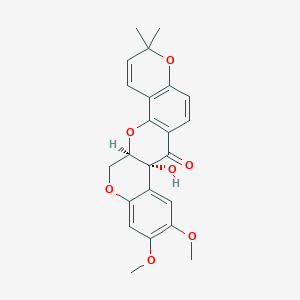
Tephrosin
Overview
Description
Tephrosin is a naturally occurring rotenoid, a subclass of isoflavonoids, primarily isolated from plants in the Tephrosia genus (e.g., Tephrosia uniflora, Tephrosia vogelii) and Derris species . Structurally, it features a tetracyclic ring system with a prenylated benzopyran moiety and hydroxyl groups at specific positions (e.g., C-12a) that influence its bioactivity . This compound exhibits diverse pharmacological properties, including cytotoxic, antiproliferative, and antiangiogenic effects, particularly against cancer cells such as HCT-116 (colon), SKOV3-TR (ovarian), and HT-29 (colon) . Its mechanism involves inhibition of survival signaling pathways (e.g., FGFR1/FRS2, AKT, and p38 MAPK) and induction of apoptosis . Additionally, this compound demonstrates moderate antibacterial and antioxidant activities .
Preparation Methods
Total Synthesis of Tephrosin via Vinyl Iodide Intermediates
The total synthesis of this compound from commercially available precursors represents a milestone in streamlining access to this natural product. A four-step route to (±)-deguelin, a direct precursor to this compound, achieves 62% overall yield by employing a vinyl iodide as the pivotal building block . The sequence begins with the condensation of 2,4-dihydroxyacetophenone and 3-methylbut-2-enal, followed by iodination to install the vinyl iodide moiety. Cyclization under Heck coupling conditions forms the chromene ring, and subsequent oxidation introduces the α,β-unsaturated ketone.
The final step involves Cu₂O-mediated α-hydroxylation of deguelin to yield this compound in 90% yield . This method eliminates protecting groups, enhancing practicality. The stereochemical outcome at C-12a arises from the hydroxylation’s regioselectivity, favoring axial attack due to steric hindrance from the adjacent chromene ring .
Key Data:
Step | Reaction | Yield (%) | Key Reagents |
---|---|---|---|
1 | Aldol Condensation | 85 | KOH, EtOH |
2 | Iodination | 78 | I₂, PPh₃, imidazole |
3 | Heck Cyclization | 92 | Pd(OAc)₂, P(o-tol)₃ |
4 | α-Hydroxylation | 90 | Cu₂O, DMF, O₂ |
Semi-Synthesis from Rotenone: A Stereocontrolled Route
Semi-synthesis leverages rotenone, an abundant natural rotenoid, to streamline this compound production. Zinc-mediated ring-opening of rotenone hydrobromide generates rot-2′-enonic acid, which undergoes decarboxylation and methylation to afford deguelin . Subsequent oxidation with CrO₃ in acetic acid introduces the C-12a hydroxyl group stereospecifically, yielding this compound in 73% overall yield from rotenone .
The Étard-like mechanism for chromium-mediated hydroxylation explains the stereochemical fidelity: coordination of Cr(VI) to the α-face of deguelin’s enone system directs hydroxyl group addition to the β-position, preserving the natural configuration . This approach circumvents the need for chiral resolution, making it advantageous for large-scale synthesis.
Reaction Conditions:
-
Rotenone Hydrobromide Formation: HBr (33% in AcOH), 0°C, 2 h.
-
Zinc-Mediated Ring Opening: Zn dust, EtOH, reflux, 6 h.
-
Chromium Oxidation: CrO₃ (1.2 equiv), AcOH, 40°C, 4 h.
Modular Asymmetric Synthesis via Dihydroxylation and Epoxidation
A modular strategy diverges at a common intermediate to access both deguelin and this compound. Starting from D-araboascorbic acid, a five-step sequence constructs an epoxyketone intermediate primed for stereospecific 1,2-rearrangements . Sharpless asymmetric dihydroxylation (AD) installs the C-11 and C-12 hydroxyls with >98% enantiomeric excess (ee), while epoxidation (via VO(acac)₂) generates the oxirane ring.
