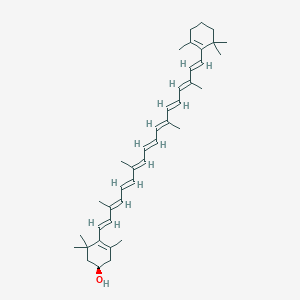
beta-Cryptoxanthin
Overview
Description
β-Cryptoxanthin is an oxygenated xanthophyll carotenoid found abundantly in citrus fruits, papaya, and pumpkin . Structurally, it contains a hydroxyl group, distinguishing it from hydrocarbon carotenes like β-carotene. It exists in both free and esterified forms in plants, with its bioavailability in human diets exceeding that of other major carotenoids such as β-carotene and lutein . β-Cryptoxanthin serves as a provitamin A source and exhibits unique biological activities, including anti-inflammatory, antioxidant, and bone health-promoting properties .
Preparation Methods
Plant-Based Extraction Methods
Solvent Extraction and Crystallization
The US9771323B2 patent outlines a method for isolating beta-cryptoxanthin crystals from plant matrices, prioritizing paprika and citrus peels . The process involves:
-
Pretreatment : Raw materials are dried (40–60°C) and ground to ≤500 µm particles.
-
Solvent Extraction : A hexane:ethanol (3:1 v/v) mixture refluxed at 60°C for 4 hours liberates carotenoids, yielding a 12–15% crude extract.
-
Crystallization : The extract is cooled to −20°C, inducing this compound crystal formation with 89–93% purity .
Optimization Insights :
-
Particle size reduction (<300 µm) increases extraction efficiency by 22% .
-
Saponification (0.5 M KOH in methanol, 60°C, 1 hour) removes esterified lipids, boosting purity to 95% .
Enzyme-Assisted Extraction from Physalis alkekengi
The CN102219721B patent details a multi-step extraction from Physalis alkekengi calyx, achieving 18 mg/g this compound content :
Step | Parameters | Outcome |
---|---|---|
Enzyme Treatment | Cellulase (50 U/g), pH 5.0, 50°C, 2 hours | Cell wall hydrolysis increases yield by 37% |
Ethanol Extraction | 70% ethanol, 1:15 solid:liquid ratio, 60°C, 3 hours | 94% carotenoid recovery |
Saponification | 0.1 M NaOH, 50°C, 30 minutes | Lipid removal without carotenoid degradation |
This method reduces processing time by 40% compared to conventional techniques .
Microbial Fermentation via Engineered Yarrowia lipolytica
Metabolic Engineering Strategies
A 2024 study identified Chondromyces crocatus β-carotene hydroxylase (CcBCH) as a critical enzyme for converting β-carotene to this compound . Key modifications in Y. lipolytica include:
-
Gene Insertion : Heterologous expression of CrtZ (β-carotene hydroxylase) and CrtE (geranylgeranyl diphosphate synthase).
-
Subcellular Targeting : Localizing CcBCH to peroxisomes increased zeaxanthin production by 66%, while ER targeting enhanced this compound titer to 24 mg/L .
Fermentation Parameters :
Condition | Value | Impact |
---|---|---|
pH | 6.0 | Optimal enzyme activity |
Temperature | 28°C | Maximizes biomass yield |
Oxygenation | 30% DO | Prevents carotenoid oxidation |
Challenges in Microbial Production
-
Low Conversion Rates : Only 28% of β-carotene is hydroxylated to this compound due to competing pathways .
-
Cost Implications : Media costs account for 60% of total expenses, necessitating cheaper carbon sources (e.g., glycerol).
Nanoliposomal Encapsulation for Enhanced Stability
Thin-Film Hydration Technique
Gharib et al. (2015) developed nanoliposomal this compound using a phosphatidylcholine:cholesterol (7:3 molar ratio) film hydrated with 10 mM PBS (pH 7.4) .
