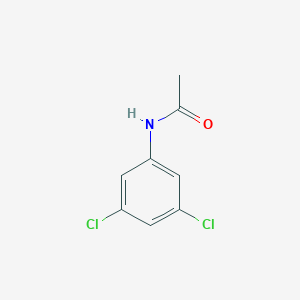
N-(3,5-dichlorophenyl)acetamide
Overview
Description
N-(3,5-Dichlorophenyl)acetamide (chemical formula: C₈H₆Cl₂NO) is a halogenated aromatic acetamide derivative characterized by two chlorine atoms at the meta positions (3 and 5) of the phenyl ring and an acetamide group at the para position. This compound is a key subject in crystallographic and synthetic studies due to its structural simplicity and relevance in understanding substituent effects on molecular packing and hydrogen-bonding interactions .
Preparation Methods
Direct Acetylation of 3,5-Dichloroaniline
The most widely documented method involves the acetylation of 3,5-dichloroaniline using acetic anhydride or acetyl chloride. This reaction follows a nucleophilic acyl substitution mechanism, where the amine group attacks the electrophilic carbonyl carbon of the acetylating agent .
Reaction Conditions and Optimization
-
Reagents : 3,5-Dichloroaniline is reacted with acetic anhydride in a 1:1.2 molar ratio under inert conditions.
-
Solvent System : Anhydrous toluene or dichloromethane is preferred to minimize hydrolysis of the acetylating agent.
-
Catalysis : Pyridine or triethylamine (2–5 mol%) is added to scavenge HCl, preventing protonation of the aniline and ensuring efficient acetylation .
-
Temperature : Reactions proceed at 60–80°C for 4–6 hours, achieving yields exceeding 85% after recrystallization from ethanol .
Mechanistic Insights :
The reaction initiates with the lone pair on the aniline’s nitrogen attacking the electrophilic carbonyl carbon of acetic anhydride, forming a tetrahedral intermediate. Subsequent elimination of acetate yields the acetamide product. Steric hindrance from the 3,5-dichloro substituents slightly slows the reaction compared to unsubstituted anilines, necessitating prolonged heating .
Synthesis via 3,5-Dichloroaniline Intermediate
When 3,5-dichloroaniline is unavailable, it can be synthesized from 2,4-dichloroaniline through bromination and amination, followed by acetylation.
Bromination of 2,4-Dichloroaniline
-
Step 1 : 2,4-Dichloroaniline is dissolved in hydrochloric acid and brominated at 0–5°C to form 2-bromo-4,6-dichloroaniline hydrochloride .
-
Isolation : The precipitate is filtered and washed with cold ethanol to remove excess bromine .
Thermal Rearrangement to 3,5-Dichlorobromobenzene
-
Step 2 : The brominated intermediate is treated with sodium nitrite in ethanol at 0°C, followed by heating to reflux (78°C) to eliminate nitrogen and form 3,5-dichlorobromobenzene :
Amination to 3,5-Dichloroaniline
-
Step 3 : 3,5-Dichlorobromobenzene undergoes catalytic amination with aqueous ammonia at 130–180°C under 5–10 bar pressure, using CuBr as a catalyst :
-
Yield : This three-step sequence achieves a 65–70% overall yield of 3,5-dichloroaniline .
Final Acetylation
The synthesized 3,5-dichloroaniline is acetylated as described in Section 1, completing the pathway.
Comparative Analysis of Preparation Methods
Chemical Reactions Analysis
Types of Reactions:
Substitution Reactions: N-(3,5-dichlorophenyl)acetamide can undergo nucleophilic substitution reactions, particularly at the chlorine atoms. Common reagents for these reactions include sodium methoxide or potassium tert-butoxide.
Hydrolysis: The compound can be hydrolyzed under acidic or basic conditions to yield 3,5-dichloroaniline and acetic acid.
Oxidation: Oxidative reactions can convert the amide group to a nitro group, although this is less common.
