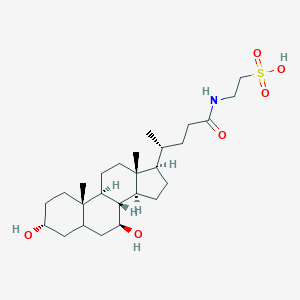
Tauroursodeoxycholic acid
Overview
Description
Tauroursodeoxycholic acid (TUDCA) is a taurine-conjugated derivative of ursodeoxycholic acid (UDCA), a hydrophilic bile acid with established therapeutic roles in hepatobiliary disorders such as primary biliary cholangitis . TUDCA exhibits cytoprotective properties, primarily by reducing endoplasmic reticulum (ER) stress, stabilizing the unfolded protein response (UPR), and modulating apoptosis . Mechanistically, TUDCA suppresses pro-apoptotic markers like CHOP and ATF4, enhances insulin sensitivity, and regulates bile acid metabolism and gut microbiota .
Preparation Methods
Mixed Anhydride Synthesis via Ursodeoxycholic Acid
Reaction Mechanism and Reagent Stoichiometry
The EP2137206A1 patent details a scalable method using ursodeoxycholic acid (UDCA) as the primary substrate . The synthesis involves:
-
Mixed Anhydride Formation : UDCA reacts with ethyl chloroformate in acetone at sub-zero temperatures (<10°C) in the presence of triethylamine (TEA) as a tertiary base. This generates a reactive mixed anhydride intermediate, facilitating subsequent nucleophilic attack by taurine’s amino group .
-
Sodium Taurinate Coupling : An aqueous solution of sodium taurinate (prepared from 95.6 kg taurine) is introduced to the anhydride, yielding the sodium salt of TUDCA .
-
Acidification and Purification : Hydrochloric acid (32–36% concentration) precipitates impurities (taurine, sodium chloride), while TUDCA remains soluble. Subsequent ethanol resuspension and solvent-induced precipitation achieve crude TUDCA with ≤0.3% impurities .
Key Parameters :
-
Temperature Control : Maintaining ≤10°C during anhydride formation prevents side reactions.
-
Acid Equivalents : 1.0–1.1 equivalents of HCl optimize impurity precipitation without degrading TUDCA .
-
Solvent Ratios : Acetone:water ratios of 3:1 enhance mixed anhydride stability.
Table 1: Industrial-Scale Synthesis Parameters (EP2137206A1)
Parameter | Value | Impact on Yield/Purity |
---|---|---|
UDCA Input | 250 kg | Baseline for 95% yield |
Ethyl Chloroformate | 69.4 kg | 1:1 molar ratio with UDCA |
TEA | 64.9 kg | Neutralizes HCl, maintains pH 8–9 |
Precipitation Time | 20–60 min | Maximizes impurity removal |
Final Purity | ≤0.3% impurities | Meets pharmacopeial standards |
Alternative Synthesis from Chenodeoxycholic Acid
Oxidation-Reduction Pathway
The CN104844677A patent proposes a cost-effective route using chenodeoxycholic acid (CDCA) as the starting material . This method diverges from UDCA-based synthesis by incorporating:
-
Active Ester Formation : CDCA reacts with N-hydroxysuccinimide (NHS) or hydroxybenzotriazole (HOBt) under condensing agents (e.g., dicyclohexylcarbodiimide), forming an activated ester intermediate .
-
Taurochenodeoxycholic Acid Synthesis : The active ester couples with sodium taurate in aqueous triethylamine, producing taurochenodeoxycholic acid (TCDCA) .
-
Epimerization to TUDCA : TCDCA undergoes oxidation (e.g., using Jones reagent) at the 7α-hydroxyl group, followed by stereoselective reduction (e.g., NaBH4) to yield TUDCA .
Advantages Over UDCA Routes :
-
Raw Material Cost : CDCA is ≈30% cheaper than UDCA in bulk quantities .
-
Reduced Byproducts : Epimerization minimizes 7-keto intermediate degradation.
Table 2: Comparative Analysis of CDCA vs. UDCA Routes
Metric | CDCA Route | UDCA Route |
---|---|---|
Starting Material Cost | $120/kg | $350/kg |
Reaction Steps | 4 | 3 |
Overall Yield | 68–72% | 85–90% |
Purity Post-Crystallization | 98.5% | 99.2% |
Critical Evaluation of Purification Techniques
Solvent-Induced Precipitation
Both patents emphasize solvent selection for impurity removal:
-
Ethanol-Water Systems : Remove unreacted taurine and inorganic salts via differential solubility .