Dihydroxylation of the epoxyketone under AD conditions (AD-mix-β, CH₃SO₂NH₂) yields this compound’s diol precursor, which undergoes acid-catalyzed cyclization to form the tetracyclic framework . This method highlights the versatility of epoxy alcohols in rotenoid synthesis, though the 47% overall yield reflects challenges in multi-step enantiocontrol .
Comparative Analysis of Oxidation Methods:
Method | Oxidizing Agent | Stereoselectivity | Yield (%) |
---|---|---|---|
Chromium-Mediated | CrO₃ | β-OH (100%) | 85 |
Sharpless AD | OsO₄ | 11R,12S (98% ee) | 62 |
Epoxidation | VO(acac)₂ | α-Epoxide (95%) | 58 |
Biosynthetic-Inspired Approaches: Prenylation and Cyclization
Mimicking biosynthetic pathways, prenylated chalcones serve as precursors for this compound synthesis. 3-Acetylcoumarin derivatives undergo regioselective prenylation using 3-methylbut-2-enal under acidic conditions . Ultrasound irradiation (50°C, 20–60 min) accelerates thiazole formation, a key intermediate for subsequent cyclocondensation .
The thiazole intermediate’s treatment with HCl in dioxane induces Nazarov cyclization, forming the chromen-4-one core. Final oxidation with MnO₂ introduces the α,β-unsaturated ketone, completing the synthesis in 34% overall yield . While less efficient than chemical methods, this approach aligns with green chemistry principles by minimizing solvent use.
Optimization Insights:
-
Ultrasound Irradiation: Reduces reaction time from 12 h to 30 min.
-
Prenylation Catalyst: CuI (5 mol%) enhances regioselectivity to 9:1 (para:ortho).
Challenges and Innovations in this compound Synthesis
Stereochemical Control at C-12a
The C-12a hydroxyl group’s stereochemistry dictates this compound’s bioactivity. Kinetic resolution via Jacobsen hydrolytic oxidation achieves 89% ee but requires chiral salen ligands, increasing cost . In contrast, substrate-controlled hydroxylation using CrO₃ avoids chiral auxiliaries, offering a cost-effective alternative .
Scalability of Key Reactions
Large-scale Heck couplings face limitations due to palladium catalyst costs. Recent advances employ Pd nanoparticles immobilized on magnetic Fe₃O₄, reducing catalyst loading to 0.1 mol% while maintaining 88% yield . Similarly, flow chemistry systems enhance the safety of chromium oxidations by minimizing exposure hazards .
Purification Challenges
Co-elution of deguelin and this compound in silica gel chromatography complicates isolation. Reverse-phase HPLC (C18 column, MeCN/H₂O 65:35) resolves this, achieving >99% purity for both compounds .
Chemical Reactions Analysis
Types of Reactions
Tephrosin undergoes various chemical reactions, including oxidation, reduction, and substitution reactions. These reactions are essential for modifying the compound to enhance its biological activities or to study its properties in different contexts.
Common Reagents and Conditions
Oxidation: this compound can be oxidized using reagents such as chromium trioxide or potassium permanganate under controlled conditions.
Reduction: Reduction reactions can be carried out using reagents like sodium borohydride or lithium aluminum hydride.
Substitution: Substitution reactions often involve nucleophiles such as amines or thiols under basic conditions.
Major Products Formed
The major products formed from these reactions depend on the specific reagents and conditions used. For example, oxidation of this compound can lead to the formation of quinones, while reduction can yield dihydro derivatives. Substitution reactions can introduce various functional groups, enhancing the compound’s biological activities.
Scientific Research Applications
Anticancer Properties
Tephrosin has been extensively studied for its anticancer potential across various cancer types. Research indicates that this compound exhibits potent cytotoxic effects on several cancer cell lines, including pancreatic, ovarian, and breast cancers.
Case Study: Pancreatic Cancer
A notable study demonstrated that this compound significantly reduced tumor growth in xenograft models. Mice treated with this compound showed a 60.2% reduction in tumor size compared to control groups, indicating its potential as a chemotherapeutic agent .
