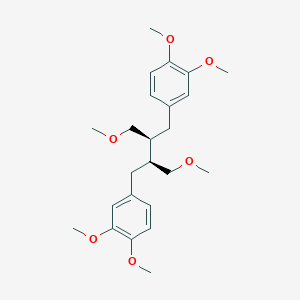
Phyllanthin
Overview
Description
Phyllanthin is a bioactive lignan predominantly isolated from Phyllanthus species, including P. amarus, P. niruri, and P. tenellus. It is recognized for its hepatoprotective, antioxidant, anticancer, and metabolic regulatory properties . Structurally, this compound (C${24}$H${30}$O$_6$) is characterized by a dibenzylbutane skeleton, with a molecular weight of 418 g/mol, as inferred from its sodium adduct mass ([M+Na]$^+$ = 441 m/z) . It is a key marker compound for standardizing Phyllanthus extracts due to its therapeutic significance and species-specific distribution .
This compound's pharmacological profile includes:
- Antioxidant activity: IC$_{50}$ of 7.4 µmol/mL in scavenging free radicals .
- Anticancer synergy: Enhances doxorubicin efficacy by suppressing autophagy and apoptosis resistance in breast cancer cells .
- Metabolic regulation: Mitigates high-fat-diet-induced obesity, insulin resistance, and hepatic lipid accumulation in mice .
- Radioprotection: Reduces radiation-induced chromosomal aberrations via free radical scavenging .
Preparation Methods
Conventional Solvent-Based Extraction Methods
Soxhlet Extraction and Solvent Selection
Soxhlet extraction remains a cornerstone for preliminary phyllanthin isolation due to its simplicity and reproducibility. Studies using P. niruri aerial parts demonstrated that nonpolar solvents like hexane outperform polar solvents in lignan selectivity. For instance, hexane-based Soxhlet extraction yielded 0.82% (w/w) extract with a this compound content of 36.2 ± 2.6 mg/g, whereas methanol produced only 3.1 mg/g despite a higher extract yield (3.6% w/w) . This disparity underscores the importance of solvent polarity in lignan solubility, as this compound’s lipophilic nature favors nonpolar environments.
Table 1: Soxhlet Extraction Efficiency Across Solvents
Solvent | Extract Yield (% w/w) | This compound Content (mg/g) |
---|---|---|
Hexane | 0.82 | 36.2 ± 2.6 |
CH₂Cl₂ | 1.12 | 11.7 ± 1.68 |
Acetone | 3.40 | 11.7 ± 1.10 |
Methanol | 3.60 | 3.1 |
Percolation and Maceration Techniques
Percolation, a dynamic extraction method, enhances mass transfer by continuously passing solvent through powdered plant material. A patent by detailed a optimized percolation process for P. amarus leaves using binary solvent systems (e.g., n-hexane:ethyl acetate, 9:1 to 1:1) at elevated temperatures (up to 80°C). This approach achieved a this compound-rich residue (25.9 mg/g) after subsequent fat precipitation and silica gel chromatography. Comparatively, maceration with 96% ethanol yielded high flavonoid content but suboptimal lignan recovery, highlighting the need for solvent-system customization based on target compounds .
Advanced Extraction Methodologies
Microwave-Assisted Extraction (MAE)
Microwave technology leverages dielectric heating to disrupt plant cell walls, accelerating solvent penetration. Using 80% methanol and 50°C irradiation, MAE produced an 8.13% (w/w) extract with 21.2 ± 1.30 mg/g this compound, surpassing conventional methanol extraction by nearly sevenfold . The method’s efficiency stems from rapid energy transfer, which reduces thermal degradation of heat-sensitive lignans.
Enzyme-Assisted Extraction (EAE)
Enzymatic pre-treatment with cellulase (9 U/g) and protease (4 U/g) hydrolyzes polysaccharide-protein networks in P. niruri cell walls, liberating bound lignans. This sequential enzymatic digestion at 50°C for 84 hours yielded 13.92% (w/w) extract with 85.87 mg/g total lignans, including 25.9 mg/g this compound . EAE’s mild conditions preserve this compound stability while avoiding toxic solvent residues, making it ideal for pharmaceutical applications.
