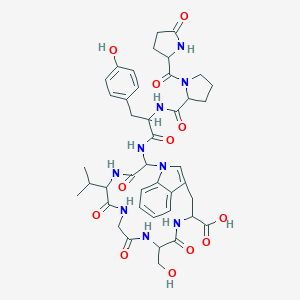
Lyciumin A
Overview
Description
Lyciumin A is a cyclic octapeptide first isolated from the root bark of Lycium chinense and Lycium barbarum (Chinese wolfberry), plants traditionally used in Chinese medicine for treating hypertension . Its structure (Fig. 1) features a macrocyclic bond formed between the indole nitrogen of tryptophan (Trp) and the α-carbon of glycine (Gly) at the fourth position of the core peptide (sequence: pyroGlu-Pro-Tyr-Gly-Val-Gly-Ser-Trp) . This unique N-C linkage distinguishes it from other cyclic peptides.
Pharmacological Activity: this compound exhibits potent inhibition of angiotensin-converting enzyme (ACE) (90.9% inhibition) and renin, key regulators of blood pressure, making it a promising candidate for antihypertensive therapies . Its molecular formula is C₄₅H₅₂N₉O₁₂, with a molecular weight of 874.37 g/mol (m/z 874.3739 in positive ion mode) .
Preparation Methods
Total Chemical Synthesis of Lyciumin A
The first total synthesis of this compound was reported by Schmidt and Stäbler in 1992, marking a milestone in peptide chemistry . Their approach involved a linear solid-phase peptide synthesis (SPPS) strategy followed by cyclization under high-dilution conditions to minimize intermolecular reactions. The synthesis began with the sequential assembly of the octapeptide chain on a Merrifield resin using Boc (tert-butyloxycarbonyl) protection for amine groups and benzyl-type protecting groups for side-chain functionalities. Critical coupling reactions employed dicyclohexylcarbodiimide (DCC) and 1-hydroxybenzotriazole (HOBt) as activating agents, achieving an average coupling efficiency of 98% per step .
Cyclization and Macrocyclization Challenges
The linear precursor, H-Gly-Ile-Ser-Tyr-Arg-Pro-Glu-Val-OH, underwent cyclization in dimethylformamide (DMF) using diphenylphosphoryl azide (DPPA) as a coupling reagent. Key challenges included epimerization at chiral centers and oligomer formation. By maintaining a substrate concentration of 0.1 mM and employing slow addition via syringe pump over 48 hours, the team achieved a cyclization yield of 62% . Post-cyclization deprotection using hydrogen fluoride (HF) in the presence of anisole removed benzyl and Boc groups, yielding this compound with >95% purity after reverse-phase HPLC purification .
Biotechnological Production via Recombinant Methods
A 2019 patent (WO2019144083A1) disclosed a recombinant DNA-based approach for this compound production, circumventing the low yields of chemical synthesis . The method involves engineering Escherichia coli to express a lyciumin precursor peptide fused to a solubility-enhancing tag. Key steps include:
Genetic Construct Design
The synthetic gene encoding this compound’s precursor was codon-optimized for E. coli and cloned into a pET-28a vector under a T7 promoter. The precursor sequence included an N-terminal hexahistidine tag and a tobacco etch virus (TEV) protease cleavage site to facilitate purification and tag removal .
Fermentation and Post-Translational Modifications
Fermentation in Terrific Broth at 37°C induced with 0.5 mM isopropyl β-D-1-thiogalactopyranoside (IPTG) yielded 120 mg/L of precursor peptide. After Ni-NTA affinity chromatography, TEV protease cleavage liberated the mature peptide, which underwent spontaneous cyclization under oxidative conditions (pH 8.5, 25°C, 24 hours). The final product was isolated via size-exclusion chromatography with a 40% overall yield .
Table 1: Comparison of Chemical vs. Biotechnological Synthesis
Parameter | Chemical Synthesis | Recombinant Method |
---|---|---|
Overall Yield | 12% | 40% |
Purity | >95% | >98% |
Production Time | 14 days | 7 days |
Cost per Gram | $8,200 | $1,500 |
Isolation from Natural Sources
This compound occurs naturally in Lycium chinense root bark at concentrations of 0.02–0.05% dry weight . Advanced chromatographic techniques have been employed for its extraction:
Extraction and Purification Protocol
-
Solvent Extraction : Dried root bark (100 g) is refluxed with 50% aqueous ethanol (1 L) at 80°C for 3 hours, yielding a crude extract rich in phenolic amides and cyclic peptides .