Parameter | Value | Outcome |
---|---|---|
Sonication Time | 15 minutes | Reduces particle size to 85 nm |
Encapsulation Efficiency | 92% | Maintains bioactivity during storage |
Stability Analysis :
Impact on Leukemia Cell Lines
Nanoliposomal this compound induced 48% apoptosis in K562 cells at 50 µM, compared to 22% for free carotenoid, attributed to enhanced cellular uptake .
Emerging Techniques: Air-Assisted Liquid-Liquid Microextraction
A 2016 study introduced a green extraction method using 1-dodecanol as a low-density solvent :
-
Emulsification : 5 mL juice + 1 mL ethanol + 100 µL 1-dodecanol, vortexed for 30 seconds.
-
Solidification : Cooling at −20°C for 10 minutes separates the organic phase.
Performance Metrics :
Metric | Value |
---|---|
Recovery Rate | 98.2% |
LOD | 0.02 µg/mL |
This method reduces solvent use by 90% compared to conventional techniques .
Chemical Reactions Analysis
Types of Reactions
Cryptoxanthin undergoes various chemical reactions, including oxidation, reduction, and substitution reactions.
Common Reagents and Conditions
Oxidation: Cryptoxanthin can be oxidized using reagents such as potassium permanganate or chromium trioxide under acidic conditions.
Reduction: Reduction of cryptoxanthin can be achieved using hydrogen gas in the presence of a palladium catalyst.
Substitution: Substitution reactions can occur with halogens or other nucleophiles, often under basic conditions.
Major Products Formed
The major products formed from these reactions include various oxidized or reduced derivatives of cryptoxanthin, which can have different biological activities and properties .
Scientific Research Applications
Nutritional Applications
Source of Vitamin A:
β-Cryptoxanthin is recognized as a precursor to vitamin A, contributing to the body's retinol levels. Studies indicate that it has a higher bioavailability compared to other carotenoids like β-carotene and α-carotene, making it an effective source of this essential nutrient in the diet . Foods rich in β-cryptoxanthin include tangerines, red peppers, and pumpkins.
Dietary Impact:
Research shows that β-cryptoxanthin-rich diets are associated with lower risks of degenerative diseases. For instance, a study highlighted that regular consumption of β-cryptoxanthin can lead to improved bone health and a reduced risk of osteoporosis due to its anabolic effects on bone metabolism .
Preventive Health Applications
Antioxidant Properties:
As a potent antioxidant, β-cryptoxanthin plays a crucial role in combating oxidative stress, which is linked to various chronic diseases including cancer and cardiovascular diseases. Its ability to scavenge free radicals contributes to its protective effects against cellular damage .
Non-Alcoholic Fatty Liver Disease:
Recent studies have demonstrated that β-cryptoxanthin can significantly improve conditions related to non-alcoholic fatty liver disease (NAFLD). It enhances insulin sensitivity and reduces hepatic lipid accumulation by modulating metabolic pathways such as SIRT1 activity. This suggests potential therapeutic applications in managing NAFLD and related metabolic disorders .
Cancer Prevention
Epidemiological Evidence:
Observational studies have indicated an inverse relationship between β-cryptoxanthin intake and the risk of certain cancers, particularly lung cancer. The compound's antioxidant properties are believed to play a role in mitigating cancer risk by reducing oxidative damage to DNA .
Mechanistic Insights:
In vitro studies show that β-cryptoxanthin influences cell signaling pathways associated with cancer progression. For example, it has been observed to inhibit the proliferation of cancer cells and induce apoptosis through various molecular mechanisms .
Case Studies
Mechanism of Action
Cryptoxanthin exerts its effects through several mechanisms:
Antioxidant Activity: Cryptoxanthin acts as an antioxidant, neutralizing free radicals and preventing oxidative damage to cells and DNA.
Provitamin A Activity: Cryptoxanthin is converted into vitamin A (retinol) in the human body, which is essential for vision, immune function, and skin health.
