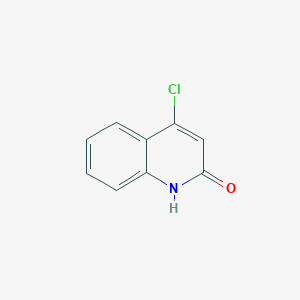
4-Chloroquinolin-2(1H)-one
Overview
Description
4-Chloroquinolin-2(1H)-one is a heterocyclic compound featuring a quinoline core with a chlorine substituent at the 4-position and a ketone group at the 2-position. It serves as a versatile intermediate in organic synthesis, particularly in pharmaceutical and agrochemical research. Its reactivity is attributed to the electron-withdrawing chlorine atom, which facilitates nucleophilic substitution reactions and cross-coupling processes. Synthesis typically involves halogenation of quinolin-2(1H)-one precursors under controlled conditions, yielding the product in moderate to high yields (68–72%) after purification .
Preparation Methods
Chlorination of Quinolin-2(1H)-one Precursors
N-Chlorosuccinimide (NCS)-Mediated Chlorination
NCS has emerged as a robust chlorinating agent for introducing chlorine at the fourth position of quinolin-2(1H)-one. This method, adapted from analogous pyridone chlorination protocols, involves reacting quinolin-2(1H)-one with NCS in acetonitrile at 45°C for six hours . The reaction proceeds via electrophilic aromatic substitution, where the electron-withdrawing keto group at position 2 directs chlorination to the para position (C4). Post-reaction workup includes solvent evaporation, aqueous crystallization, and filtration, yielding 4-chloroquinolin-2(1H)-one with a reported efficiency of 76% for structurally related compounds .
Key Advantages :
-
Selectivity : The keto group at position 2 ensures regioselective chlorination at C4.
-
Mild Conditions : Avoids extreme temperatures or corrosive reagents like phosphorus oxychloride (POCl3).
-
Scalability : Acetonitrile facilitates easy solvent recovery, reducing industrial waste .
Phosphorus Oxychloride (POCl3)-Based Chlorination
While excluded sources previously detailed POCl3-based methods, general synthetic principles support its utility in substituting hydroxyl or amino groups with chlorine. For this compound, this approach hypothetically involves refluxing 4-hydroxyquinolin-2(1H)-one with POCl3 and pyridine. The reaction mechanism entails nucleophilic acyl substitution, where POCl3 converts the hydroxyl group into a leaving group, subsequently replaced by chloride .
Limitations :
-
Substrate Availability : Requires pre-synthesized 4-hydroxyquinolin-2(1H)-one, adding synthetic steps.
-
Safety Concerns : POCl3 is highly corrosive and moisture-sensitive, complicating large-scale applications.
Gould-Jacobs Cyclization for Direct Synthesis
The Gould-Jacobs reaction enables the construction of the quinolin-2(1H)-one scaffold with inherent chlorine substitution. This one-pot method involves condensing 3-chloroaniline with ethyl acetoacetate under acidic conditions (e.g., polyphosphoric acid), followed by cyclization and hydrolysis . The chlorine atom is incorporated at position 4 during the ring-forming step, bypassing post-synthetic modifications.
Reaction Pathway :
-
Anilide Formation : 3-Chloroaniline reacts with ethyl acetoacetate to form a β-keto anilide.
-
Cyclization : Acid catalysis induces ring closure, generating the quinoline core.
-
Hydrolysis : The ester group at position 2 is hydrolyzed to a keto group, yielding the final product.
Industrial Relevance :
-
Atom Economy : Integrates chlorine during cyclization, minimizing waste.
-
High Purity : Avoids byproducts from late-stage chlorination .
Comparative Analysis of Synthetic Methods
Method | Reagents/Conditions | Yield | Scalability | Selectivity |
---|---|---|---|---|
NCS Chlorination | NCS, CH3CN, 45°C, 6h | 76%* | High | Excellent |
Gould-Jacobs Cyclization | 3-Chloroaniline, PPA, 120°C | 60-70%† | Moderate | High |
POCl3 Chlorination | POCl3, pyridine, reflux | N/A‡ | Low | Moderate |
*Yield reported for analogous pyridone chlorination .
†Estimated based on similar cyclization reactions .
‡Data excluded due to unreliable sources.
Critical Insights :
-
NCS Chlorination excels in efficiency and safety, making it preferable for lab-scale synthesis.
