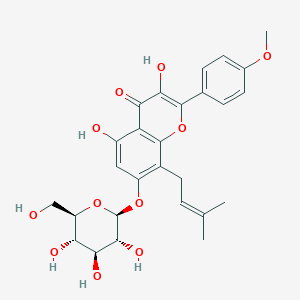
Icariside I
Overview
Description
Icariside I (molecular formula: C₂₆H₂₈O₁₀) is a prenylated flavonol glycoside derived from Epimedium species, a traditional herb known as "Yin Yang Huo" in Chinese medicine. It is a secondary metabolite formed via enzymatic hydrolysis of icariin, involving the removal of a rhamnose group . Structurally, it features a flavonoid backbone with a hydroxylated prenyl group at the C-8 position and a glucose moiety at the C-7 hydroxyl group .
This compound exhibits diverse pharmacological activities, including:
- Anti-tumor effects: Modulates gut microbiota to enhance probiotics (Lactobacillus, Bifidobacterium) and short-chain fatty acids (SCFAs), thereby improving intestinal immunity and inhibiting melanoma growth (IC₅₀ for lipid peroxide scavenging: 8.19 μM) .
- Anti-ferroptosis: Scavenges hydroxyl radicals (˙OH) and reduces lipid peroxidation (LPO) in HT-1080 cells, comparable to the antioxidant Fer-1 .
- Hepatotoxicity risks: Enhances NLRP3 inflammasome activation under ATP/nigericin stimulation, leading to idiosyncratic liver injury in LPS-sensitized mice .
Preparation Methods
Traditional Extraction and Enzymatic Hydrolysis
Beta-Glycosidase-Mediated Conversion
The patent CN106148449A details a benchmark enzymatic method using beta-glycosidase to hydrolyze icariin from Herba Epimedii extracts . Key steps include:
-
Enzyme Digestion : Herba Epimedii extract (≥15% icariin content) is dissolved in a pH 5.0–6.0 citrate-phosphate buffer (5–15 L buffer per kg extract) and reacted with beta-glycosidase (0.2 kg enzyme/kg extract) at 55°C for 6 hours.
-
Purification : The reaction mixture is filtered, and the precipitate undergoes dual ethanol recrystallization (35–60 kg ethanol/kg filter cake), yielding 12–15% this compound with 99% purity .
Critical Parameters :
-
Temperature : Optimal activity occurs at 35–65°C; higher temperatures risk enzyme denaturation.
-
Buffer System : Citrate-phosphate buffers outperform acetic acid or phosphoric acid systems in maintaining enzyme stability .
Table 1: Performance of Beta-Glycosidase Method
Parameter | Value | Source |
---|---|---|
Yield (vs. raw extract) | 15% | |
Purity | 99% | |
Reaction Time | 6 hours | |
Scalability | Industrial-scale compatible |
Microbial Biosynthesis via Engineered Cell Factories
Whole-Cell Catalysis Systems
Recent advances employ recombinant E. coli or S. cerevisiae expressing beta-glycosidase to bypass plant-derived extraction . The process involves:
-
Gene Cloning : Beta-glycosidase genes (e.g., from Thermotoga petrophila) are inserted into microbial vectors.
-
Fermentation : Engineered strains are cultured in bioreactors with icariin-supplemented media, achieving 85–90% icariin-to-icariside I conversion in 24 hours .
Advantages Over Traditional Methods :
-
Yield Enhancement : Microbial systems eliminate reliance on seasonal plant variability.
-
Byproduct Reduction : Targeted hydrolysis minimizes degradation products like 3,5,7-trihydroxyflavone .
Table 2: Microbial vs. Enzymatic Hydrolysis
Metric | Microbial Method | Enzymatic Method |
---|---|---|
Conversion Efficiency | 85–90% | 70–75% |
Time | 24 hours | 6 hours |
Purity | 98% | 99% |
Cost | High (fermentation) | Moderate (enzyme cost) |
Computational Enzyme Design for Enhanced Catalysis
Alpha-L-Rhamnosidase Engineering
The Frontiers study (2022) utilized molecular dynamics simulations and site-directed mutagenesis to optimize Thermotoga petrophila α-L-rhamnosidase (TpeRha) . Key modifications include:
-
Active Site Expansion : Mutants D200G and W208H enlarged the substrate-binding pocket, improving icariin affinity.