Common Reagents and Conditions:
Nucleophilic Substitution: Sodium methoxide in methanol, reflux conditions.
Hydrolysis: Aqueous sodium hydroxide or hydrochloric acid, reflux conditions.
Oxidation: Potassium permanganate in acidic medium.
Major Products Formed:
Substitution: Products depend on the nucleophile used, such as methoxy derivatives.
Hydrolysis: 3,5-dichloroaniline and acetic acid.
Oxidation: Nitro derivatives, though less common.
Scientific Research Applications
Chemistry
- Precursor in Organic Synthesis : N-(3,5-dichlorophenyl)acetamide serves as a precursor for synthesizing more complex organic molecules. Its ability to undergo various chemical reactions (oxidation, reduction, substitution) makes it valuable in developing new compounds with desired properties.
Biology
- Biological Interactions : Research indicates that this compound interacts with biological macromolecules, potentially affecting enzyme activity or receptor signaling pathways. This interaction can lead to various biological effects, making it a candidate for further studies in biochemical applications.
Medicine
- Antiviral Properties : Recent studies have highlighted the antiviral efficacy of derivatives of this compound against SARS-CoV-2. For instance, compounds derived from it have shown significant reductions in viral load in preclinical models, indicating potential as therapeutic agents against COVID-19 .
- Anti-inflammatory and Antimicrobial Activities : Investigations into the therapeutic properties of this compound have revealed its potential anti-inflammatory and antimicrobial effects. These properties are crucial for developing treatments for infections and inflammatory diseases.
Industry
- Material Development : In industrial applications, this compound is utilized in developing new materials and chemical processes. Its unique chemical structure allows it to be incorporated into various formulations that require specific thermal or chemical stability.
Data Table: Summary of Applications
Application Area | Specific Use | Findings |
---|---|---|
Chemistry | Precursor for organic synthesis | Facilitates formation of complex molecules |
Biology | Interaction with macromolecules | Alters enzyme activity and receptor signaling |
Medicine | Antiviral agent | Significant reduction in viral load against SARS-CoV-2 |
Anti-inflammatory properties | Potential for treating inflammatory diseases | |
Industry | Material development | Used in formulations requiring specific stability |
Case Studies
- Antiviral Activity Against SARS-CoV-2 : A study demonstrated that derivatives of this compound significantly reduced viral RNA loads in infected models. These findings suggest that such compounds could serve as effective antiviral agents .
- Toxicity Studies : Toxicity assessments conducted on animal models indicated that this compound derivatives exhibited low toxicity levels at therapeutic doses, supporting their potential use in medical applications without significant adverse effects .
- Biological Mechanisms : Investigations into the biological mechanisms revealed that these compounds could inhibit specific enzymes involved in viral replication, highlighting their role as potential therapeutic agents against viral infections .
Mechanism of Action
The mechanism of action of N-(3,5-dichlorophenyl)acetamide largely depends on its application. In antimicrobial studies, it is believed to disrupt cell membrane integrity or interfere with essential enzymatic processes in microorganisms. The exact molecular targets and pathways are still under investigation, but its chlorine atoms and acetamide group are thought to play crucial roles in its bioactivity.
Comparison with Similar Compounds
Key Structural Features:
- Crystal System: Monoclinic (as per related analogs like 2-chloro-N-(3,5-dichlorophenyl)acetamide) .
- Hydrogen Bonding : Stabilized by intermolecular N–H⋯O and N–H⋯Cl interactions, forming chains along the crystallographic axis .
- Synthetic Route : Typically synthesized via condensation of 3,5-dichloroaniline with acetylating agents (e.g., trichloroacetic acid and phosphoryl chloride) .
Comparison with Structurally Similar Compounds
Substituent Effects on Crystallography and Geometry
The meta-dichloro substitution pattern in N-(3,5-dichlorophenyl)acetamide distinguishes it from other N-aryl acetamides. Below is a comparative analysis of its structural and electronic properties against analogs:
Table 1: Crystallographic and Substituent-Based Comparison
Key Observations :
- Electron-Withdrawing vs. Electron-Donating Groups : The 3,5-dichloro substitution (electron-withdrawing) reduces electron density on the phenyl ring, leading to stronger intermolecular halogen bonds compared to methyl-substituted analogs .