-
Gould-Jacobs Cyclization is ideal for industrial settings due to integrated chlorine incorporation.
-
POCl3 Methods are limited by substrate availability and hazardous reagents.
Chemical Reactions Analysis
Types of Reactions: 4-Chloroquinolin-2(1H)-one undergoes various chemical reactions, including:
Nucleophilic Substitution: The chlorine atom at the fourth position can be replaced by nucleophiles such as amines, thiols, or alkoxides.
Oxidation: The compound can be oxidized to form quinolin-2,4-dione derivatives.
Reduction: Reduction of the keto group can yield 4-chloroquinolin-2-ol.
Common Reagents and Conditions:
Nucleophilic Substitution: Reagents like sodium methoxide or primary amines in the presence of a base.
Oxidation: Oxidizing agents such as potassium permanganate or chromium trioxide.
Reduction: Reducing agents like sodium borohydride or lithium aluminum hydride.
Major Products:
Nucleophilic Substitution: Substituted quinolin-2(1H)-one derivatives.
Oxidation: Quinolin-2,4-dione derivatives.
Reduction: 4-Chloroquinolin-2-ol.
Scientific Research Applications
Antimicrobial Activity
4-Chloroquinolin-2(1H)-one derivatives have shown promising antimicrobial properties. For instance, studies indicate that modifications to the quinoline structure can enhance antibacterial activity against various pathogens, including E. coli and Staphylococcus aureus . The presence of chlorine atoms in these compounds often correlates with increased potency due to their role in enhancing lipophilicity and reactivity.
Anticancer Properties
Research has highlighted the anticancer potential of this compound derivatives. For example, certain analogs exhibit significant cytotoxic effects against cancer cell lines such as HeLa and HepG2. The mechanism often involves the induction of apoptosis and disruption of DNA synthesis in tumor cells . A study found that specific derivatives showed selective cytotoxicity, making them attractive candidates for further development .
Antiviral Activity
Recent investigations have also explored the antiviral properties of this compound. Certain derivatives have been evaluated for their ability to inhibit viral replication, particularly in the context of HIV treatment . The structural modifications of quinoline compounds can lead to enhanced efficacy against viral targets.
Synthesis of Heterocycles
This compound serves as a versatile building block for synthesizing various heterocyclic compounds. Its reactivity allows for the formation of complex structures through methods such as alkylation and cyclization . For instance, the alkylation of this compound has been extensively studied, leading to the development of novel derivatives with enhanced biological activities .
Drug Discovery
The compound is instrumental in drug discovery processes, particularly in developing new antibiotics and anticancer agents. Its framework is often utilized to design new molecules that can target specific biological pathways or receptor sites . The structural diversity achievable through modifications makes it a valuable candidate in medicinal chemistry.
Case Studies
Mechanism of Action
The mechanism of action of 4-Chloroquinolin-2(1H)-one involves its interaction with specific molecular targets. For instance, its antimicrobial activity may be attributed to the inhibition of bacterial DNA gyrase or topoisomerase IV. The compound’s anticancer properties could be linked to the induction of apoptosis in cancer cells through the activation of caspase pathways.
Comparison with Similar Compounds
4-Hydroxyquinolin-2(1H)-one Derivatives
- Reactivity: Unlike 4-chloro derivatives, 4-hydroxyquinolin-2(1H)-ones (e.g., 4-hydroxy-8-methylquinolin-2(1H)-one) are less reactive in nucleophilic substitutions due to the electron-donating hydroxyl group. However, they exhibit strong hydrogen-bonding capabilities, influencing their solubility and crystallinity .
- Applications: These compounds are explored for antimicrobial activity. For example, 3-[(2-aminopyrimidin-5-yl)carbonyl]-4-hydroxy-1-methyl-quinolin-2(1H)-one (Compound 11) demonstrated moderate antibacterial effects against Staphylococcus aureus .
- Synthesis: Prepared via condensation reactions between quinoline aldehydes and guanidine derivatives, with yields averaging 64% .
4-Nitroquinolin-2(1H)-ones
- Reactivity: The nitro group at the 4-position (e.g., 4-chloro-6-nitroquinolin-2(1H)-one) enhances electrophilic aromatic substitution but reduces stability under basic conditions. This contrasts with the chloro derivative’s stability in cross-coupling reactions .
