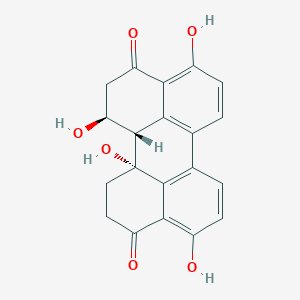
Altertoxin I
Overview
Description
Altertoxin I (ATX-I; C₂₀H₁₆O₆, molecular weight 352.34 g/mol) is a perylenequinone mycotoxin produced by Alternaria species, notorious for contaminating fruits, grains, and vegetables . Structurally, it features a dihydroperylenequinone core with two epoxide groups, distinguishing it from other altertoxins (e.g., ATX-II, ATX-III) . ATX-I exhibits mutagenic and genotoxic properties, primarily through DNA intercalation and oxidative stress induction . Its stability in acidic environments (e.g., 75% remaining in pH 3 buffer after two weeks) and persistence in fruit juices underscore its relevance to food safety .
Preparation Methods
Biosynthesis of Altertoxin I in Alternaria alternata
ATX-I is predominantly biosynthesized through fungal cultivation, leveraging the polyketide pathway of Alternaria alternata. Key studies have utilized modified Czapek-Dox medium to maximize yield, with glucose and ammonium sulfate serving as primary carbon and nitrogen sources .
Culture Medium Composition
The standard protocol involves inoculating A. alternata conidia into a sterile medium containing:
-
Glucose : 4–8 g/L
-
Ammonium sulfate : 0.27 g/L
-
Trace elements : Citric acid, ZnSO₄·6H₂O, Fe₂(NH₄)₂(SO₄)₂·6H₂O, CuSO₄·5H₂O, MnSO₄, H₃BO₃, and Na₂MoO₄·6H₂O .
Optimal pH is adjusted to 5.5 to mimic natural fungal habitats. Sterilization at 121°C for 20 minutes ensures aseptic conditions prior to inoculation .
Incubation and Harvesting
Cultures are incubated at 26°C for 7–9 days under continuous shaking (110 rpm) . Post-incubation, the biomass is homogenized and extracted with dichloromethane (DCM) or DCM-methanol (1:1), yielding a crude extract rich in ATX-I and related toxins .
Table 1: ATX-I Biosynthesis Yields Under Optimized Conditions
Parameter | Value | Yield (µg/g dry biomass) | Source |
---|---|---|---|
Glucose concentration | 4 g/L | 1.1 | |
Ammonium sulfate | 0.27 g/L | 1.1 | |
Incubation period | 7 days | 0.9 | |
Shaking speed | 110 rpm | 1.1 |
Extraction and Purification Strategies
Solvent Extraction
Crude homogenates are subjected to sequential solvent extraction:
-
Primary extraction : DCM (2×100 mL, 6 hours) for non-polar metabolites .
-
Secondary extraction : Ethyl acetate (2×20 mL) for medium-polarity compounds .
-
Defatting : Pentane (3×6 mL) removes lipids prior to chromatographic purification .
Chromatographic Purification
Preparative high-performance liquid chromatography (HPLC) with acetonitrile-water gradients (50:50 to 84:16 v/v) achieves >99% purity . Recent advances employ supercritical fluid chromatography (SFC) for higher resolution, utilizing CO₂-methanol gradients on a Torus 1-Aminoanthracene column .
Table 2: ATX-I Recovery Rates Across Purification Methods
Optimization of Culture Conditions
Carbon and Nitrogen Modulation
Glucose concentration directly impacts polyketide synthase activity, with 4 g/L yielding 1.1 mg ATX-I per g dry biomass . Substituting nitrate with ammonium sulfate enhances toxin production by 40%, likely due to improved nitrogen assimilation .
Isotopic Labeling for Analytical Standards
[¹³C₂₀]-ATX-I synthesis uses [¹³C₆]glucose, achieving 100% isotopic enrichment in the polyketide backbone. Natural ¹²C incorporation from trace elements results in minor isotopologues (e.g., [¹³C₁₈¹²C₂] at 40% abundance) .
