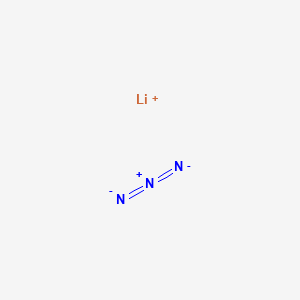
Lithium azide
Overview
Description
Lithium azide (LiN₃) is an inorganic compound composed of lithium cations (Li⁺) and azide anions (N₃⁻). It has a molecular mass of 48.96 g/mol (monoisotopic mass: 49.025) and a CAS number of 19597-69-4 . LiN₃ is typically synthesized via metathesis reactions, such as between sodium azide (NaN₃) and lithium sulfate (Li₂SO₄), or by reacting barium azide (Ba(N₃)₂) with lithium salts . It decomposes upon heating to release nitrogen gas (N₂) and lithium metal, making it a reactive compound with applications in synthetic chemistry and materials science.
Preparation Methods
Metathesis Reaction with Sodium Azide and Lithium Nitrate
Reaction Equation
The simplest method involves the metathesis of sodium azide (NaN₃) and lithium nitrate (LiNO₃) in aqueous solution:
Procedure
-
Reactant Dissolution : Equimolar quantities of NaN₃ and LiNO₃ are dissolved in distilled water at ambient temperature.
-
Precipitation : The high solubility of LiN₃ (66.4 g/100 mL at 16°C) ensures it remains in solution, while NaNO₃ precipitates due to its lower solubility (87.6 g/100 mL at 20°C).
-
Filtration and Crystallization : The mixture is filtered to remove NaNO₃, and the filtrate is evaporated under reduced pressure to yield LiN₃ crystals.
Yield and Purity
Considerations
-
Safety : NaN₃ is highly toxic; reactions must be conducted in a fume hood.
-
Scalability : Suitable for large-scale synthesis but generates stoichiometric NaNO₃ waste .
Metathesis Reaction with Sodium Azide and Lithium Sulfate
Reaction Equation
This alternative metathesis employs lithium sulfate (Li₂SO₄) to enhance yield:
Procedure
-
Stoichiometric Mixing : A 2:1 molar ratio of NaN₃ to Li₂SO₄ is dissolved in water.
-
Selective Precipitation : Na₂SO₄ precipitates due to its low solubility (47.6 g/100 mL at 25°C), leaving LiN₃ in solution.
-
Isolation : The solution is filtered, and LiN₃ is recovered via rotary evaporation.
Yield and Purity
Considerations
Reaction with Barium Azide and Lithium Sulfate
Reaction Equation
Barium azide (Ba(N₃)₂) reacts with Li₂SO₄ to produce LiN₃ and insoluble BaSO₄:
Procedure
-
Precipitation Reaction : Equimolar Ba(N₃)₂ and Li₂SO₄ are mixed in aqueous solution.
-
Filtration : BaSO₄ is removed via vacuum filtration.
-
Crystallization : The filtrate is concentrated and cooled to 10°C to precipitate LiN₃.
Yield and Purity
Considerations
Neutralization of Lithium Carbonate with Hydrazoic Acid
Reaction Equation
High-purity LiN₃ is obtained by neutralizing lithium carbonate (Li₂CO₃) with hydrazoic acid (HN₃):
Procedure
-
Acid Addition : HN₃ gas is bubbled into a suspension of Li₂CO₃ in anhydrous ethanol.
-
Gas Evolution : CO₂ is released, monitored by mass loss.
-
Drying : The resulting LiN₃ is dried under vacuum at 80°C.
Yield and Purity
Considerations
Solvent-Mediated Synthesis from Lithium Sulfate Monohydrate
Reaction Equation
A modified metathesis using lithium sulfate monohydrate (Li₂SO₄·H₂O) and NaN₃ in aqueous-alcoholic media:
Procedure
-
Dissolution : NaN₃ (91.3 g) and Li₂SO₄·H₂O (1.41 g) are dissolved in 7 mL water at 35°C.
-
Alcohol Addition : 35 mL of 96% ethanol is added to induce Na₂SO₄ precipitation.
-
Filtration and Evaporation : The mixture is filtered, and the filtrate is evaporated to dryness.
-
Recrystallization : Crude LiN₃ is digested in ethanol at 35°C and dried at 80°C.