-
Acetone Anti-Solvent : Precipitates TUDCA while retaining chlorides in the mother liquor .
Chromatographic Refinement
Challenges and Optimization Strategies
Byproduct Management
-
Triethylamine Hydrochloride : Generated during mixed anhydride synthesis, removed via centrifugation and acetone washes .
-
7-Keto Intermediates : In CDCA routes, over-oxidation risks are mitigated by strict temperature control (<5°C during Jones reagent addition) .
Scalability Considerations
Scientific Research Applications
Neurodegenerative Diseases
TUDCA has shown promise in treating several neurodegenerative diseases, including Alzheimer’s disease, Parkinson’s disease, and amyotrophic lateral sclerosis (ALS). Its mechanisms of action primarily involve anti-apoptotic effects, reduction of endoplasmic reticulum (ER) stress, and modulation of mitochondrial pathways.
Key Findings:
- Alzheimer’s Disease: TUDCA reduces Amyloid-β protein deposition and protects neuronal synapses, showing potential for preventing cognitive decline .
- Amyotrophic Lateral Sclerosis: A study demonstrated that TUDCA significantly slowed the progression of ALS symptoms compared to placebo, with an 87% response rate in treated patients .
- Parkinson’s Disease: Research indicates that TUDCA may protect against neuronal death and improve motor function in animal models .
Metabolic Disorders
TUDCA has been investigated for its effects on metabolic disorders, particularly those involving insulin resistance and obesity-related complications.
Key Findings:
- Diabetes: TUDCA administration improved glucose tolerance and reduced markers of ER stress in diabetic mouse models .
- Obesity: In studies involving obese mice, TUDCA treatment led to improved cardiac function and reduced blood pressure, suggesting a protective role against obesity-induced cardiac dysfunction .
Liver Diseases
Originally approved for treating cholestatic liver diseases, TUDCA's hepatoprotective properties extend beyond traditional uses.
Key Findings:
- Cholestatic Liver Diseases: TUDCA is effective in managing conditions like primary biliary cholangitis and primary sclerosing cholangitis by reducing liver inflammation and fibrosis .
- Hepatocellular Carcinoma: Research indicates that TUDCA can mitigate ER stress in liver cells, potentially reducing tumor burden in experimental models .
Cardiovascular Applications
Emerging research suggests that TUDCA may have beneficial effects on cardiovascular health.
Key Findings:
- Heart Failure: Studies indicate that TUDCA can alleviate myocardial contractile dysfunction associated with obesity by reducing ER stress and improving calcium handling in cardiomyocytes .
- Cardiac Fibrosis: In models of pressure overload, TUDCA reduced myocardial fibrosis and improved cardiac function, highlighting its potential as a therapeutic agent for heart diseases .
Data Table: Summary of Clinical Applications of TUDCA
Case Study 1: Amyotrophic Lateral Sclerosis
In a multicenter randomized controlled trial involving ALS patients treated with TUDCA (1 g twice daily), results showed a significant improvement in functional ratings compared to placebo over a 54-week period. The study highlighted the potential for prolonged survival among those receiving higher doses of TUDCA .
Case Study 2: Diabetic Nephropathy
A study on diabetic db/db mice treated with TUDCA demonstrated significant reductions in renal histopathology and albuminuria after eight weeks. These findings suggest that TUDCA may protect against renal injury through the inhibition of ER stress pathways .
Mechanism of Action
Tauroursodeoxycholic acid exerts its effects through several mechanisms:
Anti-apoptotic Effects: It inhibits the apoptotic cascade by reducing oxidative stress, protecting mitochondria, and stabilizing proteins
Endoplasmic Reticulum Stress Response: this compound alleviates endoplasmic reticulum stress and stabilizes the unfolded protein response, acting as a chemical chaperone.
Anti-inflammatory Action: It reduces inflammation by modulating immune responses and decreasing pro-inflammatory cytokine production.
Cholesterol Regulation: this compound reduces cholesterol absorption in the small intestine, thereby lowering dietary cholesterol intake.