Analytical Validation and Quantification
Liquid Chromatography-Tandem Mass Spectrometry (LC-MS/MS)
ATX-I is quantified using reverse-phase C18 columns with electrospray ionization (ESI⁻). Key transitions include m/z 353→297 (quantifier) and 353→265 (qualifier) . Internal standards like [¹³C₂₀]-ATX-I correct for matrix effects in food samples .
Challenges in Stability Assessment
ATX-I degrades <10% over 72 hours in acetonitrile-water (50:50) at 4°C, whereas stemphyltoxin III (a structural analog) shows 90% degradation under identical conditions .
Chemical Reactions Analysis
Types of Reactions: Altertoxin I undergoes various chemical reactions, including oxidation, reduction, and substitution.
Common Reagents and Conditions:
Oxidation: Typically involves reagents like hydrogen peroxide or potassium permanganate under acidic or basic conditions.
Reduction: Commonly uses reagents such as sodium borohydride or lithium aluminum hydride.
Substitution: Often involves nucleophiles like halides or amines under conditions that facilitate the substitution reaction.
Major Products: The major products formed from these reactions depend on the specific conditions and reagents used. For example, oxidation may yield hydroxylated derivatives, while reduction could produce reduced forms of the toxin.
Scientific Research Applications
Altertoxin I has several scientific research applications:
Chemistry: Used as a model compound to study the synthesis and reactivity of perylenequinone derivatives.
Biology: Investigated for its mutagenic and cytotoxic effects on bacterial and mammalian cells.
Medicine: Studied for its potential role in carcinogenicity and its interactions with cellular pathways.
Industry: Monitored as a contaminant in food safety studies to ensure the safety of agricultural products.
Mechanism of Action
Altertoxin I exerts its effects through multiple mechanisms. It has been shown to inhibit casein kinase 2, a ubiquitous enzyme involved in various cellular processes . This inhibition can influence estrogen receptor transcription and DNA-binding affinity, potentially leading to estrogenic effects . Additionally, this compound can interact with the nuclear factor erythroid-derived 2-like 2/antioxidant response element pathway, which is involved in the cellular response to oxidative stress .
Comparison with Similar Compounds
Comparative Analysis with Similar Compounds
Structural Comparisons
The altertoxin family comprises over 14 variants, differing in ring systems, oxygenation, and substituents (Table 1). Key structural distinctions include:
- ATX-I : Two fused rings with two epoxide groups.
- ATX-III : A tri-epoxidized derivative with higher polarity .
Table 1. Structural and Physicochemical Properties of Select Altertoxins
Cytotoxicity and Mechanisms of Action
In Vitro Cytotoxicity
- ATX-I : Weak cytotoxicity (IC₅₀ > 5 µg/mL in V79 lung cells) but disrupts metabolic communication at high doses (4–5 µg/mL) .
- ATX-II : Potent cytotoxicity (IC₅₀ = 0.002–0.02 µg/mL in Ewing sarcoma cells), inducing DNA double-strand breaks and S-phase arrest .
- ATX-III : Intermediate potency (IC₅₀ = 0.04–0.2 µg/mL) .
Table 2. Cytotoxic Effects in Model Systems
Enzyme Inhibition
- ATX-I : Inhibits human topoisomerase IIα (Topo II) and bacterial gyrase at high concentrations (potency rank: 7th among 15 Alternaria toxins) .
- ATX-II : Strong Topo II inhibition (4th in potency), contributing to anti-cancer activity .
Metabolic and Environmental Fate
Biological Activity
Altertoxin I (ATX-I) is a mycotoxin produced by the fungus Alternaria tenuissima and is part of a group of compounds known for their diverse biological activities. This article provides a comprehensive overview of the biological activity of ATX-I, including its cytotoxic effects, mechanisms of action, and potential therapeutic applications.
Chemical Structure and Properties
This compound is characterized by its unique chemical structure, which contributes to its biological activity. It is an amorphous solid that melts at approximately 180 °C and exhibits fluorescence under UV light. Its structural features include a perylenequinone backbone, which is common among other Alternaria toxins.
