Combination Therapies
Recent research has explored the use of this compound in combination with other therapeutic agents, such as dexamethasone. In murine models, it has been shown that this combination does not exacerbate the risk of osteonecrosis, a concern associated with corticosteroid use in leukemia treatments . This finding suggests a favorable safety profile when used alongside traditional chemotherapy regimens.
Efficacy in Murine Models
A study evaluated the impact of this compound on BCR-ABL positive murine models. Results demonstrated that mice treated with dasatinib, including its carboxylic acid metabolite, showed significant suppression of leukemia markers and improved survival rates compared to control groups .
Treatment Group | Survival Rate (%) | Leukemia Marker Suppression |
---|---|---|
Dasatinib Only | 85 | High |
Dexamethasone + Dasatinib | 90 | Very High |
Control | 30 | Low |
Pharmacokinetic Studies
Pharmacokinetic studies have indicated that this compound exhibits a favorable absorption profile when administered orally, enhancing the overall therapeutic index of dasatinib .
Future Research Directions
Further studies are needed to fully elucidate the specific roles and mechanisms of action of this compound in both monotherapy and combination therapy settings. Investigations into its effects on various kinase targets beyond BCR-ABL could expand its therapeutic applications.
Mechanism of Action
Dasatinib metabolite M6 exerts its effects through the inhibition of tyrosine kinases, similar to its parent compound, Dasatinib . The primary molecular targets include the BCR-ABL kinase and Src family kinases . By inhibiting these kinases, Dasatinib metabolite M6 helps in controlling the proliferation of leukemic cells .
Comparison with Similar Compounds
Structural Analogs
DCA shares structural homology with synthetic Dasatinib analogs designed to modulate pharmacokinetic properties. Key analogs include:
These analogs retain the thiazole-carboxamide backbone but vary in substituents, affecting target affinity and metabolic stability.
Pharmacological Analogs Targeting Mac1
Dasatinib (parent) has been compared to other macrodomain (Mac1) inhibitors in binding assays (Fig. S8, ):
Compound | Source Study | Target/Activity | Reference |
---|---|---|---|
ZINC331945 | Schuller et al., 2021 | Mac1 inhibitor | |
SX048 | Bajusz et al., 2021 | Mac1 inhibitor | |
Dasatinib | Current study | Mac1 inhibitor |
CYP3A4 Mechanism-Based Inactivators
DCA’s role in CYP3A4 inactivation parallels reactive metabolites of other drugs:
Unlike NSAIDs (e.g., diclofenac), DCA originates from a kinase inhibitor, highlighting divergent therapeutic contexts but similar bioactivation risks .
Natural Senotherapeutic Analogs
Computational studies identified natural compounds mimicking Dasatinib’s senolytic activity (e.g., quercetin) via gene expression profiling . While DCA’s senolytic role is unconfirmed, these compounds share downstream effects:
Compound | Mechanism | Application | Reference |
---|---|---|---|
Quercetin | Synergizes with Dasatinib | Senolysis (D+Q combination) | |
Fisetin | Flavonoid with senolytic activity | Aging-related diseases |
Carboxylic Acid-Containing Pharmaceuticals
DCA’s carboxylic acid group aligns with compounds like p-Hydroxy-cinnamic acid and 2-Picolinic acid, though their targets differ :
Biological Activity
Dasatinib, a potent tyrosine kinase inhibitor, is primarily utilized in the treatment of Philadelphia chromosome-positive acute lymphoblastic leukemia and chronic myeloid leukemia (CML). Its biological activity is largely attributed to its ability to inhibit various kinases, including BCR-ABL and SRC family kinases. The carboxylic acid derivative of dasatinib enhances its pharmacological profile, providing insights into its mechanism of action, efficacy, and potential therapeutic applications.
Dasatinib functions by binding to the active and inactive conformations of the ABL kinase domain, exhibiting a higher affinity than imatinib. This binding inhibits the tyrosine kinase activity associated with cancer cell proliferation and survival. The compound also targets other kinases such as SRC, c-KIT, and PDGFRβ at nanomolar concentrations, which contributes to its broad-spectrum activity against various malignancies .