Table 2: Nonconventional vs. Conventional Extraction Outcomes
Method | Extract Yield (% w/w) | This compound Content (mg/g) | Total Lignans (mg/g) |
---|---|---|---|
Soxhlet (Hexane) | 0.82 | 36.2 | 48.5 |
MAE (80% Methanol) | 8.13 | 21.2 | 63.1 |
EAE (Cellulase/Protease) | 13.92 | 25.9 | 85.9 |
Alkaline Digestion | 3.10 | 22.3 | 58.4 |
Alkaline Digestion for Lignan Enrichment
Alkaline digestion with 30% potassium hydroxide selectively hydrolyzes esterified lignans from P. niruri, yielding a purified extract (3.1% w/w) with 22.34 ± 0.13 mg/g this compound . This method exploits the base stability of lignans to eliminate phenolic impurities, though it requires stringent pH control to prevent degradation.
Patented Isolation Processes
A German patent (DE10014674B4) outlines a multi-stage protocol for P. amarus:
-
Pulverization : Dried leaves ground to ≤500 µm.
-
Maceratioп : Powder mixed with calcium carbonate (19:1 to 7:3 ratio) to neutralize acidic interferents.
-
Percolation : n-Hexane:ethyl acetate (9:1) at 80°C for 24 hours.
-
Fat Removal : n-Hexane-methanol (2:1) precipitation.
-
Chromatography : Silica gel column with gradient elution (hexane → ethyl acetate).
-
Crystallization : n-Hexane recrystallization yields 98% pure this compound .
Solvent Optimization and Factorial Design
Factorial analyses reveal ethanol concentration as the dominant factor in this compound recovery. While 96% ethanol maximizes flavonoid extraction via maceration or percolation, 50% ethanol enhances phenolic content, indirectly stabilizing lignans during isolation . Such statistical optimization underscores the need for solvent-tailored protocols aligned with target metabolite profiles.
Chemical Reactions Analysis
Phyllanthin undergoes various chemical reactions, including:
Oxidation: this compound can be oxidized to form different oxidized derivatives. Common oxidizing agents include hydrogen peroxide and potassium permanganate.
Reduction: Reduction of this compound can be achieved using reducing agents such as sodium borohydride.
Substitution: this compound can undergo substitution reactions, particularly in the presence of nucleophiles. Common reagents include halogens and alkylating agents.
The major products formed from these reactions depend on the specific conditions and reagents used.
Scientific Research Applications
Pharmacological Properties
Phyllanthin exhibits a range of pharmacological activities, including hepatoprotective, immunosuppressive, anti-inflammatory, and anticancer effects. The following table summarizes key findings related to its applications:
Hepatoprotective Effects
A study on this compound's hepatoprotective effects demonstrated that it significantly reduced liver enzyme levels in animal models of liver injury induced by toxic substances. The compound was shown to enhance antioxidant defenses and mitigate oxidative stress in liver tissues.
Immunosuppressive Effects
Research investigating this compound's immunosuppressive properties revealed that it effectively inhibited the proliferation of T and B lymphocytes in mice models. The study indicated that this compound could serve as a candidate for developing immunosuppressive therapies, particularly for autoimmune diseases or transplant rejection scenarios .
Anti-inflammatory Mechanisms
In a model of high-fat diet-induced obesity, this compound supplementation resulted in decreased levels of pro-inflammatory cytokines and improved lipid profiles. The compound was associated with reduced adipose tissue inflammation and enhanced glucose metabolism, suggesting its utility in managing metabolic syndrome .
Anticancer Activity
This compound has shown promise in cancer research, particularly against leukemia cells. In vitro studies indicated that this compound inhibited the growth of MOLT-4 leukemic cells by inducing apoptosis through the activation of reactive oxygen species and modulation of key signaling pathways involved in cell survival .
Mechanism of Action
Phyllanthin exerts its effects through various molecular targets and pathways:
Antioxidant Activity: This compound increases the levels of glutathione and other antioxidant enzymes, reducing oxidative stress.