- Crystal Packing: Nitro-substituted derivatives (e.g., N-(3-nitrophenyl)acetamide) exhibit more complex hydrogen-bonding networks due to the NO₂ group’s polarity, whereas dichloro-substituted compounds prioritize N–H⋯Cl interactions .
Biological Activity
N-(3,5-dichlorophenyl)acetamide is a compound of significant interest due to its diverse biological activities. This article explores its pharmacological properties, mechanisms of action, and potential therapeutic applications based on various research findings.
Chemical Structure and Properties
This compound is characterized by a dichlorophenyl group attached to an acetamide moiety. The chemical formula is , indicating the presence of two chlorine atoms on the phenyl ring, which influences its biological reactivity and activity.
The biological activity of this compound is attributed to its interaction with various molecular targets:
- Enzyme Inhibition : It has been investigated as a potential protease inhibitor. The compound binds to the active site of specific enzymes, preventing substrate access and disrupting catalytic activity. This mechanism is particularly relevant in antiviral applications, where inhibiting viral proteases can hinder replication processes.
- Neurotransmitter Modulation : Studies suggest that this compound may modulate neurotransmitter systems, particularly those associated with gamma-aminobutyric acid (GABA). This action is believed to contribute to its anticonvulsant properties observed in animal models.
Antiviral Properties
This compound has shown promise as an antiviral agent. Its mechanism involves inhibiting viral proteases, which are crucial for viral replication. Research indicates that it may have potential activity against viruses such as SARS-CoV-2, although further studies are necessary to confirm its efficacy and safety in clinical settings .
Anticonvulsant Effects
In preclinical studies, derivatives of this compound have demonstrated significant anticonvulsant activity in various animal models. The compound's ability to interact with GABAergic systems suggests it may be effective in treating epilepsy.
Case Studies and Research Findings
Several studies have highlighted the biological activity of this compound:
Toxicity and Safety Profile
Toxicological assessments indicate that this compound exhibits low acute toxicity in animal models. In studies where rats were administered varying doses, no significant mortality was observed, and any adverse effects were transient and resolved within a few days . Long-term safety evaluations are still required to establish a comprehensive safety profile.
Future Directions
The ongoing research into this compound aims to further elucidate its pharmacodynamics and pharmacokinetics. Investigations into its interactions with different receptors and enzymes will be crucial for understanding its full therapeutic potential. Moreover, exploring its derivatives may yield compounds with enhanced efficacy and reduced side effects.
Q & A
Basic Research Questions
Q. What are the standard synthetic routes for N-(3,5-dichlorophenyl)acetamide, and how can purity be optimized?
Methodological Answer:
- Synthesis : React 3,5-dichloroaniline with acetyl chloride or acetic anhydride in the presence of a base (e.g., pyridine) under reflux. Alternative routes involve trichloroacetic acid and phosphoryl chloride (POCl₃) for derivatives like N-(3,5-dichlorophenyl)-2,2,2-trichloroacetamide .
- Purification : Recrystallize from ethanol or methanol. Slow evaporation yields single crystals suitable for X-ray diffraction (XRD) analysis .
- Purity Optimization : Monitor reactions via thin-layer chromatography (TLC) and characterize intermediates using nuclear magnetic resonance (NMR) spectroscopy .
Q. Which spectroscopic and crystallographic techniques are critical for characterizing this compound derivatives?
Methodological Answer:
- Spectroscopy :
- IR : Identify amide C=O stretching (~1650–1680 cm⁻¹) and N–H bending (~1550 cm⁻¹) .
- ¹H/¹³C NMR : Assign aromatic protons (δ 6.5–8.5 ppm) and acetamide methyl groups (δ 2.1–2.3 ppm) .