ATX-I has been shown to exhibit significant cytotoxic effects against various cell lines. The following table summarizes key findings regarding its cytotoxicity:
Cell Line | IC50 (µM) | Mechanism of Action |
---|---|---|
HCT116 (human colorectal cancer) | 3.13 | Induction of apoptosis |
V79 (Chinese hamster cells) | >5 | Cell cycle arrest |
MG-63 (human osteosarcoma) | 14.81 | Inhibition of mitochondrial function |
SMMC-7721 (hepatocellular carcinoma) | 22.87 | Disruption of cellular metabolism |
These findings indicate that ATX-I can induce apoptosis in cancer cell lines and disrupt normal cellular functions, suggesting potential as an anti-cancer agent .
Anti-HIV Activity
ATX-I has also demonstrated anti-HIV properties. Research indicates that it can inhibit HIV-1 replication in infected cells, with a notable IC50 value of 0.5 µM for complete inhibition. This suggests that ATX-I may be a candidate for further development as an anti-HIV therapeutic .
Estrogen Receptor Modulation
Emerging studies have indicated that ATX-I may interact with estrogen receptors, influencing their transcriptional activity. Specifically, it has been shown to increase the nuclear localization of estrogen receptor alpha (ERα) in certain cell types, which could have implications for hormone-related cancers . However, the exact mechanisms behind this modulation remain to be fully elucidated.
Immunosuppressive Effects
ATX-I has been implicated in immunosuppressive effects, potentially affecting immune cell function. This property raises concerns regarding its safety in food products, as exposure could lead to adverse health effects . Further research is necessary to understand the implications of these findings on human health.
Case Studies and Toxicological Assessments
A review of case studies indicates that ATX-I is present in various food products, including fruits and vegetables contaminated by Alternaria species. The detection of ATX-I in food samples underscores the need for ongoing monitoring and risk assessment to evaluate its impact on human health .
Q & A
Basic Research Questions
Q. What analytical techniques are most effective for identifying and quantifying Altertoxin I in complex matrices (e.g., food, biological samples)?
- Methodological Answer : High-performance liquid chromatography coupled with tandem mass spectrometry (HPLC-MS/MS) is widely used due to its sensitivity in detecting perylene quinones like this compound at trace levels. For structural confirmation, nuclear magnetic resonance (NMR) spectroscopy is essential to resolve its polycyclic aromatic structure, particularly distinguishing it from analogs like Altertoxin II or III . Challenges include low recovery rates in extraction protocols, necessitating optimization with solid-phase extraction (SPE) columns and solvent systems (e.g., ethyl acetate/methanol mixtures) .
Q. How does this compound’s chemical structure influence its stability under varying environmental conditions?
- Methodological Answer : this compound’s perylene quinone backbone makes it photosensitive. Stability studies should include controlled light exposure experiments (e.g., UV-Vis irradiation) paired with LC-MS to monitor degradation products like epoxidized derivatives. Its susceptibility to hydrolysis at alkaline pH (e.g., >8.0) requires buffered extraction conditions to prevent artifactual transformations during sample preparation .
Q. What are the primary fungal species known to produce this compound, and how does its biosynthesis pathway compare to other Alternaria toxins?
- Methodological Answer : Alternaria alternata and Penicillium spp. are key producers. Biosynthetic studies using isotope-labeled precursors (e.g., acetate or mevalonate) reveal that this compound derives from the polyketide pathway, diverging from alternariol biosynthesis at the cyclization step. Gene cluster comparisons (e.g., PKS and oxidoreductase genes) between fungal strains can clarify pathway variations .
Advanced Research Questions
Q. How can researchers resolve contradictions in reported bioavailability data for this compound in mammalian systems?
- Methodological Answer : Discrepancies in recovery rates (e.g., low fecal/urinary excretion in rats vs. in vitro absorption models) may stem from enzymatic de-epoxidation of Altertoxin II to this compound in vivo. To address this, use isotope-labeled this compound in pharmacokinetic studies and employ metabolomics workflows (e.g., HRMS/MS) to track biotransformation products across matrices (plasma, urine, feces) .
Q. What experimental designs are critical for elucidating this compound’s toxicological mechanisms, particularly its genotoxic potential?