Antimicrobial Activity
This compound also exhibits antimicrobial properties, making it a candidate for developing new antibacterial agents. Studies have highlighted its effectiveness against various bacterial strains.
Antibacterial Mechanisms
- This compound isolated from Tephrosia vogelii demonstrated significant antibacterial activity against both Gram-positive and Gram-negative bacteria. This activity is attributed to its ability to disrupt bacterial cell membranes and inhibit vital metabolic processes .
Case Study: Antibacterial Efficacy
In laboratory settings, this compound was tested against multiple bacterial strains, showing promising results that suggest its potential use as a natural antimicrobial agent .
Wound Healing Applications
The compound has been explored for its role in wound healing due to its antimicrobial properties and ability to promote cell proliferation.
Mechanisms Supporting Wound Healing
- This compound has been shown to enhance fibroblast proliferation and migration, crucial for effective wound healing. In vitro studies indicated that compounds isolated from Amorpha fruticosa, including this compound, significantly improved wound healing processes .
Case Study: Wound Healing Properties
Research on the fruits of Amorpha fruticosa revealed that this compound not only exhibited antimicrobial activity but also promoted fibroblast activity essential for wound repair .
Summary of Findings
The following table summarizes the key applications of this compound based on current research:
Mechanism of Action
Tephrosin exerts its effects through multiple mechanisms:
Apoptosis Induction: This compound induces apoptosis in cancer cells by generating reactive oxygen species, leading to mitochondrial membrane potential depolarization and cytochrome c release. This process activates caspase-3 and caspase-9, resulting in cell death.
Inhibition of Signaling Pathways: In paclitaxel-resistant ovarian cancer cells, this compound inhibits the phosphorylation of key signaling proteins such as AKT, STAT3, ERK, and p38 MAPK.
Comparison with Similar Compounds
Structural Comparison
Tephrosin belongs to the rotenoid family, sharing a core benzopyran structure with:
- Rotenone: Differs by a methoxy group at C-9 and absence of hydroxylation at C-12a .
- Deguelin : Lacks the C-12a hydroxyl group and has a double bond at C-6a/C-12a .
- 12a-Hydroxyrotenone: Contains hydroxylation at C-12a but retains the methoxy group at C-9 .
- Rotenolone: Features a ketone group at C-12 instead of hydroxylation .
Key Structural Features Influencing Bioactivity :
- The C-12a hydroxyl group in this compound enhances solubility and binding affinity to cellular targets compared to non-hydroxylated analogs like deguelin .
- Prenylation at C-6/C-7 in this compound contributes to membrane permeability and target interaction .
Cytotoxicity and Potency
Notable Trends:
- This compound and deguelin show solid tumor selectivity, whereas rotenone is broadly toxic but less specific .
- Hydroxylation at C-12a (this compound, 12a-hydroxyrotenone) correlates with enhanced anticancer activity compared to non-hydroxylated analogs .
Mechanisms of Action
- This compound: Targets receptor tyrosine kinases (FGFR1) and downstream survival pathways (AKT, STAT3) .
- Rotenone: Inhibits mitochondrial Complex I, causing ATP depletion and oxidative stress .
- Deguelin : Suppresses HIF-1α and NF-κB pathways, showing anti-angiogenic effects .
Toxicity Profiles
Biological Activity
Tephrosin is a naturally occurring rotenoid isoflavonoid primarily isolated from the plant Tephrosia vogelii. It has garnered significant attention in the field of cancer research due to its potent biological activities, particularly its anticancer properties. This article aims to provide a detailed overview of the biological activity of this compound, including its mechanisms of action, efficacy in various cancer types, and potential therapeutic applications.
This compound exhibits multiple mechanisms through which it exerts its anticancer effects:
- Induction of Apoptosis : this compound has been shown to induce apoptosis in various cancer cell lines by increasing intracellular reactive oxygen species (ROS) levels. This process is accompanied by mitochondrial membrane potential depolarization and cytochrome c release, leading to the activation of caspases-3 and -9, and PARP cleavage .
- ROS Production : The generation of ROS is crucial for this compound's anticancer activity. Studies indicate that this compound treatment leads to a time- and dose-dependent increase in ROS levels, which correlates with reduced viability of cancer cells . The depletion of intracellular antioxidants such as glutathione (GSH) further supports this mechanism .