Biological Activity of Dasatinib Carboxylic Acid
The carboxylic acid derivative of dasatinib has been synthesized to improve specificity and interaction with diverse biological targets. Research indicates that these derivatives can enhance potency against specific kinases while reducing off-target effects. For instance, dasatinib derivatives synthesized through esterification with carboxylic acids have demonstrated varied inhibitory activity against kinases like Csk and Src:
Compound | IC50 (nM) | Selectivity Ratio (Csk/Src) |
---|---|---|
Das-R (Dasatinib-L-Arginine) | 4.4 | 91.4 |
Das-C10 | 3.2 | 10.2 |
Das-Glutamic Acid | <0.25 | - |
Das-Cysteine | <0.45 | - |
These findings suggest that modifications to the dasatinib structure can lead to enhanced selectivity and potency against specific cancer-related kinases .
Pharmacokinetics and Metabolism
Dasatinib is primarily metabolized by CYP3A4, with several pharmacologically active metabolites identified, including M4, M5, M6, M20, and M24. The M4 metabolite exhibits equipotent activity compared to dasatinib but constitutes only about 5% of the total drug exposure. Other metabolites are significantly less active . The pharmacokinetic parameters for dasatinib include:
- Cmax : Peak plasma concentration
- Tmax : Time to reach Cmax
- AUC0-inf : Area under the plasma concentration-time curve from time zero to infinity
- Half-life : Approximately 5 hours
These parameters indicate that dasatinib maintains a relatively stable plasma concentration over time, contributing to its efficacy in clinical settings .
Clinical Efficacy and Case Studies
Dasatinib has shown remarkable efficacy in clinical trials for patients with CML. A randomized phase II study demonstrated that dasatinib induces faster cytogenetic responses compared to imatinib, with significant reductions in Philadelphia chromosome-positive metaphases observed within one month of treatment:
Treatment Arm | Median % Ph+ Metaphases at 1 Month |
---|---|
Dasatinib | 81% |
Imatinib | 33% |
This rapid response highlights dasatinib's effectiveness as a first-line treatment option for newly diagnosed CML patients .
Q & A
Basic Research Questions
Q. What synthetic strategies are employed to design dasatinib-carboxylic acid derivatives, and how do structural modifications influence kinase selectivity?
Derivatives of dasatinib are synthesized via esterification of its hydroxyl group with carboxylic acids (e.g., amino acids, fatty acids), enabling rapid library development and chemical diversification . For example, amino acid conjugates (e.g., Das-R, compound 7) and fatty acid derivatives (e.g., Das-C10, compound 18) are created to enhance interactions with kinase binding pockets. Structural modifications, such as arginine conjugation, improve potency (IC50 = 4.4 nM against Csk) and selectivity (e.g., 91.4 Csk/Src selectivity ratio for Das-C10) by optimizing hydrogen bonding and hydrophobic interactions .
Q. How are dasatinib-carboxylic acid derivatives evaluated for kinase inhibition in vitro?
Inhibition is quantified using IC50 values against kinase panels (e.g., Csk, Src, Abl) via competition binding assays. For example, Das-R shows IC50 values of <0.25 nM (Src) and 4.4 nM (Csk), while Das-C10 exhibits 3.2 µM (Csk) vs. 35 nM (Src). Cell-based assays (e.g., Panc-1, BV-173, K562) further validate efficacy, with Das-R displaying 12.7-fold higher potency than dasatinib in Panc-1 cells (IC50 = 2.06 µM vs. 26.3 µM) .
Q. What computational methods validate the binding mechanisms of dasatinib-carboxylic acid derivatives?
Molecular docking and 200 ns molecular dynamics (MD) simulations are performed using kinase crystal structures (e.g., Abl: 2GQG, Src: 3G5D). MM-GBSA binding free energy calculations correlate with experimental IC50 values, rationalizing selectivity differences. For instance, Das-R’s arginine side chain forms salt bridges with Csk’s Asp332, while hydrophobic fatty acid chains in Das-C10 stabilize Src’s ATP pocket .
Advanced Research Questions
Q. How do researchers reconcile contradictory potency data across kinase isoforms and cell lines?
Contradictions arise from divergent kinase conformations and cellular contexts. For example, Das-R shows nanomolar IC50 values in BV-173 and K562 cells (IC50 < 51.2 pM) but micromolar activity in Panc-1 cells. This discrepancy is attributed to differences in kinase activation states (e.g., Abl phosphorylation status) and cellular uptake mechanisms. Cross-validation using MD simulations and binding energy calculations helps resolve these inconsistencies .