Molecular Targets and Pathways: Cryptoxanthin interacts with various molecular targets and pathways, including the beta-carotene 15,15’-oxygenase 1 (BCO1) enzyme, which cleaves cryptoxanthin to form retinal.
Comparison with Similar Compounds
Comparative Analysis with Similar Carotenoids
Chemical Structure and Classification
Compound | Type | Structural Features | Solubility |
---|---|---|---|
β-Cryptoxanthin | Xanthophyll | Oxygenated, hydroxyl group at C3 | Lipid-soluble |
β-Carotene | Carotene | Hydrocarbon chain with two β-ionone rings | Lipid-soluble |
Lutein | Xanthophyll | Hydroxyl groups at C3 and C3' | Lipid-soluble |
Astaxanthin | Xanthophyll | Ketone groups at C4 and C4', hydroxyl groups | Lipid-soluble |
Lycopene | Carotene | Acyclic hydrocarbon chain | Lipid-soluble |
Key Differences :
- β-Cryptoxanthin’s hydroxyl group enhances its antioxidant capacity compared to β-carotene .
- Unlike lutein and zeaxanthin, β-cryptoxanthin acts as a retinoic acid receptor (RAR) ligand, albeit with lower affinity than all-trans retinoic acid .
Bioavailability and Absorption
Research Findings :
- β-Cryptoxanthin esters exhibit higher stability and bioaccessibility than free forms .
- A study in rats showed β-cryptoxanthin’s bioavailability was 72.5% higher than β-carotene .
Health Benefits and Mechanisms
Bone Health
- β-Cryptoxanthin : Stimulates osteoblast activity, inhibits osteoclast-mediated bone resorption, and shows seasonal synergy with vitamin D .
Cancer Prevention
- β-Cryptoxanthin : Reduces lung cancer risk by upregulating RARβ and suppressing cyclin D1/E in cancer cells .
Antioxidant Capacity
- β-Cryptoxanthin : Quenches singlet oxygen (SOAC value: 1,200 µmol/g) and repairs DNA oxidation damage .
- Astaxanthin : Superior singlet oxygen quenching (SOAC: 2,800 µmol/g) but lower bioavailability .
Dietary Sources
Biological Activity
β-Cryptoxanthin is a xanthophyll carotenoid predominantly found in various fruits, particularly citrus fruits and red peppers. It has garnered attention for its potential health benefits, including antioxidant properties and roles in disease prevention, particularly in relation to cancer and metabolic disorders. This article reviews the biological activities of β-cryptoxanthin, focusing on its mechanisms of action, clinical studies, and implications for human health.
Anticancer Properties
β-Cryptoxanthin has shown promising results in inhibiting cancer cell growth and inducing apoptosis in various cancer models. Research indicates that it may exert its anticancer effects through several mechanisms:
- Cell Cycle Regulation : A study demonstrated that β-cryptoxanthin inhibits the growth of non-small-cell lung cancer cells (A549) and immortalized bronchial epithelial cells (BEAS-2B) by modulating cell cycle proteins. It reduces cyclin D1 and cyclin E levels while increasing p21 expression, leading to a G1/G0 phase arrest in the cell cycle .
- Retinoic Acid Receptor Activation : β-Cryptoxanthin has been shown to up-regulate retinoic acid receptor β (RARβ) expression, which is associated with anti-proliferative effects in lung cancer cells . This receptor's activation is crucial for mediating the effects of retinoids in cancer prevention.
- Synergistic Effects with Chemotherapy : In combination with oxaliplatin, β-cryptoxanthin enhances the drug's antitumoral activity by down-regulating ΔNP73, a variant involved in tumor progression . This suggests that β-cryptoxanthin may be beneficial as an adjunct therapy in cancer treatment.
Metabolic Syndrome and Fatty Liver Disease
Recent studies have highlighted the role of β-cryptoxanthin in metabolic health:
- Fat Reduction and Oxidative Stress Protection : In vivo studies using Caenorhabditis elegans models indicated that low doses of β-cryptoxanthin significantly reduce body fat and enhance oxidative stress resistance. These findings suggest its potential therapeutic applications for metabolic syndrome .