- Methodological Answer : Combine Ames tests (with/without metabolic activation) to assess mutagenicity and comet assays to evaluate DNA strand breaks. For mechanistic depth, use transcriptomics (RNA-seq) in exposed cell lines (e.g., HepG2) to identify oxidative stress pathways linked to quinone redox cycling. Dose-response studies must account for its low solubility in aqueous media by using carriers like DMSO (≤0.1% v/v) to avoid solvent interference .
Q. How can researchers optimize in vitro models to study this compound’s interaction with gut microbiota?
- Methodological Answer : Simulated gut fermentation systems (e.g., batch cultures with fecal inocula) paired with metagenomic sequencing can identify microbial taxa capable of detoxifying or bioactivating this compound. Include controls with broad-spectrum antibiotics to distinguish microbial vs. host metabolism. Quantify metabolites using targeted LC-MS and correlate shifts with microbial diversity indices .
Q. Methodological Considerations for Reproducibility
- Sample Preparation : Document solvent ratios, SPE cartridge brands (e.g., C18 vs. HLB), and centrifugation speeds to ensure consistency in mycotoxin recovery .
- Data Reporting : Adhere to the Beilstein Journal of Organic Chemistry guidelines: provide raw NMR spectra (δ values, coupling constants) and HPLC chromatograms in supplementary materials. For novel compounds, include elemental analysis and high-resolution mass spectrometry (HRMS) data .
Properties
IUPAC Name |
(12S,12aS,12bR)-4,9,12,12b-tetrahydroxy-2,11,12,12a-tetrahydro-1H-perylene-3,10-dione | |
---|---|---|
Source | PubChem | |
URL | https://pubchem.ncbi.nlm.nih.gov | |
Description | Data deposited in or computed by PubChem | |
InChI |
InChI=1S/C20H16O6/c21-10-3-1-8-9-2-4-11(22)17-12(23)5-6-20(26,18(9)17)19-14(25)7-13(24)16(10)15(8)19/h1-4,14,19,21-22,25-26H,5-7H2/t14-,19+,20-/m0/s1 | |
Source | PubChem | |
URL | https://pubchem.ncbi.nlm.nih.gov | |
Description | Data deposited in or computed by PubChem | |
InChI Key |
GJIALGLHOBXNAT-KPOBHBOGSA-N | |
Source | PubChem | |
URL | https://pubchem.ncbi.nlm.nih.gov | |
Description | Data deposited in or computed by PubChem | |
Canonical SMILES |
C1CC2(C3C(CC(=O)C4=C(C=CC(=C34)C5=C2C(=C(C=C5)O)C1=O)O)O)O | |
Source | PubChem | |
URL | https://pubchem.ncbi.nlm.nih.gov | |
Description | Data deposited in or computed by PubChem | |
Isomeric SMILES |
C1C[C@]2([C@@H]3[C@H](CC(=O)C4=C(C=CC(=C34)C5=C2C(=C(C=C5)O)C1=O)O)O)O | |
Source | PubChem | |
URL | https://pubchem.ncbi.nlm.nih.gov | |
Description | Data deposited in or computed by PubChem | |
Molecular Formula |
C20H16O6 | |
Source | PubChem | |
URL | https://pubchem.ncbi.nlm.nih.gov | |
Description | Data deposited in or computed by PubChem | |
DSSTOX Substance ID |
DTXSID40971689 | |
Record name | 1,4,9,12a-Tetrahydroxy-1,2,11,12,12a,12b-hexahydroperylene-3,10-dione | |
Source | EPA DSSTox | |
URL | https://comptox.epa.gov/dashboard/DTXSID40971689 | |
Description | DSSTox provides a high quality public chemistry resource for supporting improved predictive toxicology. | |
Molecular Weight |
352.3 g/mol | |
Source | PubChem | |
URL | https://pubchem.ncbi.nlm.nih.gov | |
Description | Data deposited in or computed by PubChem | |
CAS No. |
56258-32-3 | |
Record name | Altertoxin I | |
Source | CAS Common Chemistry | |
URL | https://commonchemistry.cas.org/detail?cas_rn=56258-32-3 | |
Description | CAS Common Chemistry is an open community resource for accessing chemical information. Nearly 500,000 chemical substances from CAS REGISTRY cover areas of community interest, including common and frequently regulated chemicals, and those relevant to high school and undergraduate chemistry classes. This chemical information, curated by our expert scientists, is provided in alignment with our mission as a division of the American Chemical Society. | |
Explanation | The data from CAS Common Chemistry is provided under a CC-BY-NC 4.0 license, unless otherwise stated. | |
