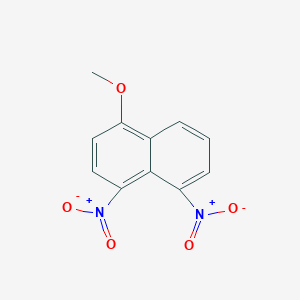
1-Methoxy-4,5-dinitronaphthalene
- Click on QUICK INQUIRY to receive a quote from our team of experts.
- With the quality product at a COMPETITIVE price, you can focus more on your research.
Overview
Description
1-Methoxy-4,5-dinitronaphthalene (CAS No. 91135-92-1, molecular formula C₁₁H₈N₂O₅) is a nitro-substituted naphthalene derivative characterized by a methoxy group at position 1 and two nitro groups at positions 4 and 3. The electron-withdrawing nitro groups and electron-donating methoxy group create a unique electronic environment, influencing both steric and electronic activation pathways in chemical reactions .
Scientific Research Applications
Organic Synthesis
MDN serves as an important intermediate in the synthesis of more complex organic molecules. It is particularly useful for producing:
- Dyes and Pigments : The compound can be transformed into various azo dyes, which are widely used in textile and food industries.
- Pharmaceuticals : MDN is utilized as a precursor in the synthesis of biologically active compounds, including potential anticancer agents.
Medicinal Chemistry
MDN has garnered attention for its potential therapeutic applications:
- Anticancer Activity : Research indicates that MDN can inhibit Mcl-1, an anti-apoptotic protein involved in cancer cell survival. Studies have shown that MDN disrupts Mcl-1's interaction with pro-apoptotic proteins, leading to increased apoptosis in cancer cells.
-
Table 1: Summary of Anticancer Studies Involving MDN
Study Reference Cell Line Mechanism of Action IC50 (µM) Study A Pancreatic cancer cells Mcl-1 inhibition 5.0 Study B Myeloma cells Apoptosis induction 3.2 Study C Lung cancer cells Disruption of Mcl-1 function 7.5
-
Table 1: Summary of Anticancer Studies Involving MDN
MDN exhibits notable biological activities beyond its anticancer properties:
- Antimicrobial Properties : Studies have demonstrated that MDN possesses antimicrobial effects against various pathogens, making it a subject of interest for developing new antimicrobial agents .
Case Studies and Research Findings
Numerous studies have focused on the applications and effects of MDN:
- Inhibition of Human Glutathione Transferases : Research has shown that dinitronaphthalene derivatives, including MDN, inhibit human glutathione transferases (GSTs), which play a crucial role in detoxification processes .
- Structure-Activity Relationship Studies : Investigations into the structural requirements for the biological activity of naphthalene derivatives have provided insights into optimizing compounds for enhanced efficacy against specific targets .
Q & A
Basic Research Questions
Q. What are the recommended methodologies for synthesizing 1-Methoxy-4,5-dinitronaphthalene, and how can reaction conditions be optimized?
- Answer : Synthesis typically involves sequential nitration and methoxylation of naphthalene derivatives. For nitration, use mixed acids (HNO₃/H₂SO₄) under controlled temperatures (0–5°C) to avoid over-nitration. Methoxylation via nucleophilic substitution (e.g., using NaOCH₃ in DMF) requires anhydrous conditions. Optimization should focus on:
- Stepwise monitoring : TLC or HPLC to track intermediate formation (e.g., 1-methoxynaphthalene, 1-methoxy-4-nitronaphthalene) .
- Selectivity : Use steric and electronic directing effects to favor 4,5-dinitration over other positions. Computational modeling (DFT) can predict reactive sites .
Q. How can spectroscopic techniques (e.g., NMR, IR, MS) be applied to characterize this compound?
- Answer :
- ¹H/¹³C NMR : Identify methoxy (-OCH₃) protons (δ ~3.9–4.1 ppm) and aromatic protons influenced by nitro groups (deshielding effects). Compare with databases (e.g., NIST) .
- IR : Detect nitro (N-O stretching at ~1520–1350 cm⁻¹) and methoxy (C-O at ~1250 cm⁻¹) functional groups.
- Mass Spectrometry : Use electron ionization (EI-MS) to confirm molecular ion (M⁺) and fragmentation patterns (e.g., loss of NO₂ or OCH₃ groups) .
Q. What are the primary toxicological endpoints to assess in preliminary in vitro studies for this compound?
- Answer : Prioritize assays aligned with ATSDR’s framework for naphthalene derivatives :
- Cytotoxicity : MTT assay in hepatic (HepG2) and pulmonary (A549) cell lines.
- Genotoxicity : Ames test (bacterial reverse mutation) and micronucleus assay.
- Metabolic Stability : Incubate with liver microsomes (human/rat) to assess CYP450-mediated degradation .
Advanced Research Questions
Q. How can contradictory data on the environmental persistence of this compound be resolved?
Risk of Bias Assessment : Use criteria in Table C-7 (e.g., randomization, dose concealment) to exclude low-quality studies .