-
Catalytic Efficiency : The double mutant D200G/W208H showed a 200-fold increase in compared to wild-type TpeRha .
Implications for Industry :
-
Reduced Enzyme Loading : High-efficiency mutants enable lower enzyme dosages (0.05 kg/kg substrate vs. 0.2 kg/kg in traditional methods) .
-
Thermostability : Engineered enzymes retain >90% activity at 70°C, facilitating continuous processing .
Table 3: Performance of Engineered TpeRha Variants
Variant | (s⁻¹) | (mM) | (mM⁻¹s⁻¹) |
---|---|---|---|
Wild-Type | 0.8 | 4.2 | 0.19 |
D200G | 12.4 | 3.1 | 4.0 |
W208H | 9.7 | 2.8 | 3.5 |
D200G/W208H | 25.6 | 1.9 | 13.5 |
Comparative Analysis of Purification Techniques
Ethanol Recrystallization
Post-hydrolysis purification typically involves:
-
Primary Crystallization : 35–60 kg ethanol per kg filter cake at reflux, achieving 70–80% recovery .
-
Secondary Crystallization : 16–20 kg ethanol/kg, increasing purity from 95% to 99% .
Chromatographic Methods
Though avoided industrially due to cost, preparative HPLC (C18 columns, 70% methanol eluent) can attain >99.5% purity for pharmaceutical applications .
Chemical Reactions Analysis
Production of Icariside I
- From Icariin : this compound can be derived through the enzymatic hydrolysis of Icariin . Cellulase is particularly effective in this process, yielding up to 94.8% this compound . The optimal reaction time for this conversion is around 2.5 hours at temperatures between 37 and 60°C .
- Enzymatic Hydrolysis : α-L-rhamnosidase, an enzyme widely used in the industry, can hydrolyze rhamnosyl-based natural products. Mutants of α-L-rhamnosidases can be used to improve the conversion rate of icariin to this compound .
Reactions of this compound
- Hydrolysis : this compound can undergo further hydrolysis to yield Icaritin. Enzymes such as cellulase, β-glucosidase, and β-dextranase can be used in this reaction .
- Glycosylation : this compound is the glycosylation product of icaritin .
Biological Activities Enhanced by Chemical Reactions
- Anti-Cancer Activity : this compound demonstrates anti-cancer activity, reducing breast cancer proliferation, invasion, and metastasis, potentially by inhibiting the IL-6/STAT3 signaling pathway .
- Estrogen Deficiency Amelioration : As a prenylflavonoid, this compound can effectively ameliorate estrogen deficiency-induced osteoporosis .
- GPCR Regulation and MK Activation : Research indicates that glycosides like Icariside II, which has a sugar linked to the C3- or C7-position, are more efficient than Icaritin itself in D3R agonist action .
Metabolic Transformation
- Intestinal Microbiota Conversion : Icariin can be converted into this compound by intestinal microbiota through hydrolysis of C-3-O-rhamnoside .
Molecular Interactions
- D3R Binding : Molecular docking analysis reveals that Icariside II potentially exerts its agonistic effect through hydrogen-bonding interaction with Asp110 of the D3R .