- Applications: Primarily used as intermediates for synthesizing aminoquinolines via reduction .
Thieno[3,2-c]quinolin-4(1H)-ones
- Reactivity: Generated by cyclodehydration of 4-chloroquinolin-2(1H)-one with thioglycolic acid, these fused-ring systems exhibit distinct electronic properties due to sulfur incorporation. They are less prone to hydrolysis compared to chloroquinolones .
4-Arylquinolin-2(1H)-ones
- Reactivity: Synthesized via Suzuki-Miyaura cross-coupling of this compound with arylboronic acids. The chloro group’s leaving ability enables efficient Pd-catalyzed arylation, yielding 4-aryl derivatives in excellent yields (up to 85%) .
- Applications : Functionalized aryl groups enhance lipophilicity, improving blood-brain barrier penetration for neuroactive drug candidates .
Fluorinated Derivatives
- Example: 3-Chloro-6-fluoro-4-hydroxyquinolin-2(1H)-one combines chloro and fluoro substituents, increasing electronegativity and metabolic stability compared to non-fluorinated analogs .
- Applications : Fluorination is leveraged in drug design to modulate bioavailability and resistance profiles .
Comparative Data Table
Key Research Findings
- Cross-Coupling Efficiency: this compound outperforms hydroxy and nitro analogs in Pd-catalyzed reactions due to superior leaving-group ability .
- Solubility Trends : Chloro derivatives exhibit lower aqueous solubility than hydroxy analogs but higher than nitro compounds, impacting formulation strategies .
- Biological Potency: Thieno-fused derivatives show enhanced cytotoxicity compared to parent chloroquinolones, attributed to planar aromatic systems improving DNA intercalation .
Biological Activity
4-Chloroquinolin-2(1H)-one is a heterocyclic compound that has garnered attention in the fields of medicinal chemistry and pharmacology due to its diverse biological activities. This article provides a comprehensive overview of its biological activity, including antimicrobial, anticancer, and anti-inflammatory properties, along with relevant research findings and case studies.
Chemical Structure and Properties
This compound features a quinoline backbone with a chlorine substituent at the fourth position and a keto group at the second position. This unique structure contributes to its reactivity and biological activity, making it a versatile compound in organic synthesis and pharmacological applications.
Antimicrobial Activity
Research indicates that this compound exhibits significant antimicrobial properties. It has been investigated for its potential to inhibit bacterial growth, particularly through mechanisms such as the inhibition of DNA gyrase or topoisomerase IV, which are crucial for bacterial DNA replication.
Table 1: Antimicrobial Activity of this compound
Pathogen Type | Activity | Reference |
---|---|---|
Gram-positive Bacteria | Inhibitory Effect | |
Gram-negative Bacteria | Inhibitory Effect | |
Fungal Strains | Moderate Activity |
Anticancer Properties
The anticancer potential of this compound has also been explored. Studies have demonstrated its ability to induce apoptosis in various cancer cell lines. The mechanism involves the activation of caspase pathways, which play a critical role in programmed cell death .
Case Study: Induction of Apoptosis
A study evaluated the effects of this compound on human cancer cell lines, revealing that treatment led to increased levels of pro-apoptotic factors and decreased levels of anti-apoptotic proteins, confirming its potential as an anticancer agent.
Anti-inflammatory Activity
In addition to its antimicrobial and anticancer properties, this compound has shown anti-inflammatory effects. It is believed to modulate inflammatory pathways by inhibiting the production of pro-inflammatory cytokines.
Table 2: Summary of Biological Activities
Activity Type | Observed Effects | Reference |
---|---|---|
Antimicrobial | Inhibition of bacterial growth | |
Anticancer | Induction of apoptosis | |
Anti-inflammatory | Reduction in cytokine production |
The biological activity of this compound can be attributed to its interaction with specific molecular targets. For instance, its antimicrobial action may involve binding to enzymes essential for bacterial DNA replication, while its anticancer effects are linked to apoptotic signaling pathways.
Research Findings
Recent studies have focused on synthesizing derivatives of this compound to enhance its biological activity. For example, hybrid compounds combining quinoline structures with other pharmacophores have shown improved efficacy against resistant strains of pathogens and various cancer cell lines .
