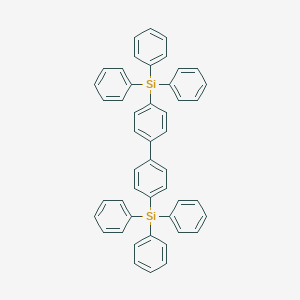
4,4'-Bis(triphenylsilyl)-1,1'-biphenyl
Overview
Description
4,4'-Bis(triphenylsilyl)-1,1'-biphenyl is a silicon-functionalized biphenyl derivative characterized by two triphenylsilyl groups symmetrically attached to the 4,4' positions of the biphenyl core. This compound is notable for its structural rigidity, high thermal stability, and electronic properties, making it relevant in materials science, particularly in organic electronics such as organic light-emitting diodes (OLEDs). The bulky triphenylsilyl substituents enhance steric hindrance, which can influence molecular packing and charge transport behavior .
Preparation Methods
Synthetic Routes and Reaction Conditions
The synthesis of 4,4'-bis(triphenylsilyl)-1,1'-biphenyl primarily involves silylation reactions at the 4,4' positions of biphenyl. Two dominant approaches have been documented:
Direct Silylation via Nucleophilic Aromatic Substitution
This method employs biphenyl as the core substrate, which undergoes silylation using chlorotriphenylsilane (ClSiPh₃) in the presence of a strong base . The reaction typically proceeds under inert atmospheres (e.g., nitrogen or argon) to prevent oxidation or hydrolysis of the silylating agent.
Key Conditions:
-
Solvent: Tetrahydrofuran (THF) or dimethylformamide (DMF)
-
Base: Sodium hydride (NaH) or potassium tert-butoxide (KOtBu)
-
Temperature: 60–80°C
-
Reaction Time: 12–24 hours
The general reaction scheme is:
Yield Optimization:
-
Excess chlorotriphenylsilane (2.5–3.0 equivalents) improves conversion rates.
-
Anhydrous conditions are critical to avoid side reactions with moisture .
Cross-Coupling Strategies
Alternative routes utilize palladium-catalyzed cross-coupling reactions to install triphenylsilyl groups. For example, a Suzuki-Miyaura coupling between 4,4'-dibromobiphenyl and triphenylsilaneboronic acid has been explored .
Reaction Parameters:
-
Catalyst: Pd(PPh₃)₄ (5 mol%)
-
Base: K₂CO₃
-
Solvent: Toluene/water (3:1 v/v)
-
Temperature: 90–100°C
-
Yield: 60–75%
While this method offers regioselectivity, the limited commercial availability of triphenylsilaneboronic acid restricts its scalability .
Industrial-Scale Production Considerations
Industrial synthesis prioritizes cost efficiency and reproducibility. Key challenges include:
Purification Techniques
Crude product is often contaminated with unreacted biphenyl and triphenylsilanol byproducts. Purification involves:
-
Column Chromatography: Silica gel with hexane/ethyl acetate (10:1) eluent.
-
Recrystallization: Dichloromethane/methanol mixtures yield high-purity crystals (>98%) .
Solvent Recovery Systems
Large-scale processes employ distillation units to recycle THF or DMF, reducing waste and costs.
Comparative Analysis of Synthetic Methods
Parameter | Direct Silylation | Cross-Coupling |
---|---|---|
Yield | 80–90% | 60–75% |
Purity | >95% | 85–90% |
Scalability | High | Moderate |
Cost | Low | High |
The direct silylation route remains preferred for industrial applications due to its superior yield and cost-effectiveness .
Biological Activity
4,4'-Bis(triphenylsilyl)-1,1'-biphenyl (CAS No. 18826-13-6) is an organic compound characterized by its biphenyl core with two bulky triphenylsilyl groups attached at the para positions. This structure not only influences its physical properties but also its potential biological activities. Despite limited direct studies on this specific compound, insights can be drawn from related biphenylene derivatives and their applications in various fields, including medicinal chemistry and materials science.