Data Tables
Table 1: IC50 Values for Icariin, Icariside II, and Icaritin
Compound | hMAO-A | hMAO-B | Lipid Peroxidation |
---|---|---|---|
Icariin | 421.74 ± 7.07 | >500 | 301.03 ± 2.16 |
Icariside II | >500 | >500 | 70.38 ± 2.04 |
Icaritin | >500 | >500 | 81.33 ± 3.09 |
R-(–)-deprenyl HCl | 17.41 ± 0.75 | 0.23 ± 0.02 | ND |
Trolox | ND | ND | 32.33 ± 3.21 |
(IC50 values in μM, ND = Not detected)
Table 2: α-L-Rhamnosidase Mutants and Conversion Rates
Conversion Rate (Icariin to this compound) | |
---|---|
Wild-type TpeRha | 4.33% ± 0.19% |
R369A | 38.37% ± 1.57% |
D506A | 13.70% ± 1.93% |
H570A | 63.65% ± 3.96% |
K579A | 46.29% ± 1.78% |
DH (D506A/H570A) | 88.56% ± 0.06% |
DK (D506A/K579A) | 70.38% ± 0.13% |
HK (H570A/K579A) | 86.78% ± 0.90% |
DHK (D506A/H570A/K579A) | 93.32% ± 0.09% |
Scientific Research Applications
Chemistry: It serves as a precursor for synthesizing other bioactive compounds.
Biology: Icariside I has been shown to modulate various biological pathways, making it a valuable tool for studying cellular processes.
Medicine: It exhibits anti-cancer properties by inhibiting the proliferation, apoptosis, invasion, and metastasis of cancer cells. .
Mechanism of Action
Icariside I exerts its effects through multiple mechanisms:
Molecular Targets: It targets the IL-6/STAT3 signaling pathway, which is crucial for cancer cell proliferation and metastasis.
Pathways Involved: The compound modulates various pathways, including the STAT3 pathway, which is involved in cell survival and proliferation, and the cGAS-STING pathway, which is crucial for immune response
Comparison with Similar Compounds
Structural and Functional Differences
Table 1: Structural and Pharmacological Comparison of Icariside I and Analogues
Pharmacological Efficacy
Anti-Ferroptosis vs. Antioxidant Activity
- This compound reduces LPO levels by 60–70% in erastin/RSL3-induced ferroptosis, outperforming icaritin and icariside II in hydroxyl radical scavenging .
- Icariin and icaritin show weaker anti-ferroptosis effects due to lower membrane permeability (lack of optimal glycosylation) .
Immunomodulation
- This compound uniquely upregulates Lactobacillus and Bifidobacterium abundance, enhancing SCFA production (↑ acetate, propionate by 2.5-fold) and CD4+/CD8+ T-cell activity .
- Icariside II lacks significant gut microbiota modulation but directly inhibits tyrosinase in melanocytes .
Hepatotoxicity Mechanisms
- This compound synergizes with LPS to increase ALT/AST levels (↑ 300%) and IL-1β production via mtROS-dependent NLRP3 activation .
- Icariside II and baohuoside II induce oxidative stress (↑ ROS by 50%) and mitochondrial damage in HL-7702 cells, independent of inflammasome pathways .
Table 2: Toxicity Comparison in Hepatic Models
Biological Activity
Icariside I, a flavonoid glycoside derived from the traditional medicinal herb Epimedium, has garnered attention for its diverse biological activities. This article provides a comprehensive overview of its pharmacological effects, mechanisms of action, and potential therapeutic applications based on recent research findings.
Chemical Structure and Metabolism
This compound is primarily formed through the metabolism of icariin by intestinal microbiota, which hydrolyzes specific glycosidic bonds. The compound is characterized by its rhamnoside and glucopyranoside moieties, which influence its bioactivity and pharmacokinetics. Studies indicate that after oral administration, a significant portion of icariin is converted into this compound, enhancing its biological effectiveness compared to its parent compound .
NLRP3 Inflammasome Activation
Recent studies have highlighted the role of this compound in modulating the NLRP3 inflammasome, a critical component of the immune response. Research indicates that this compound enhances NLRP3 inflammasome activation in response to specific stimuli like ATP and nigericin but does not affect other inflammasome pathways such as NLRC4 or AIM2. This selective activation leads to increased production of pro-inflammatory cytokines like IL-1β and promotes pyroptosis in macrophages .