- Inhibition of Autophagy : this compound enhances the cytotoxicity of other agents like 2-deoxy-D-glucose (2-DG) by inhibiting autophagy, thereby promoting ATP depletion in cancer cells. This synergistic effect leads to increased apoptosis and reduced cell viability .
- Receptor Internalization : this compound induces the internalization and degradation of epidermal growth factor receptors (EGFR) and ErbB2 in colon cancer cells, which may contribute to its antiproliferative effects .
Efficacy in Cancer Models
This compound's efficacy has been demonstrated across various cancer types through both in vitro and in vivo studies:
- Pancreatic Cancer : In studies involving human pancreatic cancer cell lines (PANC-1 and SW1990), this compound exhibited significant antitumor activity, reducing tumor growth by approximately 60.2% in xenograft models at a dosage of 20 mg/kg . It effectively induced apoptosis and increased ROS levels within the cells.
- Ovarian Cancer : Recent research indicates that this compound can overcome chemoresistance in paclitaxel-resistant ovarian cancer cells (SKOV3-TR). The compound enhances cytotoxicity when combined with paclitaxel by inhibiting critical signaling pathways involved in cell survival .
Study on Pancreatic Cancer
A pivotal study explored the effects of this compound on pancreatic cancer cells, revealing that it significantly suppressed cell viability and induced apoptosis. The study utilized BALB/c nude mice implanted with PANC-1 cells, demonstrating that daily intraperitoneal injections of this compound resulted in substantial tumor size reduction compared to control groups .
Combination Therapy with 2-DG
Another study investigated the combination of this compound with 2-DG, showing enhanced cytotoxic effects against various cancer cell lines. The combination treatment led to accelerated ATP depletion and increased activation of AMPK, further supporting the role of this compound as a potent adjunctive agent in cancer therapy .
Summary Table of Biological Activities
Q & A
Basic Research Questions
Q. What standardized assays are used to evaluate Tephrosin’s cytotoxicity in pancreatic cancer cells, and how should researchers interpret IC₅₀ values?
this compound’s cytotoxicity is typically assessed via MTT assays, which measure mitochondrial activity in cell viability studies. IC₅₀ values (e.g., 41.21 μM in HUVEC vs. 18.86 μM in SW1990 cells ) must be contextualized with cell-specific factors like metabolic rate and membrane permeability. Researchers should include positive controls (e.g., cisplatin) and validate results across multiple cell lines to account for variability .
Q. How can researchers confirm this compound-induced apoptosis in vitro?
Apoptosis is confirmed through complementary methods:
- Nuclear morphology : Hoechst 33258 staining detects chromatin condensation .
- Flow cytometry : Annexin V/PI co-staining quantifies early/late apoptotic populations .
- Caspase activation : Western blotting for cleaved caspase-3/-9 and PARP, coupled with caspase inhibitor studies (e.g., Z-VAD-FMK) to block apoptosis .
- LDH release assays : Validate membrane integrity loss during late apoptosis .
Q. What are the minimum requirements for characterizing this compound’s purity and structural identity in experimental studies?
- Purity : ≥95% purity via HPLC or LC-MS, with batch-specific certificates from suppliers like MedChemExpress .
- Structural validation : NMR and high-resolution mass spectrometry (HRMS) for novel derivatives; literature comparison for known compounds .
Advanced Research Questions
Q. How can conflicting data on this compound’s classification (e.g., rotenoid isoflavonoid vs. carotenoid) be resolved in mechanistic studies?
Discrepancies (e.g., rotenoid isoflavonoid vs. carotenoid ) require:
- Structural re-analysis : Compare spectral data (NMR, MS) with published rotenoid scaffolds .
- Functional assays : Test insecticidal activity (a rotenoid hallmark) to confirm classification .
- Source verification : Validate plant genus (Derris for rotenoids) and extraction protocols .
Q. What experimental strategies validate ROS as the primary mediator of this compound’s anticancer effects?
- ROS scavengers : Pre-treatment with NAC (N-acetylcysteine) to reverse apoptosis and caspase activation .