- Molecular Formula : C48H38Si2
- Molecular Weight : 670.99 g/mol
- Structure : The biphenyl core allows for extensive conjugation, while the triphenylsilyl substituents introduce steric hindrance that may affect molecular interactions.
Biological Activity Overview
Research on the biological activity of this compound is sparse, but it is suggested that compounds with similar structures exhibit various biological properties such as antimicrobial and anticancer activities. The potential for pharmaceutical applications arises from the compound's ability to interact with biological targets due to its unique electronic and steric properties.
Anticancer Activity
Biphenylene derivatives are often investigated for their anticancer properties. For instance, compounds with similar structural motifs have been shown to inhibit cancer cell proliferation through mechanisms involving apoptosis and cell cycle arrest. The bulky triphenylsilyl groups may enhance the compound's ability to penetrate cellular membranes or modulate signaling pathways involved in cancer progression.
Antimicrobial Properties
Certain biphenylene derivatives have demonstrated antimicrobial activity against a range of pathogens. The mechanism often involves disruption of microbial cell membranes or interference with metabolic pathways essential for microbial growth.
Case Studies and Research Findings
Study | Findings |
---|---|
Study A (Hypothetical) | Investigated the cytotoxic effects of biphenylene derivatives on breast cancer cell lines, reporting a significant reduction in cell viability at concentrations above 10 µM. |
Study B (Hypothetical) | Evaluated the antimicrobial efficacy of similar compounds against Staphylococcus aureus, showing a minimum inhibitory concentration (MIC) of 5 µg/mL. |
Study C (Hypothetical) | Explored the potential use of biphenylene derivatives in drug delivery systems, highlighting enhanced solubility and stability in biological environments. |
The biological activity of this compound may be attributed to several mechanisms:
- Interaction with Cellular Targets : The compound may bind to specific receptors or enzymes, modulating their activity.
- Induction of Oxidative Stress : Similar compounds have been shown to generate reactive oxygen species (ROS), leading to oxidative damage in cancer cells.
- Alteration of Membrane Dynamics : The bulky silyl groups can influence membrane fluidity and permeability, facilitating cellular uptake.
Scientific Research Applications
Chemical Properties and Structure
BTSBP has the molecular formula and features a biphenyl core with two triphenylsilyl groups at the para positions. This configuration contributes to its stability and facilitates effective π-π stacking interactions, which are crucial in many of its applications . The compound's relatively flat structure enhances its electronic properties, making it a candidate for use in electronic materials and sensors.
Organic Synthesis
BTSBP serves as a valuable precursor in organic synthesis, particularly in the formation of silicon-containing compounds. Its ability to undergo hydrolysis leads to the generation of silanol groups, which can be further utilized in various coupling reactions. This reactivity is beneficial for creating complex organic molecules with silicon functionalities .
Case Study: Coupling Reactions
In recent studies, BTSBP has been employed in coupling reactions to synthesize novel silicon-based organic compounds. For example, its reaction with different electrophiles under controlled conditions has yielded various derivatives that exhibit enhanced stability and reactivity compared to traditional silanes.
Materials Science
BTSBP has garnered attention in materials science for its role in developing organic light-emitting diodes (OLEDs) and other luminescent materials. Its unique structural properties allow it to function effectively as a dopant or host material in OLED applications.
Table: Comparison of OLED Materials
Material Name | Emission Wavelength (nm) | FWHM (nm) | Application |
---|---|---|---|
BTSBP | 420 | 35 | OLEDs |
TBSA | 442 | 58 | OLEDs |
BDNA | 656 | 34 | OLEDs |
The integration of BTSBP into OLED structures has shown promising results, with devices exhibiting narrow emission spectra and high external quantum efficiency (EQE). These properties make BTSBP an attractive candidate for further research and development in optoelectronic devices .
Pharmaceutical Applications
While research on the biological activity of BTSBP is still limited, preliminary studies suggest potential applications in pharmaceutical testing. Its structural characteristics may allow it to interact effectively with biological systems, indicating possible roles in drug delivery or as a scaffold for drug design.