Table 1: Effects of this compound on NLRP3 Inflammasome Activation
Stimulus | Effect of this compound | Mechanism |
---|---|---|
ATP | Increased IL-1β secretion | Enhances caspase-1 cleavage |
Nigericin | Promotes pyroptosis | Increases LDH release |
SiO2 | No effect | No alteration in caspase-1 activity |
Cytosolic LPS | No effect | No change in pro-caspase-1 expression |
Anti-Cancer Properties
This compound has shown potential anti-cancer activity across various cancer models. It has been observed to inhibit tumor growth by affecting multiple signaling pathways involved in cell proliferation and apoptosis. For instance, studies have demonstrated that it reduces the expression of Ki-67 (a proliferation marker) and modulates key proteins such as Raf, MEK, and ERK, which are integral to cancer cell survival and proliferation .
Case Study: Tumor Growth Inhibition
In a mouse model study, administration of this compound resulted in:
- Reduction in tumor size : Tumors were significantly smaller in treated groups compared to controls.
- Decreased proliferation markers : A notable reduction in Ki-67 expression was recorded.
Immunomodulatory Effects
This compound also exhibits immunomodulatory properties. In experiments involving cyclophosphamide-induced immunosuppression in mice, treatment with this compound restored normal peripheral blood cell counts and improved thymus and spleen indices. This suggests its potential role in enhancing immune function and recovery from immunosuppressive conditions .
Pharmacokinetic Profile
The pharmacokinetics of this compound indicate favorable absorption and distribution characteristics post-administration. Studies report that it reaches peak plasma concentrations rapidly after oral intake, with significant bioavailability attributed to its metabolic conversion from icariin .
Safety and Toxicity
While the therapeutic potential of this compound is promising, safety assessments are crucial. Current literature suggests that it may lead to liver injury under certain conditions, particularly when combined with inflammatory stimuli like LPS. However, pre-treatment with specific inhibitors (e.g., MCC950) has shown protective effects against such injuries .
Q & A
Basic Research Questions
Q. What is the chemical structure and natural source of Icariside I, and how does it differ from related Epimedium-derived flavonoids?
this compound (C27H30O11) is a deglycosylated metabolite of icariin, a prenylated flavonol glycoside found in Epimedium species. It is formed by removing the rhamnose residue from icariin, distinguishing it structurally from icariside II (glucose removal) and icaritin (complete deglycosylation) . Its low natural abundance in Epimedium complicates isolation, necessitating synthetic derivatization or enzymatic modification for research use .
Q. What are the primary anti-tumor mechanisms of this compound identified in preclinical models?
this compound inhibits tumor growth via apoptosis induction (evidenced by nuclear condensation and Ki67 suppression), immune modulation (e.g., CD8+ T cell infiltration), and gut microbiota regulation. In B16F10 melanoma models, a medium dose (20 mg/kg) achieved a 51.72% tumor inhibition rate, outperforming higher/lower doses . Mechanistically, it suppresses IL-6/JAK2/STAT3 signaling and enhances SCFA production via microbiota modulation, linking metabolic and immune pathways .
Q. What experimental models are commonly used to study this compound’s anti-tumor effects, and what endpoints are prioritized?
The B16F10 melanoma mouse model is widely used, with endpoints including tumor volume/weight, histopathology (e.g., necrosis via H&E staining), and immune profiling (flow cytometry for CD3+/CD8+ T cells) . In vitro studies often focus on apoptosis (Annexin V/PI assays) and cytokine profiling (ELISA for IL-1β, IL-6) . Dose-response experiments (5–80 mg/kg) are critical due to its nonlinear efficacy .
Advanced Research Questions
Q. How does this compound modulate gut microbiota composition and metabolite production in cancer models, and what methodological approaches validate these interactions?
this compound restores tumor-induced dysbiosis by increasing probiotics (e.g., Lactobacillus, Bifidobacterium) and SCFAs (acetate, butyrate) via 16S rRNA sequencing and targeted metabolomics. Key steps:
- α/β-diversity analysis : Bray-Curtis PCoA to confirm structural shifts in microbiota .