Yield and Purity
Comparative Analysis of Preparation Methods
Method | Reactants | Solvent | Yield (%) | Purity (%) | Key Advantage | Limitation |
---|---|---|---|---|---|---|
NaN₃ + LiNO₃ | NaN₃, LiNO₃ | Water | >90 | 95 | Simplicity | NaNO₃ waste generation |
NaN₃ + Li₂SO₄ | NaN₃, Li₂SO₄ | Water | 85–92 | 90 | Cost-effective | Sulfate impurities |
Ba(N₃)₂ + Li₂SO₄ | Ba(N₃)₂, Li₂SO₄ | Water | 75–80 | 98 | Sodium-free product | Hazardous Ba(N₃)₂ handling |
Li₂CO₃ + HN₃ | Li₂CO₃, HN₃ | Ethanol | 95–98 | 99.9 | Ultra-high purity | HN₃ toxicity |
Li₂SO₄·H₂O + NaN₃ | Li₂SO₄·H₂O, NaN₃ | Water/Ethanol | 70–75 | 99.5 | Scalable for labs | Low yield per batch |
Chemical Reactions Analysis
Lithium azide undergoes various chemical reactions, including:
Decomposition: When heated, this compound decomposes into lithium and nitrogen gas.
Nucleophilic Substitution: The azide ion (N3-) is a strong nucleophile and can participate in nucleophilic substitution reactions, particularly with alkyl halides, to form alkyl azides.
Cycloaddition Reactions: Azides can participate in [3+2] cycloaddition reactions to form triazoles.
Scientific Research Applications
Lithium azide has several applications in scientific research:
High-Pressure Studies: This compound is used in high-pressure studies to explore new stable nitrogen-rich phases and potential insulator-metal transitions.
Synthesis of Heterocycles: Organic azides, including this compound, are used in the synthesis of various heterocyclic compounds, such as pyrroles, pyrazoles, and triazoles.
Flame Retardants: Diphenylphosphoryl azide, a derivative of this compound, is used as a multifunctional flame retardant additive in lithium-ion batteries.
Mechanism of Action
The mechanism of action of lithium azide primarily involves its decomposition into lithium and nitrogen gas when heated. This decomposition reaction is highly exothermic and can be utilized in various applications requiring rapid gas generation . Additionally, the azide ion’s strong nucleophilicity allows it to participate in various chemical reactions, forming new compounds and intermediates .
Comparison with Similar Compounds
Comparison with Structurally Similar Azides
Alkali Metal Azides
Lithium azide belongs to the family of alkali metal azides, which include sodium azide (NaN₃), potassium azide (KN₃), rubidium azide (RbN₃), and caesium azide (CsN₃). Key comparative properties are summarized in Table 1.
Table 1: Comparative Properties of Alkali Metal Azides
*Decomposition temperature for LiN₃ is approximate due to its instability compared to heavier azides.
- Thermal Stability : this compound is less thermally stable than heavier alkali metal azides. For example, NaN₃ decomposes at ~300°C, whereas LiN₃ decomposes at significantly lower temperatures (~100°C) .
- Explosive Behavior : While NaN₃ and KN₃ are widely used in explosive applications (e.g., automotive airbags), LiN₃ is less commonly employed due to its lower stability and sensitivity .
- Synthetic Utility : LiN₃ is primarily used in organic synthesis to introduce azide groups, whereas NaN₃ is favored in industrial-scale applications due to its cost-effectiveness and stability .
Comparison with Lead Azide (Pb(N₃)₂)
Lead azide (Pb(N₃)₂) is a heavy metal azide with distinct properties:
- Explosive Sensitivity : Pb(N₃)₂ is a primary explosive, sensitive to friction and shock, whereas LiN₃ and NaN₃ require higher energy inputs for detonation .
- Electronic Structure : Ab initio studies reveal that Pb(N₃)₂ has a more complex band structure compared to LiN₃, contributing to its superior explosive performance .
- Applications : Pb(N₃)₂ is used in detonators, while LiN₃ is relegated to niche laboratory applications due to safety concerns .
Comparison with Functionally Similar Lithium Compounds
Lithium Nitride (Li₃N)
Li₃N, another lithium-nitrogen compound, differs significantly from LiN₃:
- Structure and Bonding : Li₃N has a hexagonal crystal structure with covalent Li-N bonds, whereas LiN₃ consists of ionic Li⁺ and N₃⁻ ions .
- Applications : Li₃N is used in solid-state electrolytes for lithium-ion batteries and hydrogen storage, while LiN₃ lacks significant energy storage applications due to its instability .