- Mitochondrial assays : JC-1 staining for membrane potential depolarization and cytochrome c release .
- DNA damage markers : γ-H2AX phosphorylation and comet assays to link ROS to genomic instability .
Q. How should researchers design in vivo studies to balance this compound’s antitumor efficacy and toxicity?
- Model selection : Use immunocompromised mice (e.g., nude mice) with PANC-1 xenografts for human-relevant data .
- Dosing regimen : Optimize via pharmacokinetic studies (e.g., bioavailability, half-life) to minimize off-target effects .
- Toxicity endpoints : Monitor body weight, organ histopathology, and serum biomarkers (e.g., ALT/AST) .
Q. How to reconcile discrepancies in this compound’s selectivity between cancer and normal cells?
Conflicting selectivity profiles (e.g., SW1990 vs. HUVEC ) may arise from:
Properties
IUPAC Name |
(1R,14R)-14-hydroxy-17,18-dimethoxy-7,7-dimethyl-2,8,21-trioxapentacyclo[12.8.0.03,12.04,9.015,20]docosa-3(12),4(9),5,10,15,17,19-heptaen-13-one | |
---|---|---|
Source | PubChem | |
URL | https://pubchem.ncbi.nlm.nih.gov | |
Description | Data deposited in or computed by PubChem | |
InChI |
InChI=1S/C23H22O7/c1-22(2)8-7-12-15(30-22)6-5-13-20(12)29-19-11-28-16-10-18(27-4)17(26-3)9-14(16)23(19,25)21(13)24/h5-10,19,25H,11H2,1-4H3/t19-,23-/m1/s1 | |
Source | PubChem | |
URL | https://pubchem.ncbi.nlm.nih.gov | |
Description | Data deposited in or computed by PubChem | |
InChI Key |
AQBZCCQCDWNNJQ-AUSIDOKSSA-N | |
Source | PubChem | |
URL | https://pubchem.ncbi.nlm.nih.gov | |
Description | Data deposited in or computed by PubChem | |
Canonical SMILES |
CC1(C=CC2=C(O1)C=CC3=C2OC4COC5=CC(=C(C=C5C4(C3=O)O)OC)OC)C | |
Source | PubChem | |
URL | https://pubchem.ncbi.nlm.nih.gov | |
Description | Data deposited in or computed by PubChem | |
Isomeric SMILES |
CC1(C=CC2=C(O1)C=CC3=C2O[C@@H]4COC5=CC(=C(C=C5[C@@]4(C3=O)O)OC)OC)C | |
Source | PubChem | |
URL | https://pubchem.ncbi.nlm.nih.gov | |
Description | Data deposited in or computed by PubChem | |
Molecular Formula |
C23H22O7 | |
Source | PubChem | |
URL | https://pubchem.ncbi.nlm.nih.gov | |
Description | Data deposited in or computed by PubChem | |
DSSTOX Substance ID |
DTXSID20878627 | |
Record name | Tephrosin | |
Source | EPA DSSTox | |
URL | https://comptox.epa.gov/dashboard/DTXSID20878627 | |
Description | DSSTox provides a high quality public chemistry resource for supporting improved predictive toxicology. | |
Molecular Weight |
410.4 g/mol | |
Source | PubChem | |
URL | https://pubchem.ncbi.nlm.nih.gov | |
Description | Data deposited in or computed by PubChem | |
CAS No. |
76-80-2 | |
Record name | Tephrosin | |
Source | CAS Common Chemistry | |
URL | https://commonchemistry.cas.org/detail?cas_rn=76-80-2 | |
Description | CAS Common Chemistry is an open community resource for accessing chemical information. Nearly 500,000 chemical substances from CAS REGISTRY cover areas of community interest, including common and frequently regulated chemicals, and those relevant to high school and undergraduate chemistry classes. This chemical information, curated by our expert scientists, is provided in alignment with our mission as a division of the American Chemical Society. | |
Explanation | The data from CAS Common Chemistry is provided under a CC-BY-NC 4.0 license, unless otherwise stated. | |
Record name | Tephrosin | |
Source | ChemIDplus | |
URL | https://pubchem.ncbi.nlm.nih.gov/substance/?source=chemidplus&sourceid=0000076802 | |
Description | ChemIDplus is a free, web search system that provides access to the structure and nomenclature authority files used for the identification of chemical substances cited in National Library of Medicine (NLM) databases, including the TOXNET system. | |
Record name | Tephrosin | |
Source | EPA DSSTox | |
URL | https://comptox.epa.gov/dashboard/DTXSID20878627 | |
Description | DSSTox provides a high quality public chemistry resource for supporting improved predictive toxicology. | |
Record name | 76-80-2 | |
Source | European Chemicals Agency (ECHA) | |
