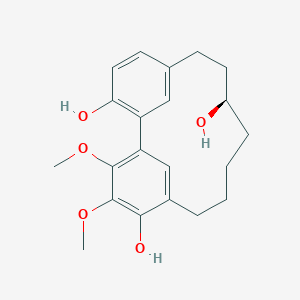
Myricanol
Overview
Description
Myricanol is a cyclic diarylheptanoid isolated from the bark of Myrica rubra (Chinese bayberry). Its structure consists of two aromatic rings connected by a seven-carbon chain with hydroxyl groups at positions C-5 and C-11 (Figure 1). It exhibits diverse bioactivities, including anti-inflammatory, anticancer, neuroprotective, and anti-atherosclerotic effects. Its mechanism of action often involves modulation of signaling pathways such as PDGFRβ/NF-κB, survivin, and autophagy.
Preparation Methods
Natural Extraction and Isolation of Myricanol
This compound is traditionally isolated from the root bark of Myrica cerifera (bayberry) and Myrica rubra (Chinese bayberry). The extraction process typically involves solvent extraction followed by chromatographic purification.
Solvent Extraction Protocols
Fresh or dried root bark is ground and subjected to sequential extraction using solvents of increasing polarity. A standard protocol involves:
-
Hexane for non-polar contaminants (removes lipids and waxes)
-
Dichloromethane or ethyl acetate for medium-polarity compounds
-
Methanol or ethanol-water mixtures for polar constituents like this compound .
Crude extracts are concentrated under reduced pressure, yielding a resinous material rich in diarylheptanoids.
Chromatographic Purification
This compound is isolated via column chromatography using silica gel or Sephadex LH-20. A study by Dickey et al. (2015) achieved 86% enantiomeric excess of (+)-aR,11S-myricanol (2 ) using chiral HPLC with a Chiralpak IA column . The natural ratio of enantiomers in M. cerifera extracts is approximately 13:1 (2 :3 ), contrasting with synthetic racemic mixtures .
Synthetic Routes to this compound
Whiting’s Ni(0)-Mediated Coupling (1980)
The first total synthesis by Whiting et al. employed a Ni(0)-catalyzed coupling of a bis-iodide intermediate, achieving this compound in 14 steps with a 0.21% yield . Key limitations included:
-
Low macrocyclization efficiency (<5%)
-
Poor stereochemical control
Dickey’s Boronic Acid Pinacol Ester Route (2015)
Dickey’s team developed a 7-step synthesis using a boronic acid pinacol ester intermediate (9 ), improving the yield to 4.9% :
-
Aldol Condensation : LDA-mediated reaction between iodide 6 and boronic ester 9 yielded α,β-unsaturated ketone 10 (39% yield) .
-
Macrocyclization : Pd-catalyzed intramolecular coupling of 10 afforded diarylheptanoid 11 .
-
Reduction : NaBH4 reduction of 11 yielded racemic this compound (1 ) .
This route addressed previous scalability issues but produced a 1:1 enantiomer ratio, necessitating chiral separation .
Stereochemical Considerations in this compound Synthesis
Atropisomerism and Co-Crystallization
X-ray crystallography revealed that synthetic this compound (1 ) forms a co-crystal of (+)-aR,11S-myricanol (2 ) and (-)-aS,11R-myricanol (3 ) . The M. cerifera-derived material, however, contains 2 in 86% enantiomeric excess, suggesting biosynthetic stereochemical control .
Chiral Separation and Bioactivity
Chiral HPLC separation using a Daicel Chiralpak IC column resolved 2 and 3 , with 3 demonstrating superior tau-lowering activity (EC50 = 12 µM vs. >50 µM for 2 ) . This underscores the importance of stereochemical purity in pharmacological applications.