- Thermodynamics : Li₃N is stable under ambient conditions, whereas LiN₃ decomposes readily upon heating .
Q & A
Q. Basic: What are the standard synthesis methods for lithium azide, and how can product purity be validated?
This compound is typically synthesized via metathesis reactions , such as combining sodium azide (NaN₃) with lithium sulfate (Li₂SO₄) or lithium nitrate (LiNO₃) in aqueous solutions. For example:
Li₂SO₄ + 2NaN₃ → 2LiN₃ + Na₂SO₄
Key methodological considerations :
- Use stoichiometric excess of NaN₃ to ensure complete reaction .
- Conduct reactions under inert atmospheres (e.g., argon) to avoid unintended decomposition .
- Validate purity via X-ray diffraction (XRD) for crystallinity, FTIR spectroscopy for N₃⁻ vibrational modes (~2100 cm⁻¹), and elemental analysis (Li and N content) .
Q. Basic: What safety protocols are critical when handling this compound in laboratory settings?
LiN₃ is highly toxic (T+ classification) and decomposes explosively at temperatures >115°C. Essential protocols include:
- Personal protective equipment (PPE) : Lab coat, nitrile gloves, and face shield.
- Storage : Airtight containers in dry, cool environments; avoid contact with acids (releases toxic HN₃ gas) .
- Waste disposal : Neutralize with dilute Fe(NO₃)₃ to convert azides to non-explosive iron complexes .
Q. Basic: How does this compound’s solubility in water vary with temperature, and what implications does this have for experimental design?
LiN₃ exhibits high water solubility:
Q. Advanced: How can ab initio methods (e.g., VASP) model this compound’s electronic structure and decomposition pathways?
Computational workflow :
Basis set : Use plane-wave pseudopotentials with a cutoff energy ≥400 eV for accurate electron density modeling .
Exchange-correlation functional : Opt for hybrid functionals (e.g., HSE06) to account for bandgap underestimation in DFT .
Convergence criteria : Ensure forces <0.01 eV/Å and energy differences <1×10⁻⁵ eV/atom in geometry optimizations .
Decomposition analysis : Calculate activation energy barriers (NEB method) for LiN₃ → Li + 3N₂ reactions to predict thermal stability .
Q. Advanced: How should researchers resolve contradictions in experimental data (e.g., decomposition temperatures reported as 115°C vs. 298°C)?
Discrepancies often arise from:
- Sample purity : Impurities (e.g., residual NaN₃) lower decomposition onset .
- Heating rate : Fast heating (>10°C/min) may mask intermediate phases.
Methodological solutions : - Perform thermogravimetric analysis (TGA) at multiple heating rates (1–20°C/min) under inert gas.
- Cross-validate with mass spectrometry (MS) to detect N₂ evolution peaks .
Q. Advanced: What strategies optimize experimental design for studying this compound’s reactivity without triggering decomposition?
- Low-temperature techniques : Use cryostatic reactors (<0°C) for reactions with acids or oxidizers.
- In situ monitoring : Pair Raman spectroscopy with pressure sensors to detect HN₃ gas formation .
- Microfluidic systems : Minimize sample mass (<10 mg) to reduce explosion risks during thermal studies .
Q. Advanced: How can computational and experimental results be integrated to predict this compound’s solid-state properties?
Band structure validation : Compare DFT-predicted bandgaps (e.g., ~3.5 eV for LiN₃ ) with experimental UV-Vis absorption edges.
Phonon dispersion : Use density functional perturbation theory (DFPT) to simulate vibrational modes and match with experimental IR/Raman spectra .
Diffraction refinement : Employ Rietveld analysis of XRD patterns, guided by computational lattice parameters (e.g., orthorhombic Pnma symmetry ).
Q. Advanced: What statistical frameworks are recommended for analyzing uncertainties in this compound’s thermodynamic measurements?