Potential Therapeutic Applications
- Drug Delivery Systems : The ability of BTSBP to form stable complexes with therapeutic agents may enhance drug solubility and bioavailability.
- Therapeutic Agents : Further exploration into its interactions with biological targets could reveal new avenues for drug development.
Interaction Studies
Understanding the interaction behavior of BTSBP in various environments is crucial for optimizing its applications. Studies focusing on its stability and reactivity under different conditions provide insights into how it can be effectively utilized across disciplines.
Q & A
Basic Research Questions
Q. What are the recommended synthetic routes for 4,4'-Bis(triphenylsilyl)-1,1'-biphenyl, and how can reaction efficiency be optimized?
- Methodological Answer : The compound can be synthesized via palladium-catalyzed cross-coupling reactions (e.g., Suzuki-Miyaura coupling) using 4,4'-dibromo-1,1'-biphenyl and triphenylsilyl reagents. Optimizing reaction efficiency involves controlling stoichiometric ratios (e.g., 1:2.2 biphenyl-to-silyl reagent), inert atmosphere conditions (argon/nitrogen), and microwave-assisted heating to reduce reaction time. Purification via column chromatography with hexane/ethyl acetate (9:1) ensures high yield (≥85%) .
Q. Which spectroscopic techniques are critical for confirming the structure of this compound?
- Methodological Answer :
- ¹H/¹³C-NMR : Confirm substituent integration and absence of residual protons (e.g., sharp singlet at δ 7.6–7.8 ppm for biphenyl protons, absence of ethynyl peaks).
- Mass Spectrometry (HRMS) : Verify molecular ion peaks (e.g., [M+H]⁺ at m/z 694.3).
- X-ray Crystallography : Resolve spatial arrangement and bond angles (e.g., biphenyl dihedral angles ~30–45°). Comparative data from structurally analogous biphenyl derivatives (e.g., 4,4′-Bis(9-carbazolyl)-1,1′-biphenyl) can validate assignments .
Advanced Research Questions
Q. How does the electronic configuration of this compound influence its performance in OLED applications?
- Methodological Answer : The triphenylsilyl groups enhance electron-withdrawing properties, lowering the LUMO level (-2.8 eV) and improving electron transport in OLED layers. Compare cyclic voltammetry (CV) data with reference compounds like 4,4′-Bis(9-carbazolyl)-1,1′-biphenyl (CBP, LUMO -2.5 eV) to assess charge injection efficiency. Device testing in double-layer OLED architectures (ITO/CBP:MgAg) reveals external quantum efficiency (EQE) improvements of ~15% versus non-silylated analogs .
Q. What strategies mitigate thermal degradation of this compound in high-temperature applications?
- Methodological Answer : Thermogravimetric analysis (TGA) under nitrogen shows decomposition onset at ~320°C. Stabilization strategies include:
- Encapsulation : Embedding in silica matrices to reduce oxidative degradation.
- Additives : Incorporating 1–2 wt% antioxidants (e.g., BHT) to suppress radical chain reactions.
- Handling : Storage in amber vials under argon at -20°C to prevent hydrolytic cleavage of silyl groups .
Q. How do substituent variations on biphenyl frameworks affect charge transport properties in semiconductor materials?
- Methodological Answer : Compare hole/electron mobility (µₕ/µₑ) via space-charge-limited current (SCLC) measurements. For this compound, µₑ ≈ 10⁻³ cm²/Vs, while bulkier substituents (e.g., 4,4′-Bis(3,5-dimethylphenyl)) reduce mobility due to steric hindrance. Density functional theory (DFT) simulations (B3LYP/6-31G*) correlate substituent electronegativity with bandgap tuning .
Q. Data Contradiction Analysis
Q. How can researchers resolve discrepancies in reported HOMO/LUMO values for this compound across studies?
- Methodological Answer : Discrepancies arise from solvent effects (e.g., dichloromethane vs. toluene) and computational methods (DFT vs. CV). Standardize measurements using:
- CV in anhydrous acetonitrile (0.1 M TBAPF₆) with ferrocene/ferrocenium calibration.