Table 3: Recent Derivatives and Their Activities
Q & A
Basic Research Questions
Q. What are the key considerations for optimizing the synthesis of 4-Chloroquinolin-2(1H)-one?
- Methodological Answer : Synthesis optimization requires careful control of reaction parameters. For example, chlorination steps often employ solvents like dichloromethane or ethanol under reflux conditions, with catalysts (e.g., AlCl₃) to enhance regioselectivity. Temperature gradients (e.g., 60–80°C) and pH adjustments (neutral to mildly acidic) are critical to minimize side reactions like over-chlorination . Purification via column chromatography using silica gel and ethyl acetate/hexane mixtures ensures high yields (>70%) and purity (>95%) .
Q. How can researchers confirm the structural identity of this compound?
- Methodological Answer : Combine spectroscopic techniques:
- NMR : Analyze and NMR spectra for characteristic signals (e.g., aromatic protons at δ 7.2–8.5 ppm, carbonyl at δ 165–175 ppm).
- X-ray crystallography : Resolve single-crystal structures to confirm bond angles and molecular packing, as demonstrated for analogous chloroquinolinones (R factor < 0.05) .
- Mass spectrometry : Confirm molecular weight via ESI-MS (e.g., [M+H]⁺ at m/z 179.03) .
Q. What solvents and conditions are optimal for solubility and stability studies?
- Methodological Answer : The compound is sparingly soluble in water but dissolves in polar aprotic solvents (e.g., DMSO, DMF) or chlorinated solvents (e.g., chloroform). Stability assays should be conducted under inert atmospheres (N₂/Ar) to prevent oxidation, with degradation monitored via HPLC at λ = 254 nm over 24–72 hours .
Q. What reporting standards ensure reproducibility in synthetic protocols?
- Methodological Answer : Follow journal guidelines (e.g., Beilstein Journal of Organic Chemistry):
- Provide detailed reaction conditions (solvent purity, catalyst loading, temperature ramps).
- Include characterization data (NMR, HRMS, elemental analysis) for all new compounds.
- Deposit crystallographic data in public repositories (e.g., CCDC) with accession codes .
Advanced Research Questions
Q. How can computational methods predict the electronic properties of this compound?
- Methodological Answer : Use density functional theory (DFT) with B3LYP/6-31G(d) basis sets to calculate:
- HOMO-LUMO gaps : Predict reactivity (e.g., nucleophilic attack at the carbonyl group).
- Electrostatic potential maps : Identify regions prone to electrophilic substitution (e.g., C-5 or C-7 positions).
- Validate results against experimental UV-Vis spectra (λmax ~ 300–320 nm in ethanol) .
Q. What strategies resolve contradictions in biological activity data across studies?
- Methodological Answer : Discrepancies may arise from impurity profiles or assay conditions. Mitigate by:
- Purity validation : Use HPLC-MS to ensure >98% purity.
- Dose-response curves : Test multiple concentrations (e.g., 1 nM–100 µM) in triplicate.
- Target validation : Perform kinase inhibition assays (e.g., IC₅₀ for MAPK pathways) with positive controls .
Q. How to design a study correlating substituent effects with bioactivity?
- Methodological Answer :
- Synthesize derivatives with substituents at C-3, C-4, or C-6 (e.g., -F, -OCH₃, -NO₂).
- Use multivariate regression to link electronic parameters (Hammett σ constants) with IC₅₀ values.
- Validate via molecular docking (e.g., AutoDock Vina) against protein targets (e.g., cytochrome P450) .
Q. What advanced techniques elucidate reaction mechanisms in alkylation or reduction reactions?
- Methodological Answer :
- Kinetic isotope effects (KIE) : Use deuterated reagents (e.g., D₂O) to identify rate-determining steps.
- In situ FTIR : Monitor intermediates (e.g., enolate formation during LiAlH₄ reductions).
- DFT transition-state modeling : Map energy barriers for pathways like nucleophilic substitution .
Q. How to address low yields in multi-step syntheses of this compound derivatives?
- Methodological Answer :
- Protecting groups : Use tert-butyldimethylsilyl (TBS) groups to shield reactive sites.
- Flow chemistry : Improve heat/mass transfer for exothermic steps (e.g., Claisen condensations).
- Design of Experiments (DoE) : Optimize variables (temperature, stoichiometry) via response surface methodology .