Properties
IUPAC Name |
lithium;azide | |
---|---|---|
Source | PubChem | |
URL | https://pubchem.ncbi.nlm.nih.gov | |
Description | Data deposited in or computed by PubChem | |
InChI |
InChI=1S/Li.N3/c;1-3-2/q+1;-1 | |
Source | PubChem | |
URL | https://pubchem.ncbi.nlm.nih.gov | |
Description | Data deposited in or computed by PubChem | |
InChI Key |
GUWHRJQTTVADPB-UHFFFAOYSA-N | |
Source | PubChem | |
URL | https://pubchem.ncbi.nlm.nih.gov | |
Description | Data deposited in or computed by PubChem | |
Canonical SMILES |
[Li+].[N-]=[N+]=[N-] | |
Source | PubChem | |
URL | https://pubchem.ncbi.nlm.nih.gov | |
Description | Data deposited in or computed by PubChem | |
Molecular Formula |
LiN3 | |
Source | PubChem | |
URL | https://pubchem.ncbi.nlm.nih.gov | |
Description | Data deposited in or computed by PubChem | |
DSSTOX Substance ID |
DTXSID8066509 | |
Record name | Lithium azide (Li(N3)) | |
Source | EPA DSSTox | |
URL | https://comptox.epa.gov/dashboard/DTXSID8066509 | |
Description | DSSTox provides a high quality public chemistry resource for supporting improved predictive toxicology. | |
Molecular Weight |
49.0 g/mol | |
Source | PubChem | |
URL | https://pubchem.ncbi.nlm.nih.gov | |
Description | Data deposited in or computed by PubChem | |
CAS No. |
19597-69-4 | |
Record name | Lithium azide | |
Source | CAS Common Chemistry | |
URL | https://commonchemistry.cas.org/detail?cas_rn=19597-69-4 | |
Description | CAS Common Chemistry is an open community resource for accessing chemical information. Nearly 500,000 chemical substances from CAS REGISTRY cover areas of community interest, including common and frequently regulated chemicals, and those relevant to high school and undergraduate chemistry classes. This chemical information, curated by our expert scientists, is provided in alignment with our mission as a division of the American Chemical Society. | |
Explanation | The data from CAS Common Chemistry is provided under a CC-BY-NC 4.0 license, unless otherwise stated. | |
Record name | Lithium azide (Li(N3)) | |
Source | ChemIDplus | |
URL | https://pubchem.ncbi.nlm.nih.gov/substance/?source=chemidplus&sourceid=0019597694 | |
Description | ChemIDplus is a free, web search system that provides access to the structure and nomenclature authority files used for the identification of chemical substances cited in National Library of Medicine (NLM) databases, including the TOXNET system. | |
Record name | Lithium azide (Li(N3)) | |
Source | EPA Chemicals under the TSCA | |
URL | https://www.epa.gov/chemicals-under-tsca | |
Description | EPA Chemicals under the Toxic Substances Control Act (TSCA) collection contains information on chemicals and their regulations under TSCA, including non-confidential content from the TSCA Chemical Substance Inventory and Chemical Data Reporting. | |
Record name | Lithium azide (Li(N3)) | |
Source | EPA DSSTox | |
URL | https://comptox.epa.gov/dashboard/DTXSID8066509 | |
Description | DSSTox provides a high quality public chemistry resource for supporting improved predictive toxicology. | |
Record name | Lithium triazide | |
Source | European Chemicals Agency (ECHA) | |
URL | https://echa.europa.eu/substance-information/-/substanceinfo/100.039.237 | |
Description | The European Chemicals Agency (ECHA) is an agency of the European Union which is the driving force among regulatory authorities in implementing the EU's groundbreaking chemicals legislation for the benefit of human health and the environment as well as for innovation and competitiveness. | |
Explanation | Use of the information, documents and data from the ECHA website is subject to the terms and conditions of this Legal Notice, and subject to other binding limitations provided for under applicable law, the information, documents and data made available on the ECHA website may be reproduced, distributed and/or used, totally or in part, for non-commercial purposes provided that ECHA is acknowledged as the source: "Source: European Chemicals Agency, http://echa.europa.eu/". Such acknowledgement must be included in each copy of the material. ECHA permits and encourages organisations and individuals to create links to the ECHA website under the following cumulative conditions: Links can only be made to webpages that provide a link to the Legal Notice page. | |
Disclaimer and Information on In-Vitro Research Products
Please be aware that all articles and product information presented on BenchChem are intended solely for informational purposes. The products available for purchase on BenchChem are specifically designed for in-vitro studies, which are conducted outside of living organisms. In-vitro studies, derived from the Latin term "in glass," involve experiments performed in controlled laboratory settings using cells or tissues. It is important to note that these products are not categorized as medicines or drugs, and they have not received approval from the FDA for the prevention, treatment, or cure of any medical condition, ailment, or disease. We must emphasize that any form of bodily introduction of these products into humans or animals is strictly prohibited by law. It is essential to adhere to these guidelines to ensure compliance with legal and ethical standards in research and experimentation.