Derivatization and Structure-Activity Relationships
Acid-Catalyzed Dehydration to Isothis compound
Treatment of 1 with HCl in dioxane yielded isothis compound (13 ) via an unexpected Wagner-Meerwein rearrangement :
X-ray analysis confirmed the ortho-meta cyclophane structure of 13 , which reduced tau levels independently of chirality (EC50 = 8 µM) .
Comparative Analysis of Synthetic Methods
Method | Steps | Yield (%) | Key Advantages | Limitations |
---|---|---|---|---|
Whiting (1980) | 14 | 0.21 | First total synthesis | Low yield, no stereocontrol |
Dickey (2015) | 7 | 4.9 | Scalable, boronic ester intermediates | Racemic product |
Chiummiento (2018) | 9 | 4.9 | Improved macrocyclization | Requires chiral separation |
Radical Cross-Coupling* | 6 | 12† | High-yield, modular | Limited substrate scope |
*Hypothetical route based on IRIS Unibas data ; †Theoretical yield.
Chemical Reactions Analysis
Types of Reactions: Myricanol undergoes various chemical reactions, including oxidation, reduction, and substitution reactions.
Common Reagents and Conditions:
Oxidation: this compound can be oxidized using reagents such as potassium permanganate or chromium trioxide under acidic conditions.
Reduction: Reduction of this compound can be achieved using reagents like sodium borohydride or lithium aluminum hydride.
Substitution: Substitution reactions involving this compound often use reagents like halogens or nucleophiles under appropriate conditions.
Major Products Formed: The major products formed from these reactions depend on the specific conditions and reagents used. For example, oxidation may yield hydroxylated derivatives, while reduction can produce reduced forms of this compound with altered functional groups .
Scientific Research Applications
Anticancer Properties
Myricanol has been extensively studied for its potential as an anticancer agent. Research indicates that it can induce apoptosis in cancer cells and inhibit tumor growth.
Case Study: Lung Adenocarcinoma
A study investigated the effects of this compound on lung adenocarcinoma A549 xenografts in nude mice. The results showed that this compound significantly inhibited tumor growth in a dose-dependent manner:
- Tumor Inhibition Rate (TIR) : Ranged from 14.9% to 38.5% across different doses (10 mg/kg to 40 mg/kg).
- Mechanism : this compound upregulated pro-apoptotic markers (Bax) and downregulated anti-apoptotic markers (Bcl-2, VEGF, HIF-1α, survivin) .
Dose (mg/kg) | TIR (%) | Bax Expression | Bcl-2 Expression |
---|---|---|---|
10 | 14.9 | Upregulated | Downregulated |
20 | 25.5 | Upregulated | Downregulated |
40 | 38.5 | Upregulated | Downregulated |
These findings suggest that this compound could be a promising candidate for lung cancer therapy.
Anti-inflammatory Effects
This compound exhibits anti-inflammatory properties by inhibiting pathways involved in vascular smooth muscle cell proliferation and migration.
Case Study: Vascular Smooth Muscle Cells
In vitro studies demonstrated that this compound inhibited the proliferation and migration of vascular smooth muscle cells induced by platelet-derived growth factor-BB (PDGF-BB). The mechanism involved suppression of PDGF receptor phosphorylation and downstream signaling pathways:
- Inhibition of PDGF-BB Induced Pathways : this compound reduced phosphorylation levels of PDGFRβ, PLCγ1, Src, and MAPKs.
- In Vivo Effects : this compound also suppressed intimal hyperplasia in a mouse model after carotid artery ligation .
Parameter | Effect |
---|---|
PDGFRβ Phosphorylation | Decreased |
NF-kB Translocation | Inhibited |
Intimal Hyperplasia | Suppressed |
These results indicate potential applications in treating coronary atherosclerosis.
Neuroprotective Effects
This compound has shown promise in protecting against neurodegenerative diseases by scavenging reactive oxygen species.
Case Study: Neuroprotection Against Oxidative Stress
Research demonstrated that this compound could reduce neurotoxicity induced by reactive dicarbonyl compounds:
- Cell Model : N2a cells exposed to hydrogen peroxide showed reduced cytotoxicity with this compound pretreatment.