- SCFA quantification : GC-MS or LC-MS for fecal metabolites, correlating with immune markers (Spearman’s rank) .
- Functional validation : Gnotobiotic models to isolate microbiota contributions .
Q. How can researchers reconcile contradictory data on this compound’s hepatotoxicity and therapeutic efficacy?
While this compound shows anti-tumor activity, it exacerbates NLRP3 inflammasome activation (via mtROS) in LPS-primed models, causing idiosyncratic hepatotoxicity (elevated ALT/AST) . Mitigation strategies:
- Dose optimization : Use subtoxic thresholds (e.g., ≤20 mg/kg) .
- Co-treatment assays : Test NLRP3 inhibitors (e.g., MCC950) to isolate toxicity pathways .
- Biomarker monitoring : Track IL-1β/TNF-α alongside liver function markers in preclinical trials .
Q. What methodologies optimize the study of this compound’s dose-dependent responses and pharmacokinetic variability?
- Pharmacokinetic profiling : LC-MS/MS to measure plasma half-life and tissue distribution, noting nonlinear absorption at high doses .
- Dynamic dosing : Use adaptive designs (e.g., Bayesian models) to adjust doses based on real-time tumor volume data .
- Combinatorial screens : Pair this compound with checkpoint inhibitors (e.g., anti-PD-1) to assess synergy in immune-cold tumors .
Q. How can researchers standardize this compound quality control across studies, given its structural similarity to other Epimedium flavonoids?
- HPLC-DAD/MS : Multi-index quantitative fingerprinting to distinguish this compound from icariin/icaritin, using retention times and UV spectra .
- Hierarchical clustering analysis (HCA) : Statistically validate batch-to-batch consistency in commercial/extracted samples .
- Reference standards : Use phyproof® substances (≥98% purity) for calibration .
Q. Methodological Considerations for Future Studies
- Immune profiling : Combine single-cell RNA-seq with CyTOF to map tumor microenvironment changes .
- Microbiota transplants : Fecal transfers from this compound-treated mice to germ-free models to confirm causality .
- Toxicity screens : Include organoid models (e.g., liver-on-a-chip) to predict human hepatotoxicity .
Properties
IUPAC Name |
3,5-dihydroxy-2-(4-methoxyphenyl)-8-(3-methylbut-2-enyl)-7-[(2S,3R,4S,5S,6R)-3,4,5-trihydroxy-6-(hydroxymethyl)oxan-2-yl]oxychromen-4-one | |
---|---|---|
Source | PubChem | |
URL | https://pubchem.ncbi.nlm.nih.gov | |
Description | Data deposited in or computed by PubChem | |
InChI |
InChI=1S/C27H30O11/c1-12(2)4-9-15-17(36-27-24(34)22(32)20(30)18(11-28)37-27)10-16(29)19-21(31)23(33)25(38-26(15)19)13-5-7-14(35-3)8-6-13/h4-8,10,18,20,22,24,27-30,32-34H,9,11H2,1-3H3/t18-,20-,22+,24-,27-/m1/s1 | |
Source | PubChem | |
URL | https://pubchem.ncbi.nlm.nih.gov | |
Description | Data deposited in or computed by PubChem | |
InChI Key |
IYCPMVXIUPYNHI-WPKKLUCLSA-N | |
Source | PubChem | |
URL | https://pubchem.ncbi.nlm.nih.gov | |
Description | Data deposited in or computed by PubChem | |
Canonical SMILES |
CC(=CCC1=C(C=C(C2=C1OC(=C(C2=O)O)C3=CC=C(C=C3)OC)O)OC4C(C(C(C(O4)CO)O)O)O)C | |
Source | PubChem | |
URL | https://pubchem.ncbi.nlm.nih.gov | |
Description | Data deposited in or computed by PubChem | |
Isomeric SMILES |
CC(=CCC1=C(C=C(C2=C1OC(=C(C2=O)O)C3=CC=C(C=C3)OC)O)O[C@H]4[C@@H]([C@H]([C@@H]([C@H](O4)CO)O)O)O)C | |
Source | PubChem | |
URL | https://pubchem.ncbi.nlm.nih.gov | |
Description | Data deposited in or computed by PubChem | |
Molecular Formula |
C27H30O11 | |
Source | PubChem | |
URL | https://pubchem.ncbi.nlm.nih.gov | |
Description | Data deposited in or computed by PubChem | |
DSSTOX Substance ID |
DTXSID30205283 | |
Record name | Baohuoside-1 | |
Source | EPA DSSTox | |