- Crystallography : Use single-crystal XRD (e.g., SHELX programs) to resolve hydrogen-bonding networks and confirm anti-parallel C=O/N–H conformations .
Q. What safety precautions are recommended when handling this compound?
Methodological Answer:
- Hazards : Causes respiratory, skin, and eye irritation due to electrophilic aromatic substituents .
- Mitigation : Use fume hoods, nitrile gloves, and safety goggles. Store at –20°C in airtight containers to prevent degradation .
Advanced Research Questions
Q. How do meta-substituents (e.g., Cl, CH₃) influence the solid-state geometry and reactivity of this compound derivatives?
Methodological Answer:
- Steric Effects : Bulkier substituents (e.g., CH₃) disrupt planar amide conformations, reducing hydrogen-bonding efficiency .
- Electronic Effects : Electron-withdrawing Cl groups enhance electrophilicity, increasing reactivity in nucleophilic aromatic substitutions .
- Validation : Compare XRD data of derivatives (e.g., 35DCPTCA vs. 35DMPTCA) to correlate substituent effects with lattice energies .
Q. What strategies are used to design this compound-based α-glucosidase inhibitors, and how is activity validated?
Methodological Answer:
- Design : Introduce heterocyclic moieties (e.g., thiazole, coumarin) to enhance target binding. Example: Compound 12 (IC₅₀ = 4.2 µM) .
- Validation :
- Enzyme Assays : Measure inhibition kinetics using p-nitrophenyl-α-D-glucopyranoside as substrate.
- Molecular Docking : Simulate interactions with α-glucosidase active sites (e.g., AutoDock Vina) .
Q. How do crystallographic challenges (e.g., twinning) in this compound derivatives affect structural refinement?
Methodological Answer:
- Twinning : Common in non-centrosymmetric space groups (e.g., Pna2₁). Use twin refinement in SHELXL with BASF parameter adjustments .
- Hydrogen Bonding : Resolve infinite chains via N–H···O interactions (2.8–3.0 Å) to stabilize crystal packing .
Q. What methodologies enable the development of immunoassays for this compound detection?
Methodological Answer:
- Hapten Synthesis : React N-(3,5-dichlorophenyl)-2-hydroxysuccinimide with succinic anhydride to generate a carboxylated derivative .
- Conjugation : Link hapten to carrier proteins (e.g., BSA) via carbodiimide crosslinkers. Validate using matrix-assisted laser desorption/ionization (MALDI-MS) .
Q. How does this compound compare to structurally analogous acetamides in agrochemical applications?
Methodological Answer:
- SAR Studies : Replace Cl with F or CH₃ to modulate lipophilicity and bioactivity. Example: N-(4-fluorophenyl) derivatives show reduced phytotoxicity .
- Field Trials : Evaluate fungicidal efficacy against Sclerotinia sclerotiorum at 50–100 ppm. Compare LC₅₀ values using probit analysis .