Comparison with Similar Compounds
Tauroursodeoxycholic Acid vs. Ursodeoxycholic Acid (UDCA)
- Chemical Properties : TUDCA is the taurine conjugate of UDCA, increasing its hydrophilicity and bioavailability compared to UDCA .
- Efficacy in Liver Diseases: In cirrhotic patients awaiting liver transplantation, TUDCA improved cholestasis markers (e.g., γ-glutamyl transpeptidase) and clinical stability, reducing hospital stays . UDCA, while effective in primary biliary cholangitis, shows inconsistent results in post-transplant settings.
- Neuroprotection :
- Metabolic Effects: In HCV-related chronic hepatitis, TUDCA (750 mg/day) reduced aminotransferase levels more effectively than placebo, a benefit less documented for UDCA .
Table 1: Comparative Efficacy of TUDCA and UDCA in Clinical Studies
TUDCA vs. Taurocholic Acid
- Diagnostic Utility: In metabolic disorder diagnostics, taurocholic acid showed higher accuracy (64.29%) than TUDCA (50%) in plasma metabolite panels .
- Pathological Roles: Both bile acids are elevated in peritoneal and pleural malignancies, but TUDCA uniquely correlates with improved metabolic health (e.g., higher fecal levels in non-diabetic obese individuals) .
Table 2: TUDCA vs. Taurocholic Acid in Disease Contexts
TUDCA vs. Other Bile Acids (e.g., Deoxycholic Acid)
- Physicochemical Profile :
- Clinical Applications: TUDCA prevents bile duct stone recurrence post-surgery (6.7% vs. 26.7% in controls) and post-ERCP (10% vs.
Table 3: TUDCA’s Superiority in Preventing Bile Duct Stone Recurrence
Intervention | Recurrence Rate (TUDCA) | Recurrence Rate (Control) | p-value | Reference |
---|---|---|---|---|
Post-surgical prophylaxis | 6.7% | 26.7% | 0.011 | |
Post-ERCP prophylaxis | 10% | 25% | <0.05 |
Key Research Findings and Clinical Implications
- Neurodegenerative Diseases: TUDCA’s ER stress modulation improves motor neuron survival in ALS models and reduces apoptosis in subarachnoid hemorrhage .
- Diabetes and Obesity : TUDCA enhances insulin receptor binding, reduces hepatic lipid accumulation, and increases beneficial gut bacteria (e.g., Allobaculum) .
Q & A
Basic Research Questions
Q. What are the standard protocols for synthesizing TUDCA, and how is purity validated?
TUDCA synthesis typically involves condensation reactions between ursodeoxycholic acid (UDCA) and taurine, followed by purification steps. For example, UDCA is activated with propyl chloroformate in the presence of triethylamine, then reacted with taurine sodium salt. Post-reaction, pH adjustment and solvent extraction are used to isolate TUDCA . Purity validation employs high-performance liquid chromatography (HPLC) and nuclear magnetic resonance (NMR), with deuterated standards (e.g., TUDCA-d4) ensuring accurate quantification in mass spectrometry (LC-MS/GC-MS) .
Q. How do researchers select between in vitro and in vivo models for studying TUDCA’s neuroprotective effects?
In vitro models (e.g., neuronal cell lines exposed to oxidative stress) are used for mechanistic studies, such as measuring ER stress markers (e.g., CHOP, XBP1 splicing). In vivo models (e.g., ALS mice or diabetic retinopathy rodents) validate therapeutic efficacy through behavioral tests and histopathology. Cross-model consistency is ensured by replicating key biomarkers (e.g., caspase-3 activation) across both systems .
Q. What analytical techniques are critical for assessing TUDCA stability and bioavailability?
LC-MS with deuterated internal standards (e.g., TUDCA-d4) ensures precise quantification in biological matrices. Stability studies use accelerated degradation tests under varying pH and temperature conditions, while bioavailability is assessed via pharmacokinetic (PK) profiling in serum/plasma samples .
Q. How are clinical trials for TUDCA in neurodegenerative diseases designed?
Phase II/III trials for conditions like ALS prioritize double-blinded, placebo-controlled designs with endpoints such as ALSFRS-R scores. Systematic literature reviews and ClinicalTrials.gov data inform inclusion criteria and safety monitoring protocols .