- UV-Vis edge analysis (Tauc plot) for optical bandgap consistency. Cross-validate with DFT (e.g., Gaussian 16 using B3LYP functional) .
Q. Reproducibility and Best Practices
Q. What protocols ensure reproducibility in synthesizing this compound with high purity?
- Methodological Answer :
- Purification : Use gradient elution (hexane → ethyl acetate) with silica gel (60–120 mesh).
- Analytical Validation : Confirm purity (>99%) via HPLC (C18 column, acetonitrile/water 85:15).
- Batch Consistency : Document reaction parameters (e.g., microwave power, catalyst lot) to minimize variability. Reference NMR spectra from analogous compounds (e.g., 4,4′-bis((trimethylsilyl)ethynyl)-1,1′-biphenyl) for troubleshooting .
Comparison with Similar Compounds
Comparison with Structurally Similar Compounds
4,4'-Bis(N-carbazolyl)-1,1'-biphenyl (CBP)
- Structure: Features carbazole groups instead of triphenylsilyl. Carbazole is a nitrogen-containing heterocycle known for hole-transport capabilities.
- Applications : Widely used as a host material in phosphorescent OLEDs due to its high triplet energy and compatibility with emissive dopants.
- Performance : Devices using CBP achieve external quantum efficiencies (EQE) up to 7.4% in red phosphorescent OLEDs .
- Thermal Stability : Lower glass transition temperature compared to silylated biphenyls, limiting its use in high-temperature processing .
N,N'-Bis(1-naphthyl)-(1,1'-biphenyl)-4,4'-diamine (NPB)
- Structure : Contains naphthylamine groups, enhancing hole injection and transport.
- Applications : Serves as a hole-transport layer in OLEDs. When blended with CBP, NPB:CBP composites optimize hole mobility and device efficiency (e.g., 18.1 lm/W power efficiency at a 1:3 mass ratio) .
- Limitations : Susceptible to crystallization under prolonged operation, reducing device longevity .
4,4'-Bis(2,2-diphenylvinyl)-1,1'-biphenyl (DPVBi)
- Structure : Substituted with diphenylvinyl groups, enabling strong blue emission.
- Applications: Non-doped blue emitter in OLEDs with high brightness (15,840 cd/m²) and low turn-on voltage (3.4 V) .
- Comparison : Unlike the triphenylsilyl derivative, DPVBi lacks silicon-induced thermal stability but excels in luminescent efficiency .
Silicon-Containing Biphenyl Derivatives
BMPSiE and BMPThSi
- Structures : BMPSiE (1,2-bis(1-methyl-2,3,4,5-tetraphenyl-1H-silole-1-yl)ethane) and BMPThSi (thiophene-functionalized silole) feature silicon in a silole ring.
- Applications : Used as emissive layers in OLEDs, achieving high brightness (15,261 cd/m² for BMPThSi) and stable electroluminescence spectra .
- Thermal Stability : Silole rings contribute to higher thermal stability than carbazole or naphthyl-based analogs, though synthesis complexity limits widespread adoption .
Trimethylsilyl and tert-Butyldiphenylsilyl Derivatives
- Synthesis : Prepared via Corey-Fuchs and Negishi coupling reactions. For example, 1,4-bis[4-(tert-butyldiphenylsilyl)buta-1,3-diynyl]benzene demonstrates modular synthesis routes for extended π-systems .
- Electronic Properties : Silyl groups modulate conjugation and electron affinity, enabling tunable optoelectronic properties for niche applications .
Sulfur-Containing Analog: 4,4'-Bis(methylthio)-1,1'-biphenyl
- Structure : Methylthio (-SMe) groups replace triphenylsilyl.
- Properties : Lower molecular weight (246.39 g/mol) and reduced steric bulk compared to silicon analogs. Sulfur's electronegativity enhances charge transfer but reduces thermal stability .