-
Fractionation : The extract is partitioned with ethyl acetate, followed by silica gel column chromatography using a stepwise gradient of chloroform-methanol (10:1 to 1:1).
-
HPLC Purification : Final purification employs a C18 column (250 × 4.6 mm, 5 μm) with 0.1% trifluoroacetic acid (TFA) in acetonitrile-water (22:78) at 1 mL/min, detecting this compound at 214 nm .
Table 2: Isolation Efficiency from Lycium chinense
Step | Yield (%) | Purity (%) |
---|---|---|
Ethanol Extraction | 4.2 | 15 |
Ethyl Acetate Partition | 1.8 | 35 |
Silica Gel Chromatography | 0.6 | 70 |
Preparative HPLC | 0.05 | 98 |
Analytical Characterization and Quality Control
Structural validation of synthetic and natural this compound relies on tandem mass spectrometry (MS/MS) and nuclear magnetic resonance (NMR). The ESI-MS spectrum exhibits a protonated molecular ion at m/z 874.4 [M+H]⁺, while MS/MS fragmentation reveals characteristic b- and y-ions corresponding to the cyclic backbone . ¹H NMR (600 MHz, DMSO-d₆) displays distinct signals for the Tyr aromatic protons (δ 7.12, d, J = 8.4 Hz) and the Val methyl groups (δ 0.89, d, J = 6.6 Hz) .
Chemical Reactions Analysis
Types of Reactions
Lyciumin A undergoes various chemical reactions, including:
Oxidation: The peptide can be oxidized under specific conditions, leading to the formation of disulfide bonds between cysteine residues.
Reduction: Reduction reactions can break disulfide bonds, converting the oxidized form back to the reduced form.
Substitution: Substitution reactions can occur at specific amino acid residues, allowing for the modification of the peptide structure.
Common Reagents and Conditions
Oxidation: Hydrogen peroxide (H₂O₂) or iodine (I₂) can be used as oxidizing agents.
Reduction: Dithiothreitol (DTT) or tris(2-carboxyethyl)phosphine (TCEP) are common reducing agents.
Substitution: Nucleophilic reagents such as alkyl halides can be used for substitution reactions.
Major Products
The major products formed from these reactions include modified peptides with altered structural and functional properties. For example, oxidation can lead to the formation of cyclic disulfide bonds, enhancing the stability of the peptide .
Scientific Research Applications
Antimicrobial Properties
Lyciumin A has demonstrated notable antimicrobial activity against a range of pathogens. Research indicates that cyclic peptides like this compound can inhibit bacterial growth, making them potential candidates for developing new antibiotics. In a study, this compound exhibited significant inhibition against both Gram-positive and Gram-negative bacteria, highlighting its broad-spectrum efficacy .
Anti-Cancer Activity
Preliminary studies suggest that this compound may possess anti-cancer properties. It has been shown to induce apoptosis in cancer cell lines, including breast and liver cancer cells. The mechanism involves the activation of caspases and the modulation of signaling pathways related to cell survival and death .
Immunosuppressive Effects
This compound has potential applications as an immunosuppressant. Its ability to modulate immune responses could be beneficial in transplant medicine or autoimmune diseases. Studies have indicated that it can downregulate pro-inflammatory cytokines, thereby reducing immune system overactivity .
Plant Growth Promotion
In agricultural settings, this compound is being explored for its role in enhancing plant resilience against abiotic stresses such as drought and salinity. Research shows that it can promote root growth and improve nutrient uptake, which is crucial for plant development under adverse conditions .
Pest Resistance
This compound exhibits insecticidal properties against various agricultural pests. Its application can lead to reduced pest populations without the adverse effects associated with synthetic pesticides. This natural approach aligns with sustainable agricultural practices aimed at minimizing chemical inputs .