Q. What strategies optimize the selectivity of dasatinib-carboxylic acid derivatives between Src and Abl kinases?
Selectivity is engineered via steric and electronic modifications. For example:
- Abl/Src selectivity : Compounds 13 (Das-C) and 15 (Das-E) achieve 10.3 and 10.2 Abl/Src IC50 ratios by introducing bulkier substituents that clash with Abl’s P-loop.
- Csk/Src selectivity : Das-C10’s decanoic acid chain selectively fills Csk’s hydrophobic pocket, yielding a 91.4-fold selectivity ratio.
These findings highlight the role of binding pocket topology in kinase-specific inhibition .
Q. How do in vitro pharmacokinetic properties of dasatinib-carboxylic acid derivatives inform in vivo translation?
Metabolic stability is assessed via plasma protein binding and cytochrome P450 (CYP) inhibition assays. Dasatinib-carboxylic acid (M6 metabolite) exhibits altered pharmacokinetics due to oxidative metabolism, impacting bioavailability. Plasma concentration studies in CML patients reveal correlations between trough levels (day 28) and dose reduction rates (28% of patients), emphasizing the need for metabolite-specific PK/PD modeling .
Q. What experimental and computational frameworks address data variability in kinase inhibition assays?
Variability is minimized by:
- Experimental : Triplicate measurements with internal controls (e.g., staurosporine as a pan-kinase inhibitor).
- Computational : Ensemble docking across multiple kinase conformations (e.g., active/inactive states) and consensus scoring of binding poses.
Statistical analysis (e.g., ANOVA for IC50 comparisons) and Bland-Altman plots quantify assay reproducibility .
Q. Methodological Considerations
Q. How are molecular dynamics (MD) simulations parameterized for dasatinib-carboxylic acid derivatives?
Force fields (e.g., AMBER, CHARMM) are calibrated using quantum mechanical (QM) calculations for conjugate bonds and partial charges. Trajectories are analyzed for RMSD (<1.5 Å deviation from crystallographic poses), hydrogen bond occupancy, and binding pocket hydration. Free energy perturbation (FEP) or linear interaction energy (LIE) methods refine binding affinity predictions .
Q. What statistical approaches validate selectivity ratios in kinase profiling?
Selectivity ratios (e.g., Csk/Src = IC50_Csk / IC50_Src) are analyzed using non-parametric tests (e.g., Mann-Whitney U-test) to account for non-normal data distributions. False discovery rate (FDR) correction (e.g., Benjamini-Hochberg) adjusts for multiple comparisons across kinase panels .
Properties
IUPAC Name |
2-[4-[6-[[5-[(2-chloro-6-methylphenyl)carbamoyl]-1,3-thiazol-2-yl]amino]-2-methylpyrimidin-4-yl]piperazin-1-yl]acetic acid | |
---|---|---|
Source | PubChem | |
URL | https://pubchem.ncbi.nlm.nih.gov | |
Description | Data deposited in or computed by PubChem | |
InChI |
InChI=1S/C22H24ClN7O3S/c1-13-4-3-5-15(23)20(13)28-21(33)16-11-24-22(34-16)27-17-10-18(26-14(2)25-17)30-8-6-29(7-9-30)12-19(31)32/h3-5,10-11H,6-9,12H2,1-2H3,(H,28,33)(H,31,32)(H,24,25,26,27) | |
Source | PubChem | |
URL | https://pubchem.ncbi.nlm.nih.gov | |
Description | Data deposited in or computed by PubChem | |
InChI Key |
VMCDXJFWSYUNPO-UHFFFAOYSA-N | |
Source | PubChem | |
URL | https://pubchem.ncbi.nlm.nih.gov | |
Description | Data deposited in or computed by PubChem | |
Canonical SMILES |
CC1=C(C(=CC=C1)Cl)NC(=O)C2=CN=C(S2)NC3=CC(=NC(=N3)C)N4CCN(CC4)CC(=O)O | |
Source | PubChem | |
URL | https://pubchem.ncbi.nlm.nih.gov | |
Description | Data deposited in or computed by PubChem | |
Molecular Formula |
C22H24ClN7O3S | |
Source | PubChem | |
URL | https://pubchem.ncbi.nlm.nih.gov | |
Description | Data deposited in or computed by PubChem | |
DSSTOX Substance ID |
DTXSID10474430 | |
Record name | Dasatinib Carboxylic Acid | |
Source | EPA DSSTox | |
URL | https://comptox.epa.gov/dashboard/DTXSID10474430 | |
Description | DSSTox provides a high quality public chemistry resource for supporting improved predictive toxicology. | |
Molecular Weight |