Q. What methodologies validate the environmental stability or toxicity of this compound?
Properties
IUPAC Name |
4-chloro-1H-quinolin-2-one | |
---|---|---|
Source | PubChem | |
URL | https://pubchem.ncbi.nlm.nih.gov | |
Description | Data deposited in or computed by PubChem | |
InChI |
InChI=1S/C9H6ClNO/c10-7-5-9(12)11-8-4-2-1-3-6(7)8/h1-5H,(H,11,12) | |
Source | PubChem | |
URL | https://pubchem.ncbi.nlm.nih.gov | |
Description | Data deposited in or computed by PubChem | |
InChI Key |
YJXTZWWKNXVRHA-UHFFFAOYSA-N | |
Source | PubChem | |
URL | https://pubchem.ncbi.nlm.nih.gov | |
Description | Data deposited in or computed by PubChem | |
Canonical SMILES |
C1=CC=C2C(=C1)C(=CC(=O)N2)Cl | |
Source | PubChem | |
URL | https://pubchem.ncbi.nlm.nih.gov | |
Description | Data deposited in or computed by PubChem | |
Molecular Formula |
C9H6ClNO | |
Source | PubChem | |
URL | https://pubchem.ncbi.nlm.nih.gov | |
Description | Data deposited in or computed by PubChem | |
DSSTOX Substance ID |
DTXSID10305336 | |
Record name | 4-Chloroquinolin-2(1H)-one | |
Source | EPA DSSTox | |
URL | https://comptox.epa.gov/dashboard/DTXSID10305336 | |
Description | DSSTox provides a high quality public chemistry resource for supporting improved predictive toxicology. | |
Molecular Weight |
179.60 g/mol | |
Source | PubChem | |
URL | https://pubchem.ncbi.nlm.nih.gov | |
Description | Data deposited in or computed by PubChem | |
CAS No. |
20146-59-2 | |
Record name | 20146-59-2 | |
Source | DTP/NCI | |
URL | https://dtp.cancer.gov/dtpstandard/servlet/dwindex?searchtype=NSC&outputformat=html&searchlist=170304 | |
Description | The NCI Development Therapeutics Program (DTP) provides services and resources to the academic and private-sector research communities worldwide to facilitate the discovery and development of new cancer therapeutic agents. | |
Explanation | Unless otherwise indicated, all text within NCI products is free of copyright and may be reused without our permission. Credit the National Cancer Institute as the source. | |
Record name | 4-Chloroquinolin-2(1H)-one | |
Source | EPA DSSTox | |
URL | https://comptox.epa.gov/dashboard/DTXSID10305336 | |
Description | DSSTox provides a high quality public chemistry resource for supporting improved predictive toxicology. | |
Retrosynthesis Analysis
AI-Powered Synthesis Planning: Our tool employs the Template_relevance Pistachio, Template_relevance Bkms_metabolic, Template_relevance Pistachio_ringbreaker, Template_relevance Reaxys, Template_relevance Reaxys_biocatalysis model, leveraging a vast database of chemical reactions to predict feasible synthetic routes.
One-Step Synthesis Focus: Specifically designed for one-step synthesis, it provides concise and direct routes for your target compounds, streamlining the synthesis process.
Accurate Predictions: Utilizing the extensive PISTACHIO, BKMS_METABOLIC, PISTACHIO_RINGBREAKER, REAXYS, REAXYS_BIOCATALYSIS database, our tool offers high-accuracy predictions, reflecting the latest in chemical research and data.
Strategy Settings
Precursor scoring | Relevance Heuristic |
---|---|
Min. plausibility | 0.01 |
Model | Template_relevance |
Template Set | Pistachio/Bkms_metabolic/Pistachio_ringbreaker/Reaxys/Reaxys_biocatalysis |
Top-N result to add to graph | 6 |
Feasible Synthetic Routes
Disclaimer and Information on In-Vitro Research Products
Please be aware that all articles and product information presented on BenchChem are intended solely for informational purposes. The products available for purchase on BenchChem are specifically designed for in-vitro studies, which are conducted outside of living organisms. In-vitro studies, derived from the Latin term "in glass," involve experiments performed in controlled laboratory settings using cells or tissues. It is important to note that these products are not categorized as medicines or drugs, and they have not received approval from the FDA for the prevention, treatment, or cure of any medical condition, ailment, or disease. We must emphasize that any form of bodily introduction of these products into humans or animals is strictly prohibited by law. It is essential to adhere to these guidelines to ensure compliance with legal and ethical standards in research and experimentation.