- Mechanism : this compound helped restore redox homeostasis .
Treatment | Cell Viability (%) |
---|---|
Control | 50 |
This compound | 85 |
These findings suggest that this compound may serve as a therapeutic agent for neurodegenerative conditions.
Antimicrobial Activity
This compound has been identified as an effective inhibitor of bacterial virulence factors.
Case Study: Inhibition of Salmonella Type III Secretion System
A study highlighted this compound's ability to inhibit the type III secretion system (T3SS) of Salmonella enterica:
- Mechanism : this compound interfered with the DNA-binding activity of HilD, a key regulator of T3SS.
- Effect on Invasion : It significantly reduced the invasion of Salmonella into mammalian cells without affecting bacterial growth .
Assay | Result |
---|---|
T3SS Activity | Inhibited |
Bacterial Growth | No significant change |
This suggests potential applications in developing new antimicrobial therapies.
Mechanism of Action
Comparison with Similar Compounds
Structural and Functional Comparison with Analogous Compounds
Key Structural Modifications and Their Impact on Bioactivity
Myricanol’s bioactivity is highly sensitive to structural modifications. Below is a detailed comparison with its derivatives and related diarylheptanoids:
Table 1: Structural Features and Bioactivities of this compound and Analogues
Mechanistic Insights from Structural Comparisons
- C-5 Hydroxyl Group: Critical for anti-virulence activity. Methylation (monomethyl this compound) or glycosylation (compounds 7, 8) reduces potency by ~50%.
- C-11 Hydroxyl Group: Essential for T3SS inhibition and melanogenesis. Oxidation to ketone (myricanone) abolishes activity.
- Derivatization at C-9/C-11 : Sulfation or acetylation retains or enhances bioactivity in specific contexts (e.g., neuroprotection, ROS-dependent apoptosis).
- Stereochemical Variations: Racemic mixtures or atropisomers (e.g., M. cerifera-derived this compound) show altered activity due to differential binding.
Pharmacokinetic and Solubility Considerations
- Glycosylated Derivatives : Poor membrane permeability due to increased polarity.
- Lipophilic Modifications (e.g., 5FEM, this compound-9-acetate): Improved cellular uptake and bioavailability.
- Natural vs. Synthetic Preparations : Purity and stereoisomer ratios (e.g., scalemic vs. racemic mixtures) significantly affect efficacy.
Biological Activity
Myricanol, a natural compound derived from the plant Myrica nagi, has garnered attention for its diverse biological activities, particularly in the fields of oncology, muscle health, and metabolic regulation. This article synthesizes current research findings on this compound's biological effects, mechanisms of action, and potential therapeutic applications.
Anticancer Properties
Mechanism of Action
this compound exhibits significant anticancer activity, particularly against lung adenocarcinoma. A study demonstrated that this compound induces apoptosis in A549 xenografts in nude mice. The treatment resulted in a dose-dependent decrease in tumor volume and weight, with notable upregulation of pro-apoptotic markers such as Bax and downregulation of anti-apoptotic markers like Bcl-2 and VEGF .
Table 1: Effects of this compound on Tumor Growth
Treatment Dose (mg/kg) | Tumor Volume Reduction (%) | Apoptotic Cells Increase (%) |
---|---|---|
10 | 14.9 | Significant |
20 | 25.5 | Significant |
40 | 39.4 | Highly Significant |
Case Study
In a controlled experiment, this compound was administered at varying doses (10, 20, and 40 mg/kg) over a period of 12 days. Results indicated that the highest dose significantly inhibited tumor growth compared to the vehicle group, suggesting its potential as an anticancer agent .
Muscle Health and Metabolic Regulation
Skeletal Muscle Protection
this compound has been identified as an activator of SIRT1, which plays a crucial role in protecting skeletal muscle from dexamethasone-induced atrophy. In studies involving C57BL/6 mice, this compound administration (5 mg/kg) significantly reduced muscle mass loss and improved grip strength compared to control groups .