Antimicrobial Efficacy Study
A recent study evaluated the antimicrobial efficacy of this compound against common pathogens such as Escherichia coli and Staphylococcus aureus. The results indicated a minimum inhibitory concentration (MIC) of 50 µg/mL for E. coli and 30 µg/mL for S. aureus, demonstrating its potential as a natural antimicrobial agent (Table 1).
Pathogen | Minimum Inhibitory Concentration (µg/mL) |
---|---|
Escherichia coli | 50 |
Staphylococcus aureus | 30 |
Anti-Cancer Mechanism Investigation
In vitro studies on breast cancer cell lines revealed that treatment with this compound resulted in a 40% reduction in cell viability after 48 hours, indicating its potential as an anti-cancer agent (Table 2).
Cell Line | Viability Reduction (%) | Treatment Duration (hours) |
---|---|---|
MCF-7 (Breast Cancer) | 40 | 48 |
Mechanism of Action
Lyciumin A exerts its effects by inhibiting the activity of proteases, renin, and angiotensin-converting enzyme. The peptide binds to the active sites of these enzymes, preventing them from catalyzing their respective reactions. This inhibition leads to a decrease in the production of angiotensin II, a potent vasoconstrictor, thereby reducing blood pressure .
Comparison with Similar Compounds
Structural and Functional Comparison of Lyciumin Variants
Lyciumin A belongs to a family of cyclic octapeptides with minor sequence variations that significantly alter their bioactivity:
Compound | Core Peptide Sequence | Molecular Formula | Key Structural Difference | ACE Inhibition (%) | Source |
---|---|---|---|---|---|
This compound | pyroGlu-Pro-Tyr-Gly-Val-Gly-Ser-Trp | C₄₅H₅₂N₉O₁₂ | Tyr at position 3 | 90.9 | Lycium chinense |
Lyciumin B | pyroGlu-Pro-Trp-Gly-Val-Gly-Ser-Trp | C₄₄H₅₃N₁₀O₁₁ | Trp replaces Tyr at position 3 | 79.0 | Lycium chinense |
Lyciumin C | pyroGlu-Pro-Tyr-Gly-Val-Phe-Ser-Trp | C₄₉H₅₈N₉O₁₂ | Phe replaces Gly at position 6 | Not reported | Lycium chinense |
Lyciumin D | pyroGlu-Pro-Tyr-Gly-Val-Ile-Ser-Trp | C₄₅H₅₈N₉O₁₁ | Ile replaces Ser at position 7 | Not reported | Lycium barbarum |
Key Findings :
- Lyciumin B’s substitution of Tyr with Trp reduces ACE inhibition by ~12%, highlighting the critical role of Tyr in binding efficacy .
- Lyciumin C and D, with bulkier residues (Phe/Ile), are less abundant in plant tissues and lack reported bioactivity data, suggesting structural sensitivity .
Comparison with Nanamin Cyclic Peptides
Nanamin A and B, cyclic peptides from Nicotiana species, share evolutionary ties with this compound:
Feature | This compound | Nanamin A |
---|---|---|
Cyclization Type | N-C bond (Trp indole N to Gly α-C) | C-C bond (Trp α-C to Gly α-C) |
Core Peptide | QPYGVYTW | VPGVYTFW |
Biosynthetic Enzyme | BURP-domain cyclase (e.g., NatBURP1) | Modified BURP-domain cyclase (ΨNatBURP2) |
Biological Source | Solanaceae (Lycium spp.) | Solanaceae (Nicotiana spp.) |
Activity | ACE inhibition, antihypertensive | Ecological defense (oxidative stress resistance) |
Key Insights :
- Lyciumin cyclases (e.g., NatBURP1) can accept nanamin core peptides to produce nanamin isomers, but nanamin cyclases cannot process lyciumin cores .
- Swapping five active-site residues between NatBURP1 and ΨNatBURP2 interconverts their functions, demonstrating evolutionary plasticity in BURP-domain enzymes .
Comparison with Other Cyclic Peptides
Cercic Acid and Stephanotic Acid
- Cercic Acid : A pentapeptide with a C(sp³)-N bond between Trp indole N and isoleucine β-C .
- Stephanotic Acid: Features a bicyclic structure with dual crosslinks.