- Liver Health : β-Cryptoxanthin supplementation has been linked to reduced hepatic lipid accumulation and inflammation, showing protective effects against hepatocellular carcinoma progression. This effect appears to be independent of its cleavage by carotenoid enzymes BCO1/BCO2, indicating a direct action on liver metabolism .
Case Studies and Trials
Several clinical trials have investigated the safety and efficacy of β-cryptoxanthin supplementation:
- A randomized controlled trial involving 90 healthy women assessed the pharmacokinetics of β-cryptoxanthin over eight weeks. Results showed significant increases in plasma concentrations without adverse effects, indicating good tolerability .
- Observational studies have reported inverse associations between plasma levels of β-cryptoxanthin and risks of various cancers, including lung cancer, type 2 diabetes, and non-alcoholic fatty liver disease (NAFLD) .
Data Summary
Q & A
Basic Research Questions
Q. What experimental methods are used to encapsulate beta-cryptoxanthin in nanoliposomes, and how is encapsulation efficiency quantified?
this compound is encapsulated using the extrusion method: soy lecithin and cholesterol (4:1 molar ratio) are dissolved in chloroform, dried into a lipid film, and hydrated with this compound solution. Post-sonication and extrusion through 100 nm membranes, ultracentrifugation separates free compounds from encapsulated ones . Encapsulation efficiency (~86.3%) is quantified via HPLC by comparing the amount entrapped in nanoliposomes to the initial input . Particle size, zeta potential, and polydispersity index are measured using dynamic light scattering (Malvern Zetasizer), while cryo-TEM confirms spherical morphology .
Q. Which in vitro assays are standard for evaluating this compound’s anti-proliferative effects in cancer studies?
The MTT assay is widely used to assess anti-proliferative activity by measuring mitochondrial activity in cells (e.g., K562 leukemia cells). Apoptosis is quantified via flow cytometry using Annexin V/PI staining to distinguish early/late apoptotic and necrotic cells . Dose- and time-dependent responses (e.g., 10–100 µg/mL over 24–72 hours) are tested, with statistical significance determined via ANOVA (p < 0.05) . Hoechst 33342 staining further visualizes nuclear fragmentation in apoptotic cells .
Q. How is this compound quantified in biological samples, and what analytical challenges exist?
Reverse-phase HPLC with a C18 column and UV detection (mobile phase: ethanol/acetonitrile + 0.1% diethylamine) is standard for quantifying this compound in nanoliposomes or plasma . Challenges include low bioavailability due to lipophilicity and esterification in blood, requiring sample pretreatment (e.g., Triton X-100 lysis for nanoliposomes) . Plasma-dermal correlation studies show weak associations (r=0.07), highlighting variability in tissue distribution .
Advanced Research Questions
Q. What mechanisms explain the enhanced anti-cancer efficacy of nanoliposomal this compound versus its free form?
Nanoliposomes improve water dispersibility and cellular uptake via membrane fusion, enabling higher intracellular concentrations. In K562 cells, encapsulated this compound induces 2–3× greater apoptosis than free forms (p < 0.001) by enhancing nuclear delivery and sustained release . Cryo-TEM confirms structural stability, while zeta potential (-25 mV) prevents aggregation, prolonging circulation time . Synergy with other carotenoids (e.g., lycopene) may also amplify pro-apoptotic pathways like caspase-3 activation .
Q. How do researchers reconcile discrepancies in epidemiological data on this compound’s cancer-preventive effects?
Cohort studies show a 24% reduced lung cancer risk per 10 µg/day intake (p < 0.001) , but plasma-dermal correlations are non-significant (p=0.72) . Contradictions arise from variability in dietary sources, bioavailability (e.g., esterified vs. free forms), and confounders like smoking. Adjusting for vitamin C intake (common in this compound-rich foods like oranges) attenuates associations, suggesting synergistic antioxidant effects . Preclinical models (e.g., nicotine-exposed mice) clarify dose-dependent mechanisms, such as SIRT1 upregulation and NF-κB inhibition .