Anti-inflammatory Effects: It inhibits the activity of pro-inflammatory cytokines and enzymes such as cyclooxygenase-2 (COX-2) and nuclear factor kappa B (NF-κB).
Hepatoprotective Action: This compound reduces liver triglyceride accumulation and improves liver function by modulating lipid metabolism and reducing inflammation.
Comparison with Similar Compounds
Phyllanthin shares structural and functional similarities with other lignans in Phyllanthus species, such as hypothis compound, niranthin, phyltetralin, and nirtetralin. Below is a detailed comparison:
Hypothis compound
- Structure : Molecular formula C${24}$H${30}$O$_7$ (MW 430 g/mol; [M+Na]$^+$ = 453 m/z) .
- Pharmacological Activities :
- Concentration : Typically 30–60% lower than this compound in P. amarus extracts (e.g., 95.37 µg/mL vs. 162.69 µg/mL) .
- Distribution : Co-occurs with this compound in P. amarus and P. niruri but absent in P. acuminatus .
Niranthin
- Structure : Molecular formula C${24}$H${30}$O$_7$ (MW 432 g/mol; [M+Na]$^+$ = 455 m/z) .
- Pharmacological Activities :
- Concentration : Lower than this compound (e.g., 102.97 µg/mL vs. 162.69 µg/mL in P. amarus) .
- Distribution : Found in P. amarus, P. urinaria, and P. virgatus but absent in P. tenellus .
Phyltetralin
- Structure : Molecular formula C${23}$H${28}$O$_6$ (MW 416 g/mol; [M+Na]$^+$ = 439 m/z) .
- Pharmacological Activities: Limited data; primarily identified via LC-MS in P. amarus and P. tenellus .
- Concentration : Moderate levels (e.g., 145.83 µg/mL in P. amarus) .
- Distribution : Absent in P. acuminatus and P. maderaspatensis .
Other Compounds
- Geraniin and Corilagin : Tannins with potent antioxidant activity but distinct from lignans in structure and mechanism .
- Ellagic Acid : Highest concentration in P. amarus (218.06 µg/mL) but lacks lignan-specific bioactivity .
Comparative Data Tables
Table 1: Structural and Pharmacological Comparison
Table 2: Species-Specific Distribution
Compound | P. amarus | P. niruri | P. tenellus | P. acuminatus |
---|---|---|---|---|
This compound | Yes | Yes | Yes | No |
Hypothis compound | Yes | Yes | Yes | No |
Niranthin | Yes | Yes | No | No |
Key Research Findings
Anticancer Synergy : Hypothis compound and this compound enhance doxorubicin's efficacy in resistant breast cancer cells via SIRT1/Akt pathway modulation. However, hypothis compound promotes autophagy, while this compound inhibits it .
Species-Specific Biosynthesis : P. amarus shoot cultures yield the highest lignan content (~10 mg/g DW) under optimized growth conditions .
Extraction Challenges : this compound and hypothis compound require advanced techniques (e.g., UPLC-ESI-MS) for differentiation due to similar UV spectra .
Biological Activity
Phyllanthin, a lignan compound predominantly found in various species of the Phyllanthus genus, has garnered attention for its diverse biological activities. This article explores the pharmacological effects, mechanisms of action, and potential therapeutic applications of this compound, supported by recent research findings and case studies.
Overview of this compound
This compound is primarily extracted from Phyllanthus amarus and Phyllanthus niruri. It is known for its antioxidant , anti-inflammatory , hepatoprotective , and anticancer properties. This compound plays a significant role in traditional medicine and is being investigated for its potential in modern therapeutic applications.
Pharmacological Activities
This compound exhibits a wide range of biological activities, as summarized in Table 1 below:
Activity | Description |
---|---|
Antioxidant | Scavenges free radicals, reducing oxidative stress in cells. |
Anti-inflammatory | Inhibits inflammatory mediators and pathways, alleviating conditions like arthritis. |
Hepatoprotective | Protects liver cells from damage due to toxins and diseases. |
Anticancer | Induces apoptosis in cancer cells and inhibits tumor growth. |
Antimicrobial | Exhibits activity against various pathogens, including bacteria and viruses. |
Immunomodulatory | Modulates immune responses, enhancing or suppressing activity as needed. |
The biological activity of this compound is mediated through several mechanisms:
- Inhibition of Inflammatory Pathways : this compound has been shown to inhibit the NF-κB pathway, which is crucial in the regulation of inflammatory responses .