Advanced Research Questions
Q. How can discrepancies in TUDCA synthesis yields (e.g., 67% vs. 87.9%) be resolved?
Design of Experiments (DOE) methodologies optimize reaction parameters (temperature, catalyst concentration). For instance, lower temperatures (-10°C) during mixed anhydride formation reduce side reactions, improving yields . Reaction tracking via thin-layer chromatography (TLC) identifies intermediate bottlenecks .
Q. What strategies reconcile contradictory results between preclinical and clinical TUDCA studies?
Meta-analyses adjust for interspecies differences (e.g., bile acid metabolism in rodents vs. humans). Pharmacodynamic (PD) biomarkers (e.g., serum TUDCA levels) are correlated with clinical outcomes to identify subpopulations with optimal response .
Q. How can multi-omics approaches elucidate TUDCA’s mechanism in metabolic diseases?
Transcriptomics (RNA-seq) and proteomics (LC-MS/MS) identify pathways like UPR (Unfolded Protein Response) in diabetic models. Bioinformatics tools (e.g., STRING) map interactions between TUDCA-modulated proteins (e.g., BIP/GRP78) and disease-associated networks .
Q. What in silico models predict TUDCA’s interactions with mitochondrial targets?
Molecular docking simulations (e.g., AutoDock Vina) model TUDCA binding to anti-apoptotic proteins (e.g., Bcl-2). Molecular dynamics (MD) simulations assess stability of these complexes under physiological conditions .
Q. How do researchers validate TUDCA’s role in modulating matrix metalloproteinases (MMPs)?
Zymography and fluorescence-based assays quantify MMP activity in cancer cell lines (e.g., MDA-MB-231). siRNA knockdown of MMP genes paired with TUDCA treatment isolates specific pathway contributions .
Q. What advanced delivery systems enhance TUDCA’s bioavailability in the CNS?
Electrodeposition techniques create polymer-based nanoparticles (e.g., PEDOT-TUDCA) for controlled release. In vitro blood-brain barrier (BBB) models (e.g., hCMEC/D3 cells) assess permeability improvements .
Q. Methodological Considerations
Q. How are endoplasmic reticulum (ER) stress assays standardized in TUDCA studies?
Western blotting for ER markers (ATF6, PERK) and qPCR for XBP1 splicing are calibrated using positive controls (e.g., tunicamycin). Dose-response curves establish TUDCA’s efficacy in reducing stress across cell types .
Q. What statistical methods address heterogeneity in TUDCA clinical trial data?
Q. How is mitochondrial function assessed in TUDCA-treated models?
Seahorse XF analyzers measure oxygen consumption rates (OCR) to evaluate mitochondrial respiration. Flow cytometry with JC-1 dye quantifies membrane potential changes in apoptotic cells .
Q. What comparative studies distinguish TUDCA from other bile acids (e.g., UDCA)?
In vitro assays (e.g., cell viability under ER stress) and molecular docking compare binding affinities to targets like the TGR5 receptor. In vivo studies use bile acid transporter knockout mice to isolate TUDCA-specific effects .
Properties
Key on ui mechanism of action |
About 90% of gallstones are formed by cholesterol, which may be caused by altered gut microbiota from a high-fat diet and other factors. The gut microbiota regulates bile acid metabolism; thus, altered composition in gut microbiota may significantly change the bile acid pool and alter cholesterol secretion. While the exact mechanism of action of tauroursodeoxycholic acid in reducing and preventing gallstone formation is unclear, tauroursodeoxycholic acid may achieve this effect in a number of ways. A recent mouse study suggests that tauroursodeoxycholic acid inhibits intestinal cholesterol absorption and lowers liver cholesterol levels by upregulating the bile acid excretion from the liver to the gallbladder. Tauroursodeoxycholic acid lowers the bile cholesterol saturation in the gallbladder, thereby increasing the solubility of cholesterol in bile. It can also maintain a specific gut microbiota composition to promote the synthesis of bile acids