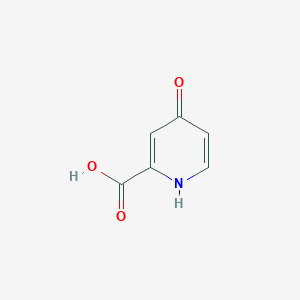
4-Hydroxypicolinic acid
Overview
Description
4-Hydroxypicolinic acid is a chemical compound with the formula C6H5NO3 . It is a derivative of pyridine and has a molecular weight of 139.11 g/mol . The IUPAC name for this compound is 4-oxo-1,4-dihydropyridine-2-carboxylic acid .
Synthesis Analysis
The synthesis of 4-Hydroxypicolinic acid involves the hydrolysis reaction of the nitrile group of the 4-alkoxy-3-hydroxypicolinonitriles . The starting picolinonitriles are typically suspended in a strong, aqueous mineral acid reaction medium and heated for a period of time at elevated temperature with good mixing .Molecular Structure Analysis
The molecular structure of 4-Hydroxypicolinic acid includes the arrangement of atoms and the chemical bonds that hold the atoms together . The molecule contains a total of 15 bonds. There are 10 non-H bonds, 4 multiple bonds, 1 rotatable bond, 4 double bonds, 1 six-membered ring, 1 carboxylic acid (aliphatic), 1 ketone (aliphatic), 1 secondary amine (aliphatic), and 1 hydroxyl group .Chemical Reactions Analysis
4-Hydroxypicolinic acid has been shown to be an anti-viral in vitro and in vivo . It works by binding to zinc finger proteins (ZFPs) in a way that changes their structures and disrupts zinc binding, inhibiting function .Physical And Chemical Properties Analysis
4-Hydroxypicolinic acid is a crystal - powder in physical form and its color ranges from white to very pale yellow . The compound is stable under normal conditions .Scientific Research Applications
4-Hydroxypicolinic acid is an organic compound with the formula C6H5NO3 . It’s a derivative of pyridine with a carboxylic acid substituent at the 2-position . The molecule contains a total of 15 bonds, including 10 non-H bonds, 4 multiple bonds, 1 rotatable bond, 4 double bonds, 1 six-membered ring, 1 carboxylic acid (aliphatic), 1 ketone (aliphatic), 1 secondary amine (aliphatic), and 1 hydroxyl group .
-
Matrix-Assisted Laser Desorption/Ionization Mass Spectrometry (MALDI-MS) : 4-Hydroxypicolinic acid has been used as a matrix substance in MALDI-MS applications for the analysis of oligonucleotides and proteins . The compound helps in the ionization process, facilitating the mass analysis of these biological molecules .
-
Chelating Agent : 4-Hydroxypicolinic acid is a bidentate chelating agent of elements such as chromium, zinc, manganese, copper, iron, and molybdenum in the human body . Many of its complexes are charge-neutral and thus lipophilic .
-
Synthetic Organic Chemistry : In synthetic organic chemistry, 4-Hydroxypicolinic acid has been used as a substrate in various reactions .
-
Thermophysical Property Datafile for Process Simulators : 4-Hydroxypicolinic acid can be used in process simulators like Aspen Plus. These simulators require accurate thermophysical property data for various chemical compounds, including 4-Hydroxypicolinic acid .
-
Free Radicals Thermodynamic Data for Oxidation, Combustion, and Thermal Cracking Kinetics : The thermodynamic properties of 4-Hydroxypicolinic acid can be used in the study of oxidation, combustion, and thermal cracking kinetics .
-
Quantum Tools for IR Spectra Interpretation : The infrared (IR) spectra of 4-Hydroxypicolinic acid can be analyzed using quantum tools. This can provide valuable insights into the molecular structure and vibrational modes of the compound .
-
Mitsunobu Reaction : In synthetic organic chemistry, 4-Hydroxypicolinic acid has been used as a substrate in the Mitsunobu reaction . The Mitsunobu reaction is an organic reaction that converts primary or secondary alcohols into esters, ethers, amines, and thioethers .
-
Hammick Reaction : 4-Hydroxypicolinic acid has also been used in the Hammick reaction . The Hammick reaction is a name reaction in the field of organic chemistry, specifically it is a rearrangement reaction .
-
Zinc Picolinate Dietary Supplements : After its role in absorption was discovered, zinc picolinate dietary supplements became popular as they were shown to be an effective means of introducing zinc into the body .