- Induction of Apoptosis : It triggers apoptotic pathways in cancer cells by upregulating pro-apoptotic proteins and downregulating anti-apoptotic factors .
- Antioxidant Activity : this compound enhances the expression of antioxidant enzymes, thereby protecting cells from oxidative damage .
Case Studies
-
Hepatoprotective Effects :
A study demonstrated that this compound significantly reduced liver enzyme levels in rats exposed to hepatotoxic agents, suggesting its protective role against liver damage . -
Anticancer Properties :
In vitro studies showed that this compound inhibited the proliferation of various cancer cell lines, including MCF-7 (breast cancer) and A549 (lung cancer) cells. The compound was found to enhance the efficacy of doxorubicin (DOX), a common chemotherapeutic agent, by promoting apoptosis in resistant cancer cells . -
Antimicrobial Activity :
Research indicated that this compound-containing formulations exhibited significant antimicrobial activity against Staphylococcus aureus, highlighting its potential use in treating infections .
Pharmacokinetics and Bioavailability
Pharmacokinetic studies have shown that this compound is well absorbed when administered orally, with peak plasma concentrations occurring within a few hours post-administration. However, its bioavailability can be affected by factors such as formulation and co-administration with other compounds .
Q & A
Basic Research Questions
Q. What are the standard methodologies for extracting Phyllanthin from plant sources, and how do they differ in efficiency?
- Methodological Answer : Common techniques include maceration, Soxhlet extraction, and supercritical fluid extraction (SFE). SFE using CO₂ with methanol as a modifier (e.g., 5–15% v/v) achieves higher yields (up to 2.1 mg/g) due to improved solubility and selectivity . Efficiency is influenced by solvent polarity, temperature (40–60°C), and extraction time (30–90 min). Comparative studies should report yield percentages and purity via HPLC-UV .
Q. What spectroscopic and chromatographic techniques are essential for characterizing this compound purity and structure?
- Methodological Answer : High-performance liquid chromatography (HPLC) with UV detection at 230 nm is standard for quantification, paired with calibration curves (R² > 0.99) . Structural confirmation requires NMR (¹H/¹³C), FT-IR for functional groups, and mass spectrometry (ESI-MS) for molecular weight validation. Purity thresholds (>95%) should align with USP guidelines .
Q. How to design dose-response studies for this compound’s hepatoprotective effects in preclinical models?
- Methodological Answer : Use in vivo rodent models (e.g., CCl₄-induced hepatotoxicity) with this compound doses ranging 50–200 mg/kg. Include positive controls (silymarin) and measure biomarkers like ALT, AST, and glutathione levels. Statistical analysis via ANOVA with post-hoc tests (p < 0.05) ensures robustness .
Q. What statistical approaches validate the reproducibility of this compound quantification in HPLC analysis?
- Methodological Answer : Intra-day and inter-day precision (RSD < 2%) via triplicate injections, linearity testing across 5–100 µg/mL, and recovery studies (spiked samples at 80–120%). Use ANOVA for inter-lab variability assessment .
Advanced Research Questions
Q. How can response surface methodology (RSM) optimize supercritical fluid extraction parameters for this compound yield?
- Methodological Answer : Apply Box-Behnken design (BBD) with 4 variables: pressure (200–400 bar), temperature (40–60°C), modifier concentration (5–15% methanol), and dynamic time (30–90 min). A second-order polynomial model identifies optimal conditions (e.g., 350 bar, 50°C, 12% modifier), validated through ANOVA (p < 0.05) and lack-of-fit tests .
Q. How do contradictory reports on this compound’s in vitro vs. in vivo bioactivity guide experimental redesign?
- Methodological Answer : Discrepancies (e.g., antioxidant activity in vitro vs. limited bioavailability in vivo) necessitate pharmacokinetic studies (Cmax, Tmax, AUC) and metabolite profiling. Use LC-MS/MS to identify active derivatives and adjust dosing regimens or delivery systems (e.g., nanoemulsions) .