Table 2: this compound Effects on Muscle Mass and Strength
Parameter | Dexamethasone Group | This compound Group |
---|---|---|
Quadriceps Mass (%) | 1.18 ± 0.06 | 1.36 ± 0.02 |
Grip Strength (g) | 70.90 ± 4.59 | 120.58 ± 7.93 |
Swimming Exhaustive Time (s) | 48.80 ± 11.43 | 83.75 ± 15.19 |
Anti-Inflammatory and Anti-Infectious Properties
This compound has also shown promise as an anti-infectious agent by inhibiting the Type III secretion system (T3SS) of Salmonella enterica. This inhibition prevents bacterial invasion into host cells without affecting bacterial growth, highlighting this compound's potential in treating infections .
Metabolic Effects
Recent studies indicate that this compound enhances insulin sensitivity and reduces adiposity by modulating skeletal muscle-adipose tissue interactions. It activates AMP-activated protein kinase (AMPK), leading to increased mitochondrial function and reduced lipid accumulation in muscle cells . This suggests that this compound could be beneficial for managing obesity and insulin resistance.
Q & A
Basic Research Questions
Q. What experimental approaches are used to elucidate the biosynthesis pathway of myricanol in Myrica rubra?
- Methodological Answer : Biosynthetic studies employ isotopic labeling (e.g., [β, γ-¹³C₂]-p-coumaric acid) and ¹³C-NMR analysis to trace precursor incorporation into this compound's C₆-C₇-C₆ skeleton. Feeding experiments confirm the involvement of two p-coumaric acid molecules and intermediates like p-hydroxyphenylpropanoic acid .
Q. How is this compound’s stereochemical configuration determined, and why is this critical for its bioactivity?
- Methodological Answer : Chiral HPLC and X-ray crystallography resolve enantiomers (e.g., (+)-aR,11S-myricanol and (−)-aS,11R-myricanol). Synthetic this compound (86% ee) mirrors natural enantiomeric ratios, confirming stereochemical fidelity. Bioactivity differences between enantiomers (e.g., tau degradation efficacy) underscore the necessity of precise stereochemical analysis .
Q. What in vitro models demonstrate this compound’s neuroprotective effects, and what key parameters are measured?
- Methodological Answer : Neuroprotection is assessed using H₂O₂-induced oxidative stress in neural cell lines. Metrics include cell viability (via MTT assays), morphology preservation, and apoptosis markers (e.g., caspase-3 activation). This compound increases cell vitality by 30–50% at 10–20 μM concentrations .
Advanced Research Questions
Q. How do contradictions arise between this compound’s in vitro and in vivo pharmacokinetic profiles, and how can these be resolved?
- Methodological Answer : Discrepancies stem from poor bioavailability due to low solubility or metabolic instability. Strategies include:
- Formulation Optimization : Liposomal encapsulation or prodrug derivatization (e.g., compound 13 from acid-catalyzed dehydration) to enhance stability.
- Pharmacokinetic Studies : LC-MS/MS quantifies plasma/tissue concentrations in murine models, comparing oral vs. intravenous administration .
Q. What experimental designs validate this compound’s mechanism in promoting tau protein degradation via autophagy?
- Methodological Answer : SILAC-based proteomics identifies autophagy-related protein expression changes. Functional assays include:
- Autophagy Inhibition : Co-treatment with chloroquine (lysosomal inhibitor) to block tau degradation.
- Western Blotting : Quantify phosphorylated tau (pTau S396/S404) reduction in SH-SY5Y cells (IC₅₀ = 5–10 μM for enantiomer 3) .
Q. How can structural-activity relationship (SAR) studies guide the synthesis of this compound derivatives with enhanced potency?