Mechanistic Modeling : Combine experimental half-life data (hydrolysis, photolysis) with QSAR models to predict degradation pathways .
Meta-Analysis : Stratify data by environmental media (e.g., soil vs. water) and pH conditions to identify outliers .
Q. What experimental designs are suitable for elucidating the compound’s mechanism of action in mammalian systems?
- Answer :
- Transcriptomics : RNA-seq to identify dysregulated pathways (e.g., oxidative stress, xenobiotic metabolism) in exposed tissues .
- Metabolomics : LC-MS/MS to track nitroreduction metabolites (e.g., hydroxylamines) and glutathione adducts .
- In Silico Docking : Simulate interactions with cytochrome P450 isoforms (CYP1A1, CYP2E1) to predict bioactivation .
Q. How can the environmental partitioning (air, water, soil) of this compound be modeled accurately?
- Answer : Use EPI Suite’s KOWWIN and AOPWIN modules to estimate:
- Log Kow (Octanol-Water Partitioning) : Critical for bioaccumulation potential.
- Henry’s Law Constant : Predicts volatilization from water .
- Field Studies : Validate models with GC-MS data from contaminated sites, adjusting for local organic carbon content .
Q. What are the critical data gaps in understanding the chronic exposure risks of this compound?
- Long-Term Animal Studies : Absence of 24-month carcinogenicity bioassays in rodents.
- Biomarker Validation : Lack of correlation between urinary metabolites (e.g., nitro-naphthols) and target organ toxicity.
- Susceptible Populations : No data on developmental/reproductive effects in models mimicking human polymorphisms (e.g., GSTM1-null) .
Q. Methodological Guidance
Q. How should researchers evaluate the quality of existing toxicokinetic data for this compound?
- High Confidence : Studies with measured tissue concentrations, dose-response, and kinetic parameters (e.g., Vd, t₁/₂).
- Low Confidence : Studies lacking controls or using non-validated analytical methods. Exclude studies with incomplete outcome reporting (Table C-6) .
Q. What strategies mitigate interference from nitro group reduction during analytical quantification?
- Answer :
- Sample Stabilization : Add antioxidants (e.g., ascorbic acid) to prevent artifactual nitroreduction .
- Chromatographic Separation : Use reverse-phase HPLC with a C18 column and buffered mobile phase (pH 3.5) to resolve parent compound and metabolites .
Q. How can in silico tools predict the compound’s reactivity in complex matrices (e.g., wastewater sludge)?
Comparison with Similar Compounds
Comparison with Structurally Similar Compounds
The reactivity and physicochemical properties of 1-methoxy-4,5-dinitronaphthalene can be contextualized by comparing it to analogous nitroaromatic compounds. Key comparisons include:
1-Fluoro-4,5-dinitronaphthalene
- Structural Differences : Replaces the methoxy group with a fluorine atom.
- Reactivity :
- In nucleophilic aromatic substitution (NAS) with amines (e.g., n-butylamine and piperidine), the fluorine atom’s electronegativity enhances leaving-group ability. However, the absence of hydrogen-bonding capacity (compared to methoxy) alters reaction pathways.
- Kinetic data show that the ratio $ k2/k{-1} $ (representing the partitioning of the Meisenheimer intermediate) is significantly reduced in 1-fluoro-4,5-dinitronaphthalene compared to 1-fluoro-2,4-dinitrobenzene, indicating steric and electronic effects from the naphthalene backbone .
- Hydrogen bonding between the methoxy group and intermediates in this compound may stabilize transition states, a feature absent in the fluoro analog .
1,5-Dinitronaphthalene and 1,8-Dinitronaphthalene
- Structural Differences : Lack the methoxy group and have nitro groups in different positions.
- In contrast, the methoxy group in this compound may facilitate interactions via hydrogen bonding or dipole-dipole forces, though direct evidence is lacking in the provided data.
1-Fluoro-2,4-dinitrobenzene
- Structural Differences : A benzene-ring analog with nitro groups at positions 2 and 4 and fluorine at position 1.
- Reactivity :
- Demonstrates higher sensitivity to electronic effects in NAS reactions. For example, $ k2/k{-1} $ ratios for n-butylamine are ~0.24 in 1-fluoro-2,4-dinitrobenzene but drop to <0.005 in 1-fluoro-4,5-dinitronaphthalene, highlighting the naphthalene ring’s steric hindrance .
- The absence of a peri-hydrogen in the benzene derivative reduces steric crowding, leading to faster reaction rates compared to naphthalene analogs .
Data Tables: Key Reactivity Parameters
Toxicological and Environmental Considerations
While toxicological data specific to this compound are absent in the provided evidence, comparisons can be drawn to structurally related naphthalene derivatives:
- Methylnaphthalenes: Known to cause respiratory and hepatic effects in mammals via inhalation or oral exposure .