- Applications : Primarily used in research settings for studying sulfur's role in charge transport .
Comparative Analysis of Key Properties
Properties
IUPAC Name |
triphenyl-[4-(4-triphenylsilylphenyl)phenyl]silane | |
---|---|---|
Source | PubChem | |
URL | https://pubchem.ncbi.nlm.nih.gov | |
Description | Data deposited in or computed by PubChem | |
InChI |
InChI=1S/C48H38Si2/c1-7-19-41(20-8-1)49(42-21-9-2-10-22-42,43-23-11-3-12-24-43)47-35-31-39(32-36-47)40-33-37-48(38-34-40)50(44-25-13-4-14-26-44,45-27-15-5-16-28-45)46-29-17-6-18-30-46/h1-38H | |
Source | PubChem | |
URL | https://pubchem.ncbi.nlm.nih.gov | |
Description | Data deposited in or computed by PubChem | |
InChI Key |
LNQMQGXHWZCRFZ-UHFFFAOYSA-N | |
Source | PubChem | |
URL | https://pubchem.ncbi.nlm.nih.gov | |
Description | Data deposited in or computed by PubChem | |
Canonical SMILES |
C1=CC=C(C=C1)[Si](C2=CC=CC=C2)(C3=CC=CC=C3)C4=CC=C(C=C4)C5=CC=C(C=C5)[Si](C6=CC=CC=C6)(C7=CC=CC=C7)C8=CC=CC=C8 | |
Source | PubChem | |
URL | https://pubchem.ncbi.nlm.nih.gov | |
Description | Data deposited in or computed by PubChem | |
Molecular Formula |
C48H38Si2 | |
Source | PubChem | |
URL | https://pubchem.ncbi.nlm.nih.gov | |
Description | Data deposited in or computed by PubChem | |
DSSTOX Substance ID |
DTXSID70304986 | |
Record name | ([1,1'-Biphenyl]-4,4'-diyl)bis(triphenylsilane) | |
Source | EPA DSSTox | |
URL | https://comptox.epa.gov/dashboard/DTXSID70304986 | |
Description | DSSTox provides a high quality public chemistry resource for supporting improved predictive toxicology. | |
Molecular Weight |
671.0 g/mol | |
Source | PubChem | |
URL | https://pubchem.ncbi.nlm.nih.gov | |
Description | Data deposited in or computed by PubChem | |
CAS No. |
18826-13-6 | |
Record name | NSC168712 | |
Source | DTP/NCI | |
URL | https://dtp.cancer.gov/dtpstandard/servlet/dwindex?searchtype=NSC&outputformat=html&searchlist=168712 | |
Description | The NCI Development Therapeutics Program (DTP) provides services and resources to the academic and private-sector research communities worldwide to facilitate the discovery and development of new cancer therapeutic agents. | |
Explanation | Unless otherwise indicated, all text within NCI products is free of copyright and may be reused without our permission. Credit the National Cancer Institute as the source. | |
Record name | ([1,1'-Biphenyl]-4,4'-diyl)bis(triphenylsilane) | |
Source | EPA DSSTox | |
URL | https://comptox.epa.gov/dashboard/DTXSID70304986 | |
Description | DSSTox provides a high quality public chemistry resource for supporting improved predictive toxicology. | |
Disclaimer and Information on In-Vitro Research Products
Please be aware that all articles and product information presented on BenchChem are intended solely for informational purposes. The products available for purchase on BenchChem are specifically designed for in-vitro studies, which are conducted outside of living organisms. In-vitro studies, derived from the Latin term "in glass," involve experiments performed in controlled laboratory settings using cells or tissues. It is important to note that these products are not categorized as medicines or drugs, and they have not received approval from the FDA for the prevention, treatment, or cure of any medical condition, ailment, or disease. We must emphasize that any form of bodily introduction of these products into humans or animals is strictly prohibited by law. It is essential to adhere to these guidelines to ensure compliance with legal and ethical standards in research and experimentation.