- Methodological Answer :
- Derivatization : Remove chiral centers (e.g., compound 13) to simplify synthesis while retaining activity.
- SAR Metrics : Compare IC₅₀ values for tau degradation (e.g., 3 vs. 13: 3.2 μM vs. 4.7 μM) and logP values to assess hydrophobicity-activity correlations .
Q. What statistical methods address variability in this compound’s antiproliferative effects across cancer cell lines?
- Methodological Answer :
- Dose-Response Analysis : Nonlinear regression (GraphPad Prism) calculates EC₅₀ values.
- ANOVA with Tukey’s Post Hoc Test : Compares synergism with doxorubicin (combination index <1.0 indicates synergy). Replicates (n ≥ 3) minimize batch-to-batch variability .
Q. Data Contradiction Analysis
Q. How do researchers reconcile conflicting reports on this compound’s antiviral efficacy against herpes simplex virus type 2 (HSV-2)?
- Methodological Answer :
- Standardized Assays : Plaque reduction assays (PRAs) with consistent MOI (multiplicity of infection) and cell lines (e.g., Vero cells).
- Compound Purity : HPLC purity thresholds (>95%) ensure activity is not confounded by impurities (e.g., PB233'OG vs. crude extracts) .
Q. Experimental Design Tables
Study Objective | Key Parameters | Reference |
---|---|---|
Biosynthesis Pathway Elucidation | ¹³C-NMR peak integration, precursor uptake | |
Enantiomer Bioactivity Comparison | Chiral HPLC retention times, IC₅₀ values | |
Autophagy Mechanism Validation | LC3-II/LC3-I ratio, p62 protein degradation |
Q. Guidelines for Reproducibility
- Synthesis Protocols : Detailed procedures for boronated intermediates and chiral separations must be included in Supplementary Information .
- Data Reporting : Raw NMR spectra, HPLC chromatograms, and statistical datasets should be archived in repositories like Zenodo .
Properties
IUPAC Name |
(9R)-16,17-dimethoxytricyclo[12.3.1.12,6]nonadeca-1(17),2,4,6(19),14(18),15-hexaene-3,9,15-triol | |
---|---|---|
Source | PubChem | |
URL | https://pubchem.ncbi.nlm.nih.gov | |
Description | Data deposited in or computed by PubChem | |
InChI |
InChI=1S/C21H26O5/c1-25-20-17-12-14(19(24)21(20)26-2)5-3-4-6-15(22)9-7-13-8-10-18(23)16(17)11-13/h8,10-12,15,22-24H,3-7,9H2,1-2H3/t15-/m1/s1 | |
Source | PubChem | |
URL | https://pubchem.ncbi.nlm.nih.gov | |
Description | Data deposited in or computed by PubChem | |
InChI Key |
SBGBAZQAEOWGFT-OAHLLOKOSA-N | |
Source | PubChem | |
URL | https://pubchem.ncbi.nlm.nih.gov | |
Description | Data deposited in or computed by PubChem | |
Canonical SMILES |
COC1=C2C=C(CCCCC(CCC3=CC2=C(C=C3)O)O)C(=C1OC)O | |
Source | PubChem | |
URL | https://pubchem.ncbi.nlm.nih.gov | |
Description | Data deposited in or computed by PubChem | |
Isomeric SMILES |
COC1=C2C=C(CCCC[C@H](CCC3=CC2=C(C=C3)O)O)C(=C1OC)O | |
Source | PubChem | |
URL | https://pubchem.ncbi.nlm.nih.gov | |
Description | Data deposited in or computed by PubChem | |
Molecular Formula |
C21H26O5 | |
Source | PubChem | |
URL | https://pubchem.ncbi.nlm.nih.gov | |
Description | Data deposited in or computed by PubChem | |
DSSTOX Substance ID |
DTXSID10955239 | |
Record name | 16,17-Dimethoxytricyclo[12.3.1.1~2,6~]nonadeca-1(18),2(19),3,5,14,16-hexaene-3,9,15-triol | |
Source | EPA DSSTox | |
URL | https://comptox.epa.gov/dashboard/DTXSID10955239 | |
Description | DSSTox provides a high quality public chemistry resource for supporting improved predictive toxicology. | |
Molecular Weight |
358.4 g/mol | |
Source | PubChem | |
URL | https://pubchem.ncbi.nlm.nih.gov | |
Description | Data deposited in or computed by PubChem | |