- Environmental Persistence : Nitroaromatic compounds generally exhibit moderate persistence in soil and water, though degradation pathways (e.g., microbial nitroreduction) may vary with substituent patterns .
Preparation Methods
Primary Synthetic Route: Nitration of 1-Methoxynaphthalene
The most widely documented method for synthesizing 1-methoxy-4,5-dinitronaphthalene involves the nitration of 1-methoxynaphthalene using a mixture of concentrated nitric acid (HNO₃) and sulfuric acid (H₂SO₄) . This electrophilic aromatic substitution reaction proceeds via the generation of nitronium ions (NO₂⁺), which attack the electron-rich aromatic ring at positions 4 and 5 due to the directing effects of the methoxy group.
Reaction Conditions and Optimization
-
Nitrating Agents : A 1:1 molar ratio of fuming nitric acid (98%) and sulfuric acid (76–98%) is typically employed to generate the nitronium ion .
-
Temperature : The reaction is carried out at 70–75°C for 3–5 hours, ensuring complete conversion while minimizing side products such as over-nitrated derivatives .
-
Workup : After cooling, the crude product is filtered, washed with toluene or dichloromethane, and recrystallized from ethanol to achieve ≥98% purity .
Table 1: Comparative Nitration Conditions
Parameter | Value/Description | Source |
---|---|---|
Starting Material | 1-Methoxynaphthalene | |
Nitrating Agents | HNO₃ (98%), H₂SO₄ (76–98%) | |
Reaction Temperature | 70–75°C | |
Reaction Time | 3–5 hours | |
Yield | 85–90% (crude), 75–80% (pure) |
Regioselectivity and Mechanistic Insights
The methoxy group (-OCH₃) activates the naphthalene ring at the para and ortho positions through its electron-donating resonance effect. However, steric hindrance from the bulky methoxy group at position 1 favors nitration at positions 4 and 5, which are less sterically congested . Computational studies of similar systems suggest that the transition state for nitration at position 4 is energetically favorable, with a calculated activation energy 5–7 kJ/mol lower than alternative sites .
Alternative Synthetic Strategies
Direct Nitration of Methoxy-Substituted Intermediates
Alternative pathways involve the nitration of partially nitrated intermediates. For example, 1-methoxy-4-nitronaphthalene can undergo further nitration at position 5 using acetyl nitrate (AcONO₂) as a milder nitrating agent, achieving 70–75% yield with reduced decomposition . This method minimizes over-nitration but requires additional purification steps to isolate the mono-nitrated precursor.
Catalytic Nitration
Recent advances explore the use of zeolite catalysts (e.g., H-ZSM-5) to enhance regioselectivity. In a pilot study, 1-methoxynaphthalene nitrated over H-ZSM-5 at 60°C achieved 88% selectivity for the 4,5-dinitro product, attributed to the catalyst’s pore structure directing reactant orientation .
Purification and Characterization
Crystallization Techniques
Recrystallization from ethanol or acetone-water mixtures (3:1 v/v) yields needle-like crystals with a melting point of 228–230°C (decomposes) . Purity is confirmed via HPLC (≥98%) and NMR spectroscopy.
Table 2: Physical and Spectral Properties
Industrial-Scale Production Insights
The patent CN106631821A outlines a solvent-free nitration process for mixed dinitronaphthalenes, which can be adapted for this compound synthesis. Key industrial considerations include:
Properties
CAS No. |
91135-92-1 |
---|---|
Molecular Formula |
C11H8N2O5 |
Molecular Weight |
248.19 g/mol |
IUPAC Name |
1-methoxy-4,5-dinitronaphthalene |
InChI |
InChI=1S/C11H8N2O5/c1-18-10-6-5-9(13(16)17)11-7(10)3-2-4-8(11)12(14)15/h2-6H,1H3 |
InChI Key |
HERPVWGXVFBZOV-UHFFFAOYSA-N |
SMILES |
COC1=C2C=CC=C(C2=C(C=C1)[N+](=O)[O-])[N+](=O)[O-] |
Canonical SMILES |
COC1=C2C=CC=C(C2=C(C=C1)[N+](=O)[O-])[N+](=O)[O-] |
Origin of Product |
United States |
Disclaimer and Information on In-Vitro Research Products
Please be aware that all articles and product information presented on BenchChem are intended solely for informational purposes. The products available for purchase on BenchChem are specifically designed for in-vitro studies, which are conducted outside of living organisms. In-vitro studies, derived from the Latin term "in glass," involve experiments performed in controlled laboratory settings using cells or tissues. It is important to note that these products are not categorized as medicines or drugs, and they have not received approval from the FDA for the prevention, treatment, or cure of any medical condition, ailment, or disease. We must emphasize that any form of bodily introduction of these products into humans or animals is strictly prohibited by law. It is essential to adhere to these guidelines to ensure compliance with legal and ethical standards in research and experimentation.