Q. What strategies optimize this compound delivery in vivo for bone health and anti-inflammatory applications?
Esterified this compound (e.g., from red fruit oil) shows enhanced absorption and bone-specific accumulation by binding to retinoic acid receptors (RARs), promoting osteoblast activity . In inflammatory polyarthritis studies, daily intake equivalent to 1 glass of orange juice (≥365 µg) reduces risk (OR=0.42, p < 0.05) by suppressing IL-6 and TNF-α . Nanoemulsions with fatty acids (e.g., palmitate) improve thermal stability and bioavailability in animal models .
Q. Methodological Considerations
Q. How are dose-response relationships established in this compound studies?
Preclinical models use escalating doses (e.g., 10–100 µg/mL in vitro; 0.5–2 mg/kg in mice) to identify IC50 values and therapeutic windows . Human trials derive intake thresholds from dietary records (e.g., tertile analysis in EPIC-Norfolk: >365 µg/day vs. <185 µg/day) . Synergy with vitamin A precursors (e.g., retinol) is controlled via multivariate regression to isolate this compound-specific effects .
Q. What experimental designs address this compound’s instability in aqueous environments?
Encapsulation in nanoliposomes or lipid-based carriers (e.g., soy lecithin) prevents oxidative degradation . In vitro release studies use dialysis membranes (8 kDa cutoff) to simulate physiological conditions, showing 84.1% stability at 37°C over 12 hours . For long-term storage, lyophilization with cryoprotectants (e.g., trehalose) maintains entrapment efficacy .
Q. Data Interpretation Challenges
Q. How do researchers differentiate this compound’s direct effects from confounding dietary factors?
Mendelian randomization and adjusted cohort analyses control for covariates (e.g., smoking, total energy intake) . Cell-specific assays (e.g., siRNA knockdown of RARs) isolate molecular pathways, while isotopic tracing (e.g., 13C-labeled this compound) tracks metabolic fate in vivo .
Q. What gaps exist in translating preclinical this compound findings to clinical trials?
Limited human pharmacokinetic data and variability in carotenoid metabolism complicate dosing. Phase I trials prioritize esterified formulations for improved bioavailability, while biomarker-driven designs (e.g., serum SIRT1 levels) validate mechanistic hypotheses from animal models .
Properties
IUPAC Name |
(1R)-3,5,5-trimethyl-4-[(1E,3E,5E,7E,9E,11E,13E,15E,17E)-3,7,12,16-tetramethyl-18-(2,6,6-trimethylcyclohexen-1-yl)octadeca-1,3,5,7,9,11,13,15,17-nonaenyl]cyclohex-3-en-1-ol | |
---|---|---|
Source | PubChem | |
URL | https://pubchem.ncbi.nlm.nih.gov | |
Description | Data deposited in or computed by PubChem | |
InChI |
InChI=1S/C40H56O/c1-30(18-13-20-32(3)23-25-37-34(5)22-15-27-39(37,7)8)16-11-12-17-31(2)19-14-21-33(4)24-26-38-35(6)28-36(41)29-40(38,9)10/h11-14,16-21,23-26,36,41H,15,22,27-29H2,1-10H3/b12-11+,18-13+,19-14+,25-23+,26-24+,30-16+,31-17+,32-20+,33-21+/t36-/m1/s1 | |
Source | PubChem | |
URL | https://pubchem.ncbi.nlm.nih.gov | |
Description | Data deposited in or computed by PubChem | |
InChI Key |
DMASLKHVQRHNES-FKKUPVFPSA-N | |
Source | PubChem | |
URL | https://pubchem.ncbi.nlm.nih.gov | |
Description | Data deposited in or computed by PubChem | |
Canonical SMILES |
CC1=C(C(CCC1)(C)C)C=CC(=CC=CC(=CC=CC=C(C)C=CC=C(C)C=CC2=C(CC(CC2(C)C)O)C)C)C | |
Source | PubChem | |
URL | https://pubchem.ncbi.nlm.nih.gov | |
Description | Data deposited in or computed by PubChem | |
Isomeric SMILES |
CC1=C(C(CCC1)(C)C)/C=C/C(=C/C=C/C(=C/C=C/C=C(\C)/C=C/C=C(\C)/C=C/C2=C(C[C@H](CC2(C)C)O)C)/C)/C | |
Source | PubChem | |
URL | https://pubchem.ncbi.nlm.nih.gov | |
Description | Data deposited in or computed by PubChem | |
Molecular Formula |
C40H56O | |
Source | PubChem | |
URL | https://pubchem.ncbi.nlm.nih.gov | |