Record name | Altertoxin I | |
Source | ChemIDplus | |
URL | https://pubchem.ncbi.nlm.nih.gov/substance/?source=chemidplus&sourceid=0056258323 | |
Description | ChemIDplus is a free, web search system that provides access to the structure and nomenclature authority files used for the identification of chemical substances cited in National Library of Medicine (NLM) databases, including the TOXNET system. | |
Record name | 1,4,9,12a-Tetrahydroxy-1,2,11,12,12a,12b-hexahydroperylene-3,10-dione | |
Source | EPA DSSTox | |
URL | https://comptox.epa.gov/dashboard/DTXSID40971689 | |
Description | DSSTox provides a high quality public chemistry resource for supporting improved predictive toxicology. | |
Record name | (12S,12aS,12bR)-4,9,12,12b-tetrahydroxy-2,11,12,12a-tetrahydro-1H-perylene-3,10-dione | |
Source | European Chemicals Agency (ECHA) | |
URL | https://echa.europa.eu/information-on-chemicals | |
Description | The European Chemicals Agency (ECHA) is an agency of the European Union which is the driving force among regulatory authorities in implementing the EU's groundbreaking chemicals legislation for the benefit of human health and the environment as well as for innovation and competitiveness. | |
Explanation | Use of the information, documents and data from the ECHA website is subject to the terms and conditions of this Legal Notice, and subject to other binding limitations provided for under applicable law, the information, documents and data made available on the ECHA website may be reproduced, distributed and/or used, totally or in part, for non-commercial purposes provided that ECHA is acknowledged as the source: "Source: European Chemicals Agency, http://echa.europa.eu/". Such acknowledgement must be included in each copy of the material. ECHA permits and encourages organisations and individuals to create links to the ECHA website under the following cumulative conditions: Links can only be made to webpages that provide a link to the Legal Notice page. | |
Retrosynthesis Analysis
AI-Powered Synthesis Planning: Our tool employs the Template_relevance Pistachio, Template_relevance Bkms_metabolic, Template_relevance Pistachio_ringbreaker, Template_relevance Reaxys, Template_relevance Reaxys_biocatalysis model, leveraging a vast database of chemical reactions to predict feasible synthetic routes.
One-Step Synthesis Focus: Specifically designed for one-step synthesis, it provides concise and direct routes for your target compounds, streamlining the synthesis process.
Accurate Predictions: Utilizing the extensive PISTACHIO, BKMS_METABOLIC, PISTACHIO_RINGBREAKER, REAXYS, REAXYS_BIOCATALYSIS database, our tool offers high-accuracy predictions, reflecting the latest in chemical research and data.
Strategy Settings
Precursor scoring | Relevance Heuristic |
---|---|
Min. plausibility | 0.01 |
Model | Template_relevance |
Template Set | Pistachio/Bkms_metabolic/Pistachio_ringbreaker/Reaxys/Reaxys_biocatalysis |
Top-N result to add to graph | 6 |
Feasible Synthetic Routes
Disclaimer and Information on In-Vitro Research Products
Please be aware that all articles and product information presented on BenchChem are intended solely for informational purposes. The products available for purchase on BenchChem are specifically designed for in-vitro studies, which are conducted outside of living organisms. In-vitro studies, derived from the Latin term "in glass," involve experiments performed in controlled laboratory settings using cells or tissues. It is important to note that these products are not categorized as medicines or drugs, and they have not received approval from the FDA for the prevention, treatment, or cure of any medical condition, ailment, or disease. We must emphasize that any form of bodily introduction of these products into humans or animals is strictly prohibited by law. It is essential to adhere to these guidelines to ensure compliance with legal and ethical standards in research and experimentation.