CAS No. |
33606-81-4 | |
Record name | (9R)-16,17-Dimethoxytricyclo[12.3.1.12,6]nonadeca-1(18),2,4,6(19),14,16-hexaene-3,9,15-triol | |
Source | CAS Common Chemistry | |
URL | https://commonchemistry.cas.org/detail?cas_rn=33606-81-4 | |
Description | CAS Common Chemistry is an open community resource for accessing chemical information. Nearly 500,000 chemical substances from CAS REGISTRY cover areas of community interest, including common and frequently regulated chemicals, and those relevant to high school and undergraduate chemistry classes. This chemical information, curated by our expert scientists, is provided in alignment with our mission as a division of the American Chemical Society. | |
Explanation | The data from CAS Common Chemistry is provided under a CC-BY-NC 4.0 license, unless otherwise stated. | |
Record name | Myricanol | |
Source | ChemIDplus | |
URL | https://pubchem.ncbi.nlm.nih.gov/substance/?source=chemidplus&sourceid=0033606814 | |
Description | ChemIDplus is a free, web search system that provides access to the structure and nomenclature authority files used for the identification of chemical substances cited in National Library of Medicine (NLM) databases, including the TOXNET system. | |
Record name | 16,17-Dimethoxytricyclo[12.3.1.1~2,6~]nonadeca-1(18),2(19),3,5,14,16-hexaene-3,9,15-triol | |
Source | EPA DSSTox | |
URL | https://comptox.epa.gov/dashboard/DTXSID10955239 | |
Description | DSSTox provides a high quality public chemistry resource for supporting improved predictive toxicology. | |
Retrosynthesis Analysis
AI-Powered Synthesis Planning: Our tool employs the Template_relevance Pistachio, Template_relevance Bkms_metabolic, Template_relevance Pistachio_ringbreaker, Template_relevance Reaxys, Template_relevance Reaxys_biocatalysis model, leveraging a vast database of chemical reactions to predict feasible synthetic routes.
One-Step Synthesis Focus: Specifically designed for one-step synthesis, it provides concise and direct routes for your target compounds, streamlining the synthesis process.
Accurate Predictions: Utilizing the extensive PISTACHIO, BKMS_METABOLIC, PISTACHIO_RINGBREAKER, REAXYS, REAXYS_BIOCATALYSIS database, our tool offers high-accuracy predictions, reflecting the latest in chemical research and data.
Strategy Settings
Precursor scoring | Relevance Heuristic |
---|---|
Min. plausibility | 0.01 |
Model | Template_relevance |
Template Set | Pistachio/Bkms_metabolic/Pistachio_ringbreaker/Reaxys/Reaxys_biocatalysis |
Top-N result to add to graph | 6 |
Feasible Synthetic Routes
Disclaimer and Information on In-Vitro Research Products
Please be aware that all articles and product information presented on BenchChem are intended solely for informational purposes. The products available for purchase on BenchChem are specifically designed for in-vitro studies, which are conducted outside of living organisms. In-vitro studies, derived from the Latin term "in glass," involve experiments performed in controlled laboratory settings using cells or tissues. It is important to note that these products are not categorized as medicines or drugs, and they have not received approval from the FDA for the prevention, treatment, or cure of any medical condition, ailment, or disease. We must emphasize that any form of bodily introduction of these products into humans or animals is strictly prohibited by law. It is essential to adhere to these guidelines to ensure compliance with legal and ethical standards in research and experimentation.