Description | Data deposited in or computed by PubChem | |
DSSTOX Substance ID |
DTXSID30912309 | |
Record name | Cryptoxanthin | |
Source | EPA DSSTox | |
URL | https://comptox.epa.gov/dashboard/DTXSID30912309 | |
Description | DSSTox provides a high quality public chemistry resource for supporting improved predictive toxicology. | |
Molecular Weight |
552.9 g/mol | |
Source | PubChem | |
URL | https://pubchem.ncbi.nlm.nih.gov | |
Description | Data deposited in or computed by PubChem | |
Physical Description |
Solid | |
Record name | beta-Cryptoxanthin | |
Source | Human Metabolome Database (HMDB) | |
URL | http://www.hmdb.ca/metabolites/HMDB0033844 | |
Description | The Human Metabolome Database (HMDB) is a freely available electronic database containing detailed information about small molecule metabolites found in the human body. | |
Explanation | HMDB is offered to the public as a freely available resource. Use and re-distribution of the data, in whole or in part, for commercial purposes requires explicit permission of the authors and explicit acknowledgment of the source material (HMDB) and the original publication (see the HMDB citing page). We ask that users who download significant portions of the database cite the HMDB paper in any resulting publications. | |
CAS No. |
472-70-8 | |
Record name | Cryptoxanthin | |
Source | CAS Common Chemistry | |
URL | https://commonchemistry.cas.org/detail?cas_rn=472-70-8 | |
Description | CAS Common Chemistry is an open community resource for accessing chemical information. Nearly 500,000 chemical substances from CAS REGISTRY cover areas of community interest, including common and frequently regulated chemicals, and those relevant to high school and undergraduate chemistry classes. This chemical information, curated by our expert scientists, is provided in alignment with our mission as a division of the American Chemical Society. | |
Explanation | The data from CAS Common Chemistry is provided under a CC-BY-NC 4.0 license, unless otherwise stated. | |
Record name | Cryptoxanthin | |
Source | ChemIDplus | |
URL | https://pubchem.ncbi.nlm.nih.gov/substance/?source=chemidplus&sourceid=0000472708 | |
Description | ChemIDplus is a free, web search system that provides access to the structure and nomenclature authority files used for the identification of chemical substances cited in National Library of Medicine (NLM) databases, including the TOXNET system. | |
Record name | Cryptoxanthin | |
Source | DrugBank | |
URL | https://www.drugbank.ca/drugs/DB15914 | |
Description | The DrugBank database is a unique bioinformatics and cheminformatics resource that combines detailed drug (i.e. chemical, pharmacological and pharmaceutical) data with comprehensive drug target (i.e. sequence, structure, and pathway) information. | |
Explanation | Creative Common's Attribution-NonCommercial 4.0 International License (http://creativecommons.org/licenses/by-nc/4.0/legalcode) | |
Record name | Cryptoxanthin | |
Source | EPA DSSTox | |
URL | https://comptox.epa.gov/dashboard/DTXSID30912309 | |
Description | DSSTox provides a high quality public chemistry resource for supporting improved predictive toxicology. | |
Record name | CRYPTOXANTHIN | |
Source | FDA Global Substance Registration System (GSRS) | |
URL | https://gsrs.ncats.nih.gov/ginas/app/beta/substances/6ZIB13GI33 | |
Description | The FDA Global Substance Registration System (GSRS) enables the efficient and accurate exchange of information on what substances are in regulated products. Instead of relying on names, which vary across regulatory domains, countries, and regions, the GSRS knowledge base makes it possible for substances to be defined by standardized, scientific descriptions. | |