and reduce liver inflammation caused by the lipopolysaccharide in the blood. Ultimately, tauroursodeoxycholic acid enhances the synthesis of bile acids in the liver and reduces cholesterol in the serum and liver. Tauroursodeoxycholic acid inhibits cell apoptosis by disrupting the mitochondrial pathway of cell death. It works by inhibiting oxygen-radical production, ameliorating endoplasmic reticulum (ER) stress, and stabilizing the unfolded protein response. Other anti-apoptotic processes mediated by tauroursodeoxycholic acid include cytochrome c release, caspase activation, DNA and nuclear fragmentation, and inhibition of p53 transactivation. It is believed that tauroursodeoxycholic acid works on multiple cellular targets to inhibit apoptosis and upregulate survival pathways. |
---|---|
CAS No. |
14605-22-2 |
Molecular Formula |
C26H45NO6S |
Molecular Weight |
499.7 g/mol |
IUPAC Name |
2-[[(4R)-4-[(3R,7S,8R,9S,10S,13R,14S,17R)-3,7-dihydroxy-10,13-dimethyl-2,3,4,5,6,7,8,9,11,12,14,15,16,17-tetradecahydro-1H-cyclopenta[a]phenanthren-17-yl]pentanoyl]amino]ethanesulfonic acid |
InChI |
InChI=1S/C26H45NO6S/c1-16(4-7-23(30)27-12-13-34(31,32)33)19-5-6-20-24-21(9-11-26(19,20)3)25(2)10-8-18(28)14-17(25)15-22(24)29/h16-22,24,28-29H,4-15H2,1-3H3,(H,27,30)(H,31,32,33)/t16-,17?,18-,19-,20+,21+,22+,24+,25+,26-/m1/s1 |
InChI Key |
BHTRKEVKTKCXOH-VSHSPWMTSA-N |
SMILES |
CC(CCC(=O)NCCS(=O)(=O)O)C1CCC2C1(CCC3C2C(CC4C3(CCC(C4)O)C)O)C |
Isomeric SMILES |
C[C@H](CCC(=O)NCCS(=O)(=O)O)[C@H]1CC[C@@H]2[C@@]1(CC[C@H]3[C@H]2[C@H](CC4[C@@]3(CC[C@H](C4)O)C)O)C |
Canonical SMILES |
CC(CCC(=O)NCCS(=O)(=O)O)C1CCC2C1(CCC3C2C(CC4C3(CCC(C4)O)C)O)C |
Key on ui other cas no. |
14605-22-2 |
physical_description |
Solid |
Synonyms |
tauroursodeoxycholate tauroursodeoxycholic acid tauroursodeoxycholic acid, (3alpha,5alpha,7alpha)-isomer tauroursodeoxycholic acid, monosodium salt, (3alpha,5beta,7alpha)-isomer tauroursodeoxycholic acid, monosodium salt, (3alpha,7alpha)-isomer TUDCA |
Origin of Product |
United States |
Retrosynthesis Analysis
AI-Powered Synthesis Planning: Our tool employs the Template_relevance Pistachio, Template_relevance Bkms_metabolic, Template_relevance Pistachio_ringbreaker, Template_relevance Reaxys, Template_relevance Reaxys_biocatalysis model, leveraging a vast database of chemical reactions to predict feasible synthetic routes.
One-Step Synthesis Focus: Specifically designed for one-step synthesis, it provides concise and direct routes for your target compounds, streamlining the synthesis process.
Accurate Predictions: Utilizing the extensive PISTACHIO, BKMS_METABOLIC, PISTACHIO_RINGBREAKER, REAXYS, REAXYS_BIOCATALYSIS database, our tool offers high-accuracy predictions, reflecting the latest in chemical research and data.
Strategy Settings
Precursor scoring | Relevance Heuristic |
---|---|
Min. plausibility | 0.01 |
Model | Template_relevance |
Template Set | Pistachio/Bkms_metabolic/Pistachio_ringbreaker/Reaxys/Reaxys_biocatalysis |
Top-N result to add to graph | 6 |
Feasible Synthetic Routes
Disclaimer and Information on In-Vitro Research Products
Please be aware that all articles and product information presented on BenchChem are intended solely for informational purposes. The products available for purchase on BenchChem are specifically designed for in-vitro studies, which are conducted outside of living organisms. In-vitro studies, derived from the Latin term "in glass," involve experiments performed in controlled laboratory settings using cells or tissues. It is important to note that these products are not categorized as medicines or drugs, and they have not received approval from the FDA for the prevention, treatment, or cure of any medical condition, ailment, or disease. We must emphasize that any form of bodily introduction of these products into humans or animals is strictly prohibited by law. It is essential to adhere to these guidelines to ensure compliance with legal and ethical standards in research and experimentation.