Safety And Hazards
Future Directions
properties
IUPAC Name |
4-oxo-1H-pyridine-2-carboxylic acid | |
---|---|---|
Source | PubChem | |
URL | https://pubchem.ncbi.nlm.nih.gov | |
Description | Data deposited in or computed by PubChem | |
InChI |
InChI=1S/C6H5NO3/c8-4-1-2-7-5(3-4)6(9)10/h1-3H,(H,7,8)(H,9,10) | |
Source | PubChem | |
URL | https://pubchem.ncbi.nlm.nih.gov | |
Description | Data deposited in or computed by PubChem | |
InChI Key |
MXXLHBCSVDDTIX-UHFFFAOYSA-N | |
Source | PubChem | |
URL | https://pubchem.ncbi.nlm.nih.gov | |
Description | Data deposited in or computed by PubChem | |
Canonical SMILES |
C1=CNC(=CC1=O)C(=O)O | |
Source | PubChem | |
URL | https://pubchem.ncbi.nlm.nih.gov | |
Description | Data deposited in or computed by PubChem | |
Molecular Formula |
C6H5NO3 | |
Source | PubChem | |
URL | https://pubchem.ncbi.nlm.nih.gov | |
Description | Data deposited in or computed by PubChem | |
DSSTOX Substance ID |
DTXSID70352740 | |
Record name | 4-Hydroxypicolinic acid | |
Source | EPA DSSTox | |
URL | https://comptox.epa.gov/dashboard/DTXSID70352740 | |
Description | DSSTox provides a high quality public chemistry resource for supporting improved predictive toxicology. | |
Molecular Weight |
139.11 g/mol | |
Source | PubChem | |
URL | https://pubchem.ncbi.nlm.nih.gov | |
Description | Data deposited in or computed by PubChem | |
Product Name |
4-Hydroxypicolinic acid | |
CAS RN |
22468-26-4 | |
Record name | 4-Hydroxypicolinic acid | |
Source | EPA DSSTox | |
URL | https://comptox.epa.gov/dashboard/DTXSID70352740 | |
Description | DSSTox provides a high quality public chemistry resource for supporting improved predictive toxicology. | |
Record name | 4-Hydroxy-2-pyridinecarboxylic Acid | |
Source | European Chemicals Agency (ECHA) | |
URL | https://echa.europa.eu/information-on-chemicals | |
Description | The European Chemicals Agency (ECHA) is an agency of the European Union which is the driving force among regulatory authorities in implementing the EU's groundbreaking chemicals legislation for the benefit of human health and the environment as well as for innovation and competitiveness. | |
Explanation | Use of the information, documents and data from the ECHA website is subject to the terms and conditions of this Legal Notice, and subject to other binding limitations provided for under applicable law, the information, documents and data made available on the ECHA website may be reproduced, distributed and/or used, totally or in part, for non-commercial purposes provided that ECHA is acknowledged as the source: "Source: European Chemicals Agency, http://echa.europa.eu/". Such acknowledgement must be included in each copy of the material. ECHA permits and encourages organisations and individuals to create links to the ECHA website under the following cumulative conditions: Links can only be made to webpages that provide a link to the Legal Notice page. | |
Retrosynthesis Analysis
AI-Powered Synthesis Planning: Our tool employs the Template_relevance Pistachio, Template_relevance Bkms_metabolic, Template_relevance Pistachio_ringbreaker, Template_relevance Reaxys, Template_relevance Reaxys_biocatalysis model, leveraging a vast database of chemical reactions to predict feasible synthetic routes.
One-Step Synthesis Focus: Specifically designed for one-step synthesis, it provides concise and direct routes for your target compounds, streamlining the synthesis process.
Accurate Predictions: Utilizing the extensive PISTACHIO, BKMS_METABOLIC, PISTACHIO_RINGBREAKER, REAXYS, REAXYS_BIOCATALYSIS database, our tool offers high-accuracy predictions, reflecting the latest in chemical research and data.
Strategy Settings
Precursor scoring | Relevance Heuristic |
---|---|
Min. plausibility | 0.01 |
Model | Template_relevance |
Template Set | Pistachio/Bkms_metabolic/Pistachio_ringbreaker/Reaxys/Reaxys_biocatalysis |
Top-N result to add to graph | 6 |
Feasible Synthetic Routes
Citations
Disclaimer and Information on In-Vitro Research Products
Please be aware that all articles and product information presented on BenchChem are intended solely for informational purposes. The products available for purchase on BenchChem are specifically designed for in-vitro studies, which are conducted outside of living organisms. In-vitro studies, derived from the Latin term "in glass," involve experiments performed in controlled laboratory settings using cells or tissues. It is important to note that these products are not categorized as medicines or drugs, and they have not received approval from the FDA for the prevention, treatment, or cure of any medical condition, ailment, or disease. We must emphasize that any form of bodily introduction of these products into humans or animals is strictly prohibited by law. It is essential to adhere to these guidelines to ensure compliance with legal and ethical standards in research and experimentation.