Properties
IUPAC Name |
N-(3,5-dichlorophenyl)acetamide | |
---|---|---|
Source | PubChem | |
URL | https://pubchem.ncbi.nlm.nih.gov | |
Description | Data deposited in or computed by PubChem | |
InChI |
InChI=1S/C8H7Cl2NO/c1-5(12)11-8-3-6(9)2-7(10)4-8/h2-4H,1H3,(H,11,12) | |
Source | PubChem | |
URL | https://pubchem.ncbi.nlm.nih.gov | |
Description | Data deposited in or computed by PubChem | |
InChI Key |
BJWFSDFXPISTBZ-UHFFFAOYSA-N | |
Source | PubChem | |
URL | https://pubchem.ncbi.nlm.nih.gov | |
Description | Data deposited in or computed by PubChem | |
Canonical SMILES |
CC(=O)NC1=CC(=CC(=C1)Cl)Cl | |
Source | PubChem | |
URL | https://pubchem.ncbi.nlm.nih.gov | |
Description | Data deposited in or computed by PubChem | |
Molecular Formula |
C8H7Cl2NO | |
Source | PubChem | |
URL | https://pubchem.ncbi.nlm.nih.gov | |
Description | Data deposited in or computed by PubChem | |
DSSTOX Substance ID |
DTXSID70185479 | |
Record name | Acetamide, N-(3,5-dichlorophenyl)- | |
Source | EPA DSSTox | |
URL | https://comptox.epa.gov/dashboard/DTXSID70185479 | |
Description | DSSTox provides a high quality public chemistry resource for supporting improved predictive toxicology. | |
Molecular Weight |
204.05 g/mol | |
Source | PubChem | |
URL | https://pubchem.ncbi.nlm.nih.gov | |
Description | Data deposited in or computed by PubChem | |
CAS No. |
31592-84-4 | |
Record name | Acetamide, N-(3,5-dichlorophenyl)- | |
Source | ChemIDplus | |
URL | https://pubchem.ncbi.nlm.nih.gov/substance/?source=chemidplus&sourceid=0031592844 | |
Description | ChemIDplus is a free, web search system that provides access to the structure and nomenclature authority files used for the identification of chemical substances cited in National Library of Medicine (NLM) databases, including the TOXNET system. | |
Record name | NSC83301 | |
Source | DTP/NCI | |
URL | https://dtp.cancer.gov/dtpstandard/servlet/dwindex?searchtype=NSC&outputformat=html&searchlist=83301 | |
Description | The NCI Development Therapeutics Program (DTP) provides services and resources to the academic and private-sector research communities worldwide to facilitate the discovery and development of new cancer therapeutic agents. | |
Explanation | Unless otherwise indicated, all text within NCI products is free of copyright and may be reused without our permission. Credit the National Cancer Institute as the source. | |
Record name | Acetamide, N-(3,5-dichlorophenyl)- | |
Source | EPA DSSTox | |
URL | https://comptox.epa.gov/dashboard/DTXSID70185479 | |
Description | DSSTox provides a high quality public chemistry resource for supporting improved predictive toxicology. | |
Synthesis routes and methods I
Procedure details
Synthesis routes and methods II
Procedure details
Retrosynthesis Analysis
AI-Powered Synthesis Planning: Our tool employs the Template_relevance Pistachio, Template_relevance Bkms_metabolic, Template_relevance Pistachio_ringbreaker, Template_relevance Reaxys, Template_relevance Reaxys_biocatalysis model, leveraging a vast database of chemical reactions to predict feasible synthetic routes.
One-Step Synthesis Focus: Specifically designed for one-step synthesis, it provides concise and direct routes for your target compounds, streamlining the synthesis process.
Accurate Predictions: Utilizing the extensive PISTACHIO, BKMS_METABOLIC, PISTACHIO_RINGBREAKER, REAXYS, REAXYS_BIOCATALYSIS database, our tool offers high-accuracy predictions, reflecting the latest in chemical research and data.
Strategy Settings
Precursor scoring | Relevance Heuristic |
---|---|
Min. plausibility | 0.01 |
Model | Template_relevance |
Template Set | Pistachio/Bkms_metabolic/Pistachio_ringbreaker/Reaxys/Reaxys_biocatalysis |
Top-N result to add to graph | 6 |
Feasible Synthetic Routes
Disclaimer and Information on In-Vitro Research Products
Please be aware that all articles and product information presented on BenchChem are intended solely for informational purposes. The products available for purchase on BenchChem are specifically designed for in-vitro studies, which are conducted outside of living organisms. In-vitro studies, derived from the Latin term "in glass," involve experiments performed in controlled laboratory settings using cells or tissues. It is important to note that these products are not categorized as medicines or drugs, and they have not received approval from the FDA for the prevention, treatment, or cure of any medical condition, ailment, or disease. We must emphasize that any form of bodily introduction of these products into humans or animals is strictly prohibited by law. It is essential to adhere to these guidelines to ensure compliance with legal and ethical standards in research and experimentation.