Q. How does molecular docking elucidate this compound’s interaction with hepatic cytochrome P450 enzymes?
- Methodological Answer : Perform in silico docking (AutoDock Vina) using CYP3A4 crystal structures (PDB ID: 1TQN). Analyze binding affinity (ΔG < -8 kcal/mol), hydrogen bonding, and hydrophobic interactions. Validate with in vitro inhibition assays (IC₅₀) and Lineweaver-Burk plots for mechanism determination .
Q. What metabolomic strategies identify this compound’s downstream biomarkers in chronic disease models?
- Methodological Answer : Employ untargeted metabolomics (UPLC-QTOF-MS) on serum/urine samples from treated models. Use multivariate analysis (PCA, OPLS-DA) to identify dysregulated pathways (e.g., lipid peroxidation, arachidonic acid metabolism). Confirm biomarkers via ROC curve analysis (AUC > 0.85) .
Q. Data Presentation Guidelines
- Tables : Include extraction yield comparisons (e.g., SFE vs. Soxhlet) with SD, p-values.
- Figures : 3D response surface plots for RSM optimization, dose-response curves with EC₅₀ values.
- References : Prioritize recent studies (post-2010) from peer-reviewed journals, avoiding non-academic sources.
Properties
IUPAC Name |
4-[(2S,3S)-3-[(3,4-dimethoxyphenyl)methyl]-4-methoxy-2-(methoxymethyl)butyl]-1,2-dimethoxybenzene | |
---|---|---|
Source | PubChem | |
URL | https://pubchem.ncbi.nlm.nih.gov | |
Description | Data deposited in or computed by PubChem | |
InChI |
InChI=1S/C24H34O6/c1-25-15-19(11-17-7-9-21(27-3)23(13-17)29-5)20(16-26-2)12-18-8-10-22(28-4)24(14-18)30-6/h7-10,13-14,19-20H,11-12,15-16H2,1-6H3/t19-,20-/m1/s1 | |
Source | PubChem | |
URL | https://pubchem.ncbi.nlm.nih.gov | |
Description | Data deposited in or computed by PubChem | |
InChI Key |
KFLQGJQSLUYUBF-WOJBJXKFSA-N | |
Source | PubChem | |
URL | https://pubchem.ncbi.nlm.nih.gov | |
Description | Data deposited in or computed by PubChem | |
Canonical SMILES |
COCC(CC1=CC(=C(C=C1)OC)OC)C(CC2=CC(=C(C=C2)OC)OC)COC | |
Source | PubChem | |
URL | https://pubchem.ncbi.nlm.nih.gov | |
Description | Data deposited in or computed by PubChem | |
Isomeric SMILES |
COC[C@@H](CC1=CC(=C(C=C1)OC)OC)[C@H](CC2=CC(=C(C=C2)OC)OC)COC | |
Source | PubChem | |
URL | https://pubchem.ncbi.nlm.nih.gov | |
Description | Data deposited in or computed by PubChem | |
Molecular Formula |
C24H34O6 | |
Source | PubChem | |
URL | https://pubchem.ncbi.nlm.nih.gov | |
Description | Data deposited in or computed by PubChem | |
DSSTOX Substance ID |
DTXSID001319088 | |
Record name | Phyllanthin | |
Source | EPA DSSTox | |
URL | https://comptox.epa.gov/dashboard/DTXSID001319088 | |
Description | DSSTox provides a high quality public chemistry resource for supporting improved predictive toxicology. | |
Molecular Weight |
418.5 g/mol | |
Source | PubChem | |
URL | https://pubchem.ncbi.nlm.nih.gov | |
Description | Data deposited in or computed by PubChem | |
CAS No. |
10351-88-9 | |
Record name | Phyllanthin | |
Source | CAS Common Chemistry | |
URL | https://commonchemistry.cas.org/detail?cas_rn=10351-88-9 | |
Description | CAS Common Chemistry is an open community resource for accessing chemical information. Nearly 500,000 chemical substances from CAS REGISTRY cover areas of community interest, including common and frequently regulated chemicals, and those relevant to high school and undergraduate chemistry classes. This chemical information, curated by our expert scientists, is provided in alignment with our mission as a division of the American Chemical Society. | |
Explanation | The data from CAS Common Chemistry is provided under a CC-BY-NC 4.0 license, unless otherwise stated. | |
Record name | Phyllanthin | |
Source | ChemIDplus | |
URL | https://pubchem.ncbi.nlm.nih.gov/substance/?source=chemidplus&sourceid=0010351889 | |