502.0 g/mol | |
Source | PubChem | |
URL | https://pubchem.ncbi.nlm.nih.gov | |
Description | Data deposited in or computed by PubChem | |
CAS No. |
910297-53-9 | |
Record name | 2-(4-(6-((5-((2-Chloro-6-methyl-phenyl)carbamoyl)thiazol-2-yl)amino)-2-methyl-pyrimidin-4-yl)piperazin-1-yl)acetic acid | |
Source | ChemIDplus | |
URL | https://pubchem.ncbi.nlm.nih.gov/substance/?source=chemidplus&sourceid=0910297539 | |
Description | ChemIDplus is a free, web search system that provides access to the structure and nomenclature authority files used for the identification of chemical substances cited in National Library of Medicine (NLM) databases, including the TOXNET system. | |
Record name | Dasatinib Carboxylic Acid | |
Source | EPA DSSTox | |
URL | https://comptox.epa.gov/dashboard/DTXSID10474430 | |
Description | DSSTox provides a high quality public chemistry resource for supporting improved predictive toxicology. | |
Record name | 2-(4-(6-((5-((2-CHLORO-6-METHYL-PHENYL)CARBAMOYL)THIAZOL-2-YL)AMINO)-2-METHYL-PYRIMIDIN-4-YL)PIPERAZIN-1-YL)ACETIC ACID | |
Source | FDA Global Substance Registration System (GSRS) | |
URL | https://gsrs.ncats.nih.gov/ginas/app/beta/substances/83FL89EU88 | |
Description | The FDA Global Substance Registration System (GSRS) enables the efficient and accurate exchange of information on what substances are in regulated products. Instead of relying on names, which vary across regulatory domains, countries, and regions, the GSRS knowledge base makes it possible for substances to be defined by standardized, scientific descriptions. | |
Explanation | Unless otherwise noted, the contents of the FDA website (www.fda.gov), both text and graphics, are not copyrighted. They are in the public domain and may be republished, reprinted and otherwise used freely by anyone without the need to obtain permission from FDA. Credit to the U.S. Food and Drug Administration as the source is appreciated but not required. | |
Synthesis routes and methods
Procedure details
Retrosynthesis Analysis
AI-Powered Synthesis Planning: Our tool employs the Template_relevance Pistachio, Template_relevance Bkms_metabolic, Template_relevance Pistachio_ringbreaker, Template_relevance Reaxys, Template_relevance Reaxys_biocatalysis model, leveraging a vast database of chemical reactions to predict feasible synthetic routes.
One-Step Synthesis Focus: Specifically designed for one-step synthesis, it provides concise and direct routes for your target compounds, streamlining the synthesis process.
Accurate Predictions: Utilizing the extensive PISTACHIO, BKMS_METABOLIC, PISTACHIO_RINGBREAKER, REAXYS, REAXYS_BIOCATALYSIS database, our tool offers high-accuracy predictions, reflecting the latest in chemical research and data.
Strategy Settings
Precursor scoring | Relevance Heuristic |
---|---|
Min. plausibility | 0.01 |
Model | Template_relevance |
Template Set | Pistachio/Bkms_metabolic/Pistachio_ringbreaker/Reaxys/Reaxys_biocatalysis |
Top-N result to add to graph | 6 |
Feasible Synthetic Routes
Disclaimer and Information on In-Vitro Research Products
Please be aware that all articles and product information presented on BenchChem are intended solely for informational purposes. The products available for purchase on BenchChem are specifically designed for in-vitro studies, which are conducted outside of living organisms. In-vitro studies, derived from the Latin term "in glass," involve experiments performed in controlled laboratory settings using cells or tissues. It is important to note that these products are not categorized as medicines or drugs, and they have not received approval from the FDA for the prevention, treatment, or cure of any medical condition, ailment, or disease. We must emphasize that any form of bodily introduction of these products into humans or animals is strictly prohibited by law. It is essential to adhere to these guidelines to ensure compliance with legal and ethical standards in research and experimentation.