Explanation | Unless otherwise noted, the contents of the FDA website (www.fda.gov), both text and graphics, are not copyrighted. They are in the public domain and may be republished, reprinted and otherwise used freely by anyone without the need to obtain permission from FDA. Credit to the U.S. Food and Drug Administration as the source is appreciated but not required. | |
Record name | beta-Cryptoxanthin | |
Source | Human Metabolome Database (HMDB) | |
URL | http://www.hmdb.ca/metabolites/HMDB0033844 | |
Description | The Human Metabolome Database (HMDB) is a freely available electronic database containing detailed information about small molecule metabolites found in the human body. | |
Explanation | HMDB is offered to the public as a freely available resource. Use and re-distribution of the data, in whole or in part, for commercial purposes requires explicit permission of the authors and explicit acknowledgment of the source material (HMDB) and the original publication (see the HMDB citing page). We ask that users who download significant portions of the database cite the HMDB paper in any resulting publications. | |
Melting Point |
172 - 173 °C | |
Record name | beta-Cryptoxanthin | |
Source | Human Metabolome Database (HMDB) | |
URL | http://www.hmdb.ca/metabolites/HMDB0033844 | |
Description | The Human Metabolome Database (HMDB) is a freely available electronic database containing detailed information about small molecule metabolites found in the human body. | |
Explanation | HMDB is offered to the public as a freely available resource. Use and re-distribution of the data, in whole or in part, for commercial purposes requires explicit permission of the authors and explicit acknowledgment of the source material (HMDB) and the original publication (see the HMDB citing page). We ask that users who download significant portions of the database cite the HMDB paper in any resulting publications. | |
Retrosynthesis Analysis
AI-Powered Synthesis Planning: Our tool employs the Template_relevance Pistachio, Template_relevance Bkms_metabolic, Template_relevance Pistachio_ringbreaker, Template_relevance Reaxys, Template_relevance Reaxys_biocatalysis model, leveraging a vast database of chemical reactions to predict feasible synthetic routes.
One-Step Synthesis Focus: Specifically designed for one-step synthesis, it provides concise and direct routes for your target compounds, streamlining the synthesis process.
Accurate Predictions: Utilizing the extensive PISTACHIO, BKMS_METABOLIC, PISTACHIO_RINGBREAKER, REAXYS, REAXYS_BIOCATALYSIS database, our tool offers high-accuracy predictions, reflecting the latest in chemical research and data.
Strategy Settings
Precursor scoring | Relevance Heuristic |
---|---|
Min. plausibility | 0.01 |
Model | Template_relevance |
Template Set | Pistachio/Bkms_metabolic/Pistachio_ringbreaker/Reaxys/Reaxys_biocatalysis |
Top-N result to add to graph | 6 |
Feasible Synthetic Routes
Disclaimer and Information on In-Vitro Research Products
Please be aware that all articles and product information presented on BenchChem are intended solely for informational purposes. The products available for purchase on BenchChem are specifically designed for in-vitro studies, which are conducted outside of living organisms. In-vitro studies, derived from the Latin term "in glass," involve experiments performed in controlled laboratory settings using cells or tissues. It is important to note that these products are not categorized as medicines or drugs, and they have not received approval from the FDA for the prevention, treatment, or cure of any medical condition, ailment, or disease. We must emphasize that any form of bodily introduction of these products into humans or animals is strictly prohibited by law. It is essential to adhere to these guidelines to ensure compliance with legal and ethical standards in research and experimentation.