Description | ChemIDplus is a free, web search system that provides access to the structure and nomenclature authority files used for the identification of chemical substances cited in National Library of Medicine (NLM) databases, including the TOXNET system. | |
Record name | Phyllanthin | |
Source | DTP/NCI | |
URL | https://dtp.cancer.gov/dtpstandard/servlet/dwindex?searchtype=NSC&outputformat=html&searchlist=619043 | |
Description | The NCI Development Therapeutics Program (DTP) provides services and resources to the academic and private-sector research communities worldwide to facilitate the discovery and development of new cancer therapeutic agents. | |
Explanation | Unless otherwise indicated, all text within NCI products is free of copyright and may be reused without our permission. Credit the National Cancer Institute as the source. | |
Record name | Phyllanthin | |
Source | EPA DSSTox | |
URL | https://comptox.epa.gov/dashboard/DTXSID001319088 | |
Description | DSSTox provides a high quality public chemistry resource for supporting improved predictive toxicology. | |
Record name | PHYLLANTHIN | |
Source | FDA Global Substance Registration System (GSRS) | |
URL | https://gsrs.ncats.nih.gov/ginas/app/beta/substances/75O1TFF47Z | |
Description | The FDA Global Substance Registration System (GSRS) enables the efficient and accurate exchange of information on what substances are in regulated products. Instead of relying on names, which vary across regulatory domains, countries, and regions, the GSRS knowledge base makes it possible for substances to be defined by standardized, scientific descriptions. | |
Explanation | Unless otherwise noted, the contents of the FDA website (www.fda.gov), both text and graphics, are not copyrighted. They are in the public domain and may be republished, reprinted and otherwise used freely by anyone without the need to obtain permission from FDA. Credit to the U.S. Food and Drug Administration as the source is appreciated but not required. | |
Retrosynthesis Analysis
AI-Powered Synthesis Planning: Our tool employs the Template_relevance Pistachio, Template_relevance Bkms_metabolic, Template_relevance Pistachio_ringbreaker, Template_relevance Reaxys, Template_relevance Reaxys_biocatalysis model, leveraging a vast database of chemical reactions to predict feasible synthetic routes.
One-Step Synthesis Focus: Specifically designed for one-step synthesis, it provides concise and direct routes for your target compounds, streamlining the synthesis process.
Accurate Predictions: Utilizing the extensive PISTACHIO, BKMS_METABOLIC, PISTACHIO_RINGBREAKER, REAXYS, REAXYS_BIOCATALYSIS database, our tool offers high-accuracy predictions, reflecting the latest in chemical research and data.
Strategy Settings
Precursor scoring | Relevance Heuristic |
---|---|
Min. plausibility | 0.01 |
Model | Template_relevance |
Template Set | Pistachio/Bkms_metabolic/Pistachio_ringbreaker/Reaxys/Reaxys_biocatalysis |
Top-N result to add to graph | 6 |
Feasible Synthetic Routes
Disclaimer and Information on In-Vitro Research Products
Please be aware that all articles and product information presented on BenchChem are intended solely for informational purposes. The products available for purchase on BenchChem are specifically designed for in-vitro studies, which are conducted outside of living organisms. In-vitro studies, derived from the Latin term "in glass," involve experiments performed in controlled laboratory settings using cells or tissues. It is important to note that these products are not categorized as medicines or drugs, and they have not received approval from the FDA for the prevention, treatment, or cure of any medical condition, ailment, or disease. We must emphasize that any form of bodily introduction of these products into humans or animals is strictly prohibited by law. It is essential to adhere to these guidelines to ensure compliance with legal and ethical standards in research and experimentation.