URL | https://comptox.epa.gov/dashboard/DTXSID30205283 | |
Description | DSSTox provides a high quality public chemistry resource for supporting improved predictive toxicology. | |
Molecular Weight |
530.5 g/mol | |
Source | PubChem | |
URL | https://pubchem.ncbi.nlm.nih.gov | |
Description | Data deposited in or computed by PubChem | |
CAS No. |
56725-99-6 | |
Record name | Icariside I | |
Source | CAS Common Chemistry | |
URL | https://commonchemistry.cas.org/detail?cas_rn=56725-99-6 | |
Description | CAS Common Chemistry is an open community resource for accessing chemical information. Nearly 500,000 chemical substances from CAS REGISTRY cover areas of community interest, including common and frequently regulated chemicals, and those relevant to high school and undergraduate chemistry classes. This chemical information, curated by our expert scientists, is provided in alignment with our mission as a division of the American Chemical Society. | |
Explanation | The data from CAS Common Chemistry is provided under a CC-BY-NC 4.0 license, unless otherwise stated. | |
Record name | Baohuoside-1 | |
Source | ChemIDplus | |
URL | https://pubchem.ncbi.nlm.nih.gov/substance/?source=chemidplus&sourceid=0056725996 | |
Description | ChemIDplus is a free, web search system that provides access to the structure and nomenclature authority files used for the identification of chemical substances cited in National Library of Medicine (NLM) databases, including the TOXNET system. | |
Record name | Baohuoside-1 | |
Source | EPA DSSTox | |
URL | https://comptox.epa.gov/dashboard/DTXSID30205283 | |
Description | DSSTox provides a high quality public chemistry resource for supporting improved predictive toxicology. | |
Retrosynthesis Analysis
AI-Powered Synthesis Planning: Our tool employs the Template_relevance Pistachio, Template_relevance Bkms_metabolic, Template_relevance Pistachio_ringbreaker, Template_relevance Reaxys, Template_relevance Reaxys_biocatalysis model, leveraging a vast database of chemical reactions to predict feasible synthetic routes.
One-Step Synthesis Focus: Specifically designed for one-step synthesis, it provides concise and direct routes for your target compounds, streamlining the synthesis process.
Accurate Predictions: Utilizing the extensive PISTACHIO, BKMS_METABOLIC, PISTACHIO_RINGBREAKER, REAXYS, REAXYS_BIOCATALYSIS database, our tool offers high-accuracy predictions, reflecting the latest in chemical research and data.
Strategy Settings
Precursor scoring | Relevance Heuristic |
---|---|
Min. plausibility | 0.01 |
Model | Template_relevance |
Template Set | Pistachio/Bkms_metabolic/Pistachio_ringbreaker/Reaxys/Reaxys_biocatalysis |
Top-N result to add to graph | 6 |
Feasible Synthetic Routes
Disclaimer and Information on In-Vitro Research Products
Please be aware that all articles and product information presented on BenchChem are intended solely for informational purposes. The products available for purchase on BenchChem are specifically designed for in-vitro studies, which are conducted outside of living organisms. In-vitro studies, derived from the Latin term "in glass," involve experiments performed in controlled laboratory settings using cells or tissues. It is important to note that these products are not categorized as medicines or drugs, and they have not received approval from the FDA for the prevention, treatment, or cure of any medical condition, ailment, or disease. We must emphasize that any form of bodily introduction of these products into humans or animals is strictly prohibited by law. It is essential to adhere to these guidelines to ensure compliance with legal and ethical standards in research and experimentation.