URL | https://echa.europa.eu/information-on-chemicals | |
Description | The European Chemicals Agency (ECHA) is an agency of the European Union which is the driving force among regulatory authorities in implementing the EU's groundbreaking chemicals legislation for the benefit of human health and the environment as well as for innovation and competitiveness. | |
Explanation | Use of the information, documents and data from the ECHA website is subject to the terms and conditions of this Legal Notice, and subject to other binding limitations provided for under applicable law, the information, documents and data made available on the ECHA website may be reproduced, distributed and/or used, totally or in part, for non-commercial purposes provided that ECHA is acknowledged as the source: "Source: European Chemicals Agency, http://echa.europa.eu/". Such acknowledgement must be included in each copy of the material. ECHA permits and encourages organisations and individuals to create links to the ECHA website under the following cumulative conditions: Links can only be made to webpages that provide a link to the Legal Notice page. | |
Record name | TEPHROSIN | |
Source | FDA Global Substance Registration System (GSRS) | |
URL | https://gsrs.ncats.nih.gov/ginas/app/beta/substances/9C081V83CC | |
Description | The FDA Global Substance Registration System (GSRS) enables the efficient and accurate exchange of information on what substances are in regulated products. Instead of relying on names, which vary across regulatory domains, countries, and regions, the GSRS knowledge base makes it possible for substances to be defined by standardized, scientific descriptions. | |
Explanation | Unless otherwise noted, the contents of the FDA website (www.fda.gov), both text and graphics, are not copyrighted. They are in the public domain and may be republished, reprinted and otherwise used freely by anyone without the need to obtain permission from FDA. Credit to the U.S. Food and Drug Administration as the source is appreciated but not required. | |
Retrosynthesis Analysis
AI-Powered Synthesis Planning: Our tool employs the Template_relevance Pistachio, Template_relevance Bkms_metabolic, Template_relevance Pistachio_ringbreaker, Template_relevance Reaxys, Template_relevance Reaxys_biocatalysis model, leveraging a vast database of chemical reactions to predict feasible synthetic routes.
One-Step Synthesis Focus: Specifically designed for one-step synthesis, it provides concise and direct routes for your target compounds, streamlining the synthesis process.
Accurate Predictions: Utilizing the extensive PISTACHIO, BKMS_METABOLIC, PISTACHIO_RINGBREAKER, REAXYS, REAXYS_BIOCATALYSIS database, our tool offers high-accuracy predictions, reflecting the latest in chemical research and data.
Strategy Settings
Precursor scoring | Relevance Heuristic |
---|---|
Min. plausibility | 0.01 |
Model | Template_relevance |
Template Set | Pistachio/Bkms_metabolic/Pistachio_ringbreaker/Reaxys/Reaxys_biocatalysis |
Top-N result to add to graph | 6 |
Feasible Synthetic Routes
Disclaimer and Information on In-Vitro Research Products
Please be aware that all articles and product information presented on BenchChem are intended solely for informational purposes. The products available for purchase on BenchChem are specifically designed for in-vitro studies, which are conducted outside of living organisms. In-vitro studies, derived from the Latin term "in glass," involve experiments performed in controlled laboratory settings using cells or tissues. It is important to note that these products are not categorized as medicines or drugs, and they have not received approval from the FDA for the prevention, treatment, or cure of any medical condition, ailment, or disease. We must emphasize that any form of bodily introduction of these products into humans or animals is strictly prohibited by law. It is essential to adhere to these guidelines to ensure compliance with legal and ethical standards in research and experimentation.