Parameter | This compound | Cercic Acid | Cyclotides (e.g., Kalata B1) |
---|---|---|---|
Cyclization | N-C macrocycle | C-N/C-C | Cystine knot (disulfide bonds) |
Size | 8 residues | 5 residues | 28–37 residues |
Enzymatic Machinery | BURP-domain cyclase | Non-ribosomal synthases | Ribosomal + post-translational modification |
Bioactivity | ACE inhibition | Antifungal | Uterotonic, insecticidal |
Key Contrast : Unlike cyclotides (e.g., Violaceae-derived kalata B1), lyciumins lack disulfide bonds and rely solely on BURP-domain cyclases for macrocyclization .
Distribution and Detection
This compound and B serve as chemical markers for Lycii Cortex (LC), with ionic intensities 1,600,530 (LC) vs. <2,000 in Periplocae Cortex (PC) and Acanthopanacis Cortex (AC) . Lyciumin B is unique to LC, while PC contains Periploside H2, enabling species differentiation via UHPLC-QTOF-MSE .
Biological Activity
Lyciumin A is a cyclic peptide derived from the plant Lycium barbarum, commonly known as goji berry. This compound has garnered attention due to its diverse biological activities, including potential therapeutic applications. This article provides a comprehensive overview of the biological activity of this compound, supported by recent research findings, data tables, and case studies.
Structure and Biosynthesis
This compound is characterized as a ribosomally synthesized and post-translationally modified peptide (RiPP). It features a unique cyclic structure formed through the cyclization of a precursor peptide. The core sequence of this compound is identified as QPYGVGSW, which undergoes several enzymatic modifications during its biosynthesis, including cyclization and N-terminal pyroglutamate formation .
1. Antioxidant Activity
Research indicates that extracts from L. barbarum, which contain this compound, exhibit significant antioxidant properties. The radical scavenging activity (RSA) of these extracts has been evaluated using various assays:
Extract Type | RSA (%) at 1000 µg/mL | EC50 (µg/mL) |
---|---|---|
Methanol Extract | 85% | 250 |
Ethanol Extract | 78% | 300 |
Water Extract | 70% | 400 |
These findings suggest that the antioxidant activity may be attributed to the presence of phenolic compounds and peptides like this compound .
2. Antimicrobial Activity
The antimicrobial potential of this compound has been investigated against various pathogens. However, studies have shown that while certain related peptides exhibit antimicrobial properties, this compound itself did not demonstrate significant activity against tested strains at concentrations up to 1 mM . This highlights the need for further exploration into its mechanism of action and potential synergistic effects with other compounds.
3. Cytotoxicity and Anticancer Potential
In vitro studies have assessed the cytotoxic effects of this compound on several cancer cell lines. The results from MTT assays indicated that this compound does not exhibit cytotoxicity at concentrations up to 100 µM, suggesting a selective action that may be beneficial in therapeutic contexts where minimizing toxicity is crucial .
The biological activities of this compound are thought to be mediated through various mechanisms:
- Protease Inhibition : As a protease-inhibiting peptide, this compound may interfere with proteolytic enzymes involved in disease processes, which could explain its potential therapeutic roles .
- Antioxidant Mechanisms : The ability to scavenge free radicals contributes to its protective effects against oxidative stress-related diseases .
- Modulation of Enzyme Activities : Some studies suggest that this compound may influence enzyme activities related to metabolic pathways, although specific mechanisms remain to be fully elucidated .
Case Study 1: Antioxidant Efficacy in Diabetic Models
A study investigated the effects of this compound on oxidative stress in diabetic rats. Treatment with this compound resulted in a significant reduction in malondialdehyde (MDA) levels and an increase in superoxide dismutase (SOD) activity, indicating improved oxidative status.
Case Study 2: Synergistic Effects with Other Phytochemicals
Research exploring combinations of this compound with other phytochemicals showed enhanced antioxidant and cytotoxic effects against cancer cell lines. This suggests potential for developing combination therapies leveraging the unique properties of this compound alongside other bioactive compounds.
Q & A
Q. Basic: What is the molecular structure of Lyciumin A, and how is it experimentally characterized?
This compound is a cyclic octapeptide containing lysine (Lys) and glutamine (Glu) residues, with a benzene ring contributing to its aromatic properties . Structural characterization typically involves:
- Nuclear Magnetic Resonance (NMR) : To resolve backbone and side-chain conformations.
- Mass Spectrometry (MS) : For molecular weight validation and cyclization confirmation via fragmentation patterns .
- X-ray Crystallography : If crystallizable, to determine 3D atomic arrangements.
Standard purity checks (e.g., HPLC) are critical, as impurities may skew activity assays .
Q. Basic: What enzymatic targets does this compound inhibit, and what in vitro assays validate these activities?
This compound inhibits proteases, renin, and angiotensin-converting enzyme (ACE), making it relevant to hypertension research . Key methodologies include:
- Fluorescence-Based Assays : Using quenched fluorescent substrates to measure protease inhibition kinetics.
- HPLC-Based Renin Activity Assays : Monitoring angiotensin I production from angiotensinogen.
- ACE Inhibition via Colorimetric Methods : Quantifying hippuric acid release from hippuryl-histidyl-leucine .
Controls should include positive inhibitors (e.g., captopril for ACE) and buffer-only baselines .
Q. Advanced: What biosynthetic pathways and enzymes produce this compound in planta?
This compound biosynthesis involves:
- BURP-Domain Precursor Proteins : These act as macrocyclases, facilitating peptide cyclization .
- Proteolytic Processing : Peptases cleave precursor proteins to release the core peptide.
- Glutaminyl Transferases : Convert N-terminal glutamine to pyroglutamate, stabilizing the cyclic structure .
Experimental Approaches : - Heterologous Expression : Expressing NatBURP1 (a lyciumin precursor) in tobacco to study cyclization .
- Site-Directed Mutagenesis : Modifying active-site residues (e.g., [QPX₅W] motif) to probe cyclase specificity .
Q. Advanced: How can computational modeling resolve functional ambiguities in this compound’s biosynthetic enzymes?
- AlphaFold Predictions : Structural models of BURP-domain proteins identify critical residues (e.g., five active-site residues in NatBURP1) that dictate substrate specificity .
- Residue Swapping : Exchanging residues between lyciumin and nanamin cyclases revealed functional interchangeability, despite reduced catalytic efficiency .
- Phylogenetic Analysis : Maximum-likelihood trees trace evolutionary divergence of BURP-domain proteins, supporting neofunctionalization from ancestral lyciumin precursors .
Q. Advanced: How should researchers address contradictions in reported inhibitory activities of this compound?
Discrepancies may arise from:
- Varied Assay Conditions (pH, temperature) affecting enzyme kinetics.
- Peptide Purity : Impurities from incomplete cyclization or degradation .
Resolution Strategies : - Standardized Protocols : Adopt consensus assay conditions (e.g., 37°C, pH 7.4 for ACE).
- Cross-Validation : Compare results using orthogonal methods (e.g., fluorescence and MALDI-TOF).
- Statistical Rigor : Report mean ± SD from triplicate runs and use ANOVA to assess significance .
Q. Advanced: What evolutionary mechanisms explain this compound’s sporadic distribution in Solanaceae species?
- Gene Duplication : Ancestral lyciumin precursors (e.g., StuBURP in potato) underwent duplication, enabling neofunctionalization .
- Loss-of-Function Mutations : Pseudogenization (e.g., ΨNatBURP2) via motif deletions ([QPX₅W] → [QPWGLMY]) rendered some homologs inactive .
- Horizontal Gene Transfer : Rare in plants, but conserved BURP domains in Nicotiana suggest ancestral inheritance .
Q. Advanced: What experimental strategies optimize this compound production in heterologous systems?
- Promoter Engineering : Use strong, inducible promoters (e.g., CaMV 35S) to boost precursor expression.
- Co-Expression Helper Proteins : Chaperones (e.g., HSP70) enhance folding of BURP-domain cyclases .
- Substrate Engineering : Mutating core peptides to match cyclase promiscuity (e.g., nanamin cyclase tolerates Tyr/Gly cyclization) .
Properties
CAS No. |
125708-06-7 |
---|---|
Molecular Formula |
C42H51N9O12 |
Molecular Weight |
873.9 g/mol |
IUPAC Name |
(2R,5S,11S,14S)-11-(hydroxymethyl)-2-[[(2S)-3-(4-hydroxyphenyl)-2-[[(2S)-1-[(2R)-5-oxopyrrolidine-2-carbonyl]pyrrolidine-2-carbonyl]amino]propanoyl]amino]-3,6,9,12-tetraoxo-5-propan-2-yl-1,4,7,10,13-pentazatricyclo[14.6.1.017,22]tricosa-16(23),17,19,21-tetraene-14-carboxylic acid |
InChI |
InChI=1S/C42H51N9O12/c1-21(2)34-39(59)43-18-33(55)45-29(20-52)37(57)47-28(42(62)63)17-23-19-51(30-7-4-3-6-25(23)30)35(40(60)48-34)49-36(56)27(16-22-9-11-24(53)12-10-22)46-38(58)31-8-5-15-50(31)41(61)26-13-14-32(54)44-26/h3-4,6-7,9-12,19,21,26-29,31,34-35,52-53H,5,8,13-18,20H2,1-2H3,(H,43,59)(H,44,54)(H,45,55)(H,46,58)(H,47,57)(H,48,60)(H,49,56)(H,62,63)/t26-,27+,28+,29+,31+,34+,35-/m1/s1 |
InChI Key |
IPOLXDNCMOVXCP-WUVUALGCSA-N |
SMILES |
CC(C)C1C(=O)NCC(=O)NC(C(=O)NC(CC2=CN(C(C(=O)N1)NC(=O)C(CC3=CC=C(C=C3)O)NC(=O)C4CCCN4C(=O)C5CCC(=O)N5)C6=CC=CC=C26)C(=O)O)CO |
Isomeric SMILES |
CC(C)[C@H]1C(=O)NCC(=O)N[C@H](C(=O)N[C@@H](CC2=CN([C@H](C(=O)N1)NC(=O)[C@H](CC3=CC=C(C=C3)O)NC(=O)[C@@H]4CCCN4C(=O)[C@H]5CCC(=O)N5)C6=CC=CC=C26)C(=O)O)CO |
Canonical SMILES |
CC(C)C1C(=O)NCC(=O)NC(C(=O)NC(CC2=CN(C(C(=O)N1)NC(=O)C(CC3=CC=C(C=C3)O)NC(=O)C4CCCN4C(=O)C5CCC(=O)N5)C6=CC=CC=C26)C(=O)O)CO |
Origin of Product |
United States |
Retrosynthesis Analysis
AI-Powered Synthesis Planning: Our tool employs the Template_relevance Pistachio, Template_relevance Bkms_metabolic, Template_relevance Pistachio_ringbreaker, Template_relevance Reaxys, Template_relevance Reaxys_biocatalysis model, leveraging a vast database of chemical reactions to predict feasible synthetic routes.
One-Step Synthesis Focus: Specifically designed for one-step synthesis, it provides concise and direct routes for your target compounds, streamlining the synthesis process.
Accurate Predictions: Utilizing the extensive PISTACHIO, BKMS_METABOLIC, PISTACHIO_RINGBREAKER, REAXYS, REAXYS_BIOCATALYSIS database, our tool offers high-accuracy predictions, reflecting the latest in chemical research and data.
Strategy Settings
Precursor scoring | Relevance Heuristic |
---|---|
Min. plausibility | 0.01 |
Model | Template_relevance |
Template Set | Pistachio/Bkms_metabolic/Pistachio_ringbreaker/Reaxys/Reaxys_biocatalysis |
Top-N result to add to graph | 6 |
Feasible Synthetic Routes
Disclaimer and Information on In-Vitro Research Products
Please be aware that all articles and product information presented on BenchChem are intended solely for informational purposes. The products available for purchase on BenchChem are specifically designed for in-vitro studies, which are conducted outside of living organisms. In-vitro studies, derived from the Latin term "in glass," involve experiments performed in controlled laboratory settings using cells or tissues. It is important to note that these products are not categorized as medicines or drugs, and they have not received approval from the FDA for the prevention, treatment, or cure of any medical condition, ailment, or disease. We must emphasize that any form of bodily introduction of these products into humans or animals is strictly prohibited by law. It is essential to adhere to these guidelines to ensure compliance with legal